11
Guided Inquiry in the Science Classroom
James Minstrell and Pamela Kraus
The story of the development of this piece of curriculum and instruction starts in the classroom of the first author more than 25 years ago. I had supposedly taught my classes about universal gravitation and the related inverse square force law. The students had performed reasonably well on questions of the sort that asked, “What would happen to the force if we increased the distance from the planet?” They supposedly understood something about gravitational forces, resistive forces of air resistance and friction, and the idea of force in general. Then came a rude awakening.
I don’t remember why, but we happened to be talking about a cart being pulled across a table by a string attached to a weight over a pulley. The students were becoming confused by the complexity of the situation. So, in an attempt to simplify the context, I suggested, “Suppose there is no friction to worry about, no rubbing, and no friction.” Still the students were confused and suggested, “Then there would be so much wind resistance.” I waved that notion away as well: “Suppose there were no friction at all and no air resistance in this situation. Suppose there were no air in the room. Now what would be the forces acting on this cart as it was moving across the table?”
I was not prepared for what I heard. Several voices around the room were saying, in effect, “Then things would just drift off the table. The weight and string and cart would all just float away.” I was tempted to say, “No, don’t think like that.” I suppressed that urge and instead asked in a nonevaluative tone, “Okay, so you say things would just float away. How do you know that?” They suggested, “You know, like in space. There is no air, and things just drift around. They aren’t held down, because there is no air
to hold them down.” The students said they knew this because they had heard from the media that in space things are weightless. Indeed, they had seen pictures of astronauts just “floating” around. They had also been told that there is no air in space, and they put the two (no air and weightless) together. But they had no first-hand experiences to relate to what they knew from these external “authorities.”
If we really want to know what students are thinking, we need to ask them and then be quiet and listen respectfully to what they say. If we are genuinely interested and do not evaluate, we can learn from our students. |
What good is having my students know the quantitative relation or equation for gravitational force if they lack a qualitative understanding of force and the concepts related to the nature of gravity and its effects? They should be able to separate the effects of gravity from the effects of the surrounding air. Later, they should be able to explain the phenomena of falling bodies, which requires that they separate the effects of gravity from those of air. While many physical science books focus on the constancy of gravitational acceleration, most students know that all things do not fall with the same acceleration. They know that a rock reaches the floor before a flat sheet of paper, for example. Not addressing the more common situation of objects falling differently denies the students’ common experiences and is part of the reason “school science” may not seem relevant to them. So, we need to separate the effects of air from those of gravity.
Learning is an active process. We need to acknowledge students’ attempts to make sense of their experiences and help them confront inconsistencies in their sense making. |
Even more fundamental, I want my students to understand and be able to apply the concept of force as an interaction between objects in real-life situations. They should have first-hand experiences that will lead to the reasonable conclusion that force can be exerted by anything touching an object, and also that forces can exist as “actions at a distance” (i.e., without touching the object, forces might be exerted through the mechanisms of gravity, electrostatic force, and magnetic force).
I also want my students to understand the nature of scientific practice. They should be able to interpret or explain common phenomena and design simple experiments to test their ideas. In short, I want them to have the skills necessary to inquire about the world around them, to ask and answer their own questions, and to know what questions they need to ask themselves in the process of thinking about a problematic situation.
Teachers’ questions can model the sorts of questions students might ask themselves when conducting personal inquiry. |
Research and best practice suggest that, if we are really clever and careful, students will come more naturally to the conceptual ideas and processes we want them to learn. Being clever means incorporating what we have come to understand about how students learn. This chapter describes a series of activities from which the experience of teachers and researchers demonstrates students do learn about the meaning of force and about the nature and processes of science. It also explains how the specific activities and teaching strategies delineated here relate to what we know from research on how people learn, as reflected in the three guiding principles set forth in Chapter 1 with regard to students’ prior knowledge, the need to develop deep understanding, and the development of metacognitive awareness. We attempt to give the reader a sense of what it means to implement curriculum that supports these principles. It is our hope that researchers will see that we have built upon their work in designing these activities and creating the learning environment. We want teachers to get a sense of what it means to teach in such an environment. We also want readers to get an idea of what it is like to be a learner.
The following unit could come before one on forces to explain motion (i.e., Newton’s Laws). By the end of this unit, students should have arrived at a qualitative understanding of force as applied in contexts involving buoyancy, gravitation, magnetics, and electrostatics. The activities involved are designed to motivate and develop a sense of the interrelationships between ideas and events. The expected outcome includes qualitative understanding of ideas, not necessarily formulas.
THE UNIT: THE NATURE OF GRAVITY AND ITS EFFECTS
Part A: What Gravity Is Not
Getting the Unit Started: Finding Out About Students’ Initial Ideas
Teachers need to unconditionally respect students’ capacities for learning complex ideas, and students need to learn to respect the teacher as an instructional leader. Teachers will need to earn that respect through their actions as a respectful guide to learning. |
For students to understand the following lessons, we need to establish some prerequisite knowledge and dispositions during earlier lessons. Students will need to understand that measurements of a single quantity may vary depending on three factors: the object being measured, the instrument being used, and the person using the instrument. The teacher needs to have enough experience with the class so that the students are confident that the class will achieve resolution over time. Thus, this unit comes about a month or so into the school year. Students need to persevere in learning and trusting that the teacher will help guide them to the big ideas. This should probably not be the students’ first experience with guided inquiry. While the set of experiences in Part A below takes a week or more to resolve, prior initial experiences with guided inquiry may take a class period or two, depending on the students’ tolerance for ambiguity.
Identifying Preconceptions: What Would Happen If …?
Teachers need to know students’ initial and developing conceptions. Students need to have their initial ideas brought to a conscious level. |
One way to find out about students’ preconceptions for a particular unit is to ask them to give, in writing, their best answers to one or more questions related to the unit. At the beginning of this unit on the nature of gravity and its effects, the teacher poses the following situation and questions associated with Figure 11-1.
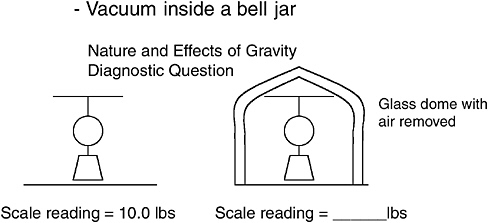
FIGURE 11-1 A diagnostic question to use at the beginning of this unit.
Nature and Effects of Gravity, Diagnostic Question 1: Predict the scale reading under the glass dome with air removed.
In the diagram with question 1, we have a large frame and a big spring scale, similar to what you might see at the local market. Suppose we put something on the scale and the scale reading is 10.0 lb. Now suppose we put a large glass dome over the scale, frame and all, and seal all the way around the base of the dome. Then, we take a large vacuum pump and evacuate all the air out from under the dome. We allow all the air to escape through the pump, so there is no air left under the glass dome.
What would happen to the scale reading with no air under the dome? You may not be able to give a really precise answer, but say what you think would happen to the scale reading, whether it would increase, decrease, or stay exactly the same and if you think there will be a change, about how much? And briefly explain how you decided.
I will not grade you on whether your answer is correct. I just want to know your ideas about this situation at this time. We are just at the beginning of the unit. What I care most about is that you give a good honest best attempt to answer at this point in time. I know that some of you may be tempted to say “I don’t know,” but just give your best answer at this time. I’m pretty sure most all of you can come up with an answer and, most importantly, some rationale to support that answer. Just give me your best answer and reasoning at this point in time. We will be working to investigate this question over the next few days.
When asked, more than half of students cite answers that suggest they believe air only presses down. Half of those suggest that the scale reading would go to zero in the vacuous environment. About a third of introductory students believe that the surrounding air has absolutely no effect on the scale reading regardless of the precision of the scale. Most of the rest believe that air only pushes up on the object and that it does so with a strong force. Typically, only about one student in a class will suggest that the air pushes up and down but with slightly greater force in the upward direction, the result being a very slight increase in the scale reading for the vacuous environment—a “best answer” at this time.
This question may be more about understanding buoyancy than understanding gravity. However, part of understanding the effects of gravity is learning what effects are not due to gravity.
Students need opportunities to explore the relationships among ideas. |
Gravitational force is an interaction between any two objects that have mass. In this case, the gravitational force is an interaction between the object on the scale and the earth as the other object. Many students believe gravity is an interaction between the object and the surrounding air. Thus, this has become a first preconception to address in instruction. If teachers fail to address this idea, we know from experience that students will likely not change their basic conceptual understanding, and teachers will obtain the poor results described earlier.
In contrast with the above question, we have seen curricula that attempt to identify students’ preconceptions simply by asking students to write down what they know about X. In our experience, this question is so generic that students tend not to pay much attention to it and simply “do the assignment” by writing anything. Instead, preinstruction questions should be more specific to a context, but open up the issues of the discipline as related to that context. These sorts of questions are not easy to create and typically evolve out of several iterations of teaching a unit and finding out through discussions what situations elicit the more interesting responses with respect to the content at hand.
A Benchmark Lesson1: Weighing in a Vacuum
In discussion following the posing of this question, I encourage students to share their answers and rationales. Because I am interested in getting students’ thinking out in the open, I ask that other students not comment or offer counter arguments at this point, but just listen to the speaker’s argument. I, in turn, listen carefully to the sorts of thinking exhibited by the students. I know this will faciliate my helping the class move forward later.
With encouragement and support on my part, some students volunteer to share their answers. Some suggest the scale will go to zero “with no air to hold the object down.” Others suggest, “The scale reading will not go to zero but will go down some because gravity is still down and the weight of the air pushes down too, but since air doesn’t weigh very much, the downward air won’t be down much and the scale reading won’t go down much.” Some students suggest that the scale reading will increase (slightly or substantially) “because there is no air to hold the object up. It’s about buoyancy. The air is like water. Water pushes up and so does air. No air, there is no buoyancy.” Still others suggest that the scale reading should stay the same “because air doesn’t do anything. The weight is by gravity not by air pressure.” And others agree that the scale reading will not change, “but air is pushing on the object. It pushes up and down equally on the object, so there shouldn’t be any change.” By now several students have usually chimed in to say that one or another of the ideas made sense to them. The ideas are now “owned” by several class members, so we can discuss and even criti-
cize the ideas without criticizing a particular person. It is important to be supportive of free expression of ideas while at the same time being critical of ideas.
Students are more likely to share their thinking in a climate where others express genuine interest in what they have to say. Waiting until one student has completely expressed his or her idea fosters deeper thinking on that speaker’s part. Asking speakers critical questions to clarify what they are saying or to help them give more complete answers and explanations fosters their own engagement and learning. |
With most of their initial thinking having been expressed, I encourage students to share potentially contradictory arguments in light of the candidate explanations. Students might suggest, “When they vacuum pack peanuts, they take the air away and the weight doesn’t go to zero”; or “The weight of the column of air above an object pushes down on the object”; or “Air acts like water and when you lift a rock in water it seems lighter than lifting it out of water, so air would help hold the object up”; or “But, I read where being on the bottom of the ocean is like having an elephant standing on your head, so air must push down if it acts like water”; or “Air is just around things. It doesn’t push on things at all, unless there is a wind.” Some students begin to say they are getting more confused, for many of these observations and arguments sound good and reasonable.
Once arguments pro and con for most of the ideas have been expressed, it is time to begin resolving issues. Thus far, we have been freely expressing ideas, but I want students to know that science is not based simply on opinion. We can achieve some resolution by appealing to nature; indeed, our inferences should be consistent with our observations of nature. I ask, “Sounds like a lot of good arguments and experiences suggested here, so how can we get an answer? Should we just vote on which should be the right answer and explanation?” Typically, several of the students suggest, “No, we can try it and see what happens. Do you have one of those vacuum things? Can we do the experiment?”
I just happen to have a bell jar and vacuum pump set up in the back room. First, I briefly demonstrate what happens when a slightly inflated balloon (about 2 inches in diameter) is placed under the bell jar and the pump is turned on: the balloon gets larger. I ask the students to explain this result. The students (high school age at least) usually are able to articulate that I did not add air to the balloon, but the air outside the balloon (within the bell jar) was evacuated, so the air in the balloon was freer to expand the balloon.
Attention is extremely important to learning. |
We hang a weight on the spring scale, put it under the jar, and seal the edges, and I ask students to “place their bets.” This keeps students motivated and engaged. “How many think the scale reading will increase?” Hands go up. “Decrease?” Many hands go up. “Decrease to zero?” A few hands go up. “Stay exactly the same?” Several hands go up. I start the pump.
It is important to give students opportunities to apply (without being told, if possible) ideas learned earlier. |
The result surprises many students. The scale reading does not appear to change at all. Some students give a high five. I ask, “What can we conclude about the effects of air on the scale reading?” Some students suggest, “Air doesn’t do anything.” Sometimes to get past this response, I need to prime the discussion of implications of the results by asking, “Do we know air has absolutely no effect?” A few students are quick to say, “We don’t know that it has absolutely no effect. We just know it doesn’t have enough effect to make a difference.” I ask, “Why do you say that?” They respond, “Remember about measurements, there is always some plus or minus to it. It could be a tiny bit more than it was. It could be a tiny bit less, or it might be exactly the same. We can’t tell for sure. Maybe if we had a really, really accurate scale we could tell.”
I also want the students to see that conclusions are different from results, so I often guide them carefully to discuss each. “First, what were the actual results of the experiment? What did happen? What did we observe?” Students agree that there was no observable change in the scale reading. “Those were the results. We observed no apparent change in the scale reading.”
Students should be provided opportunities to differentiate between summarizing observable results and the conclusions generalized from those results. |
Because I want students to understand the role of experimentation in science, I press them for a conclusion: “So, what do we know from this experiment? Did we learn anything?” Although a few students suggest, “We didn’t learn anything,” others are quick to point out, “There can’t be any big changes. We know that the air doesn’t have a big effect.” At this point, it appears students have had sufficient experience talking about the ideas, so I may try to clarify the distinction between results and conclusions: “Conclusions are different from results. Conclusions are about the meaning of the
results, about making sense of what we observed. So, what can we conclude? What do these results tell us about the effects of the air?” With some additional discussion among the students, and possibly some additional clarification of the difference between results and conclusions, most students are ready to believe the following summary of their comments: “If the air has any effect on the scale reading, it is not very large. And apparently gravity is not caused by air pressure pressing things down.”
Activity A1
Activity A1 is a simple worksheet asking students to review their answers to questions about their initial ideas, other ideas that have come out in discussion, and the results and conclusions from the preceding benchmark lesson. Typically, I hand this summary sheet out as homework and collect it at the beginning of the next class. By reviewing what students have written, I can identify related issues that need to be discussed further with certain students. Alternatively, I may ask students to check and discuss their answers with each other in groups and to add a page of corrections to their own answers before handing in their original responses. One purpose of this activity is to encourage students to monitor their own learning.
Students need opportunities to learn to monitor their own learning. |
Progressing from the preinstruction question through the benchmark discussion takes about one class period. In showing that gravity is not caused by air pressure, we have generated questions about the effects of the surrounding air. Students now want to know the answer to the original question. I used to end the investigations of the surrounding air at this point and move on to investigating factors affecting gravity, but I discovered that students slipped back to believing that air pressed only down or only up. Therefore, we redesigned the curriculum activities to include more time for investigation into the effects of surrounding fluids. Doing so also allows us to incorporate some critical introductory experiences with qualitative ideas about forces on objects. This experience helps lay the groundwork for the later unit on forces, when we will revisit these ideas and experiences. To deepen students’ understanding of the effects of surrounding fluids then, we now engage in several elaboration activities wherein students have opportunities to test various hypotheses that came up in the benchmark discussion.
Revisiting ideas in new contexts helps organize them in a rich conceptual framework and facilitates application across contexts. |
Opportunities for Students to Suggest and Test Related Hypotheses
In the benchmark lesson, several ideas were raised that need further testing. Some students suggested air only pushed up, others that air only pushed down, still others that air pushed equally or did not push at all. Some suggested that air was like water; others contested that idea. Each of the following activities is intended to give students opportunities to test these ideas in several contexts, recognizable from their everyday world. That is, each activity could easily be repeated at home; in fact, some students may have already done them. One goal of my class is for students to leave seeing the world differently. Groups of three or four students each are assigned to “major” in one of the elaboration activities and then to get around also to investigating each of the other activities more briefly. In every case, they are asked to keep the original bell jar experiment in mind: “How does this activity help us understand the bell jar situation?” With respect to the activity in which they are majoring, they will also be expected to present their results and conclusions to the class.
Elaboration Activity A2: The Inverted Glass of Water. This activity was derived from a trick sometimes done at parties. A glass of water with a plastic card over the opening is inverted. If this is done carefully, the water stays in the glass. Students are asked to do the activity and see what they can learn about the directions in which air and water can push. They are also given the opportunity to explore the system and see what else they can learn.
Allowing students freedom to explore may give teachers opportunities to learn. Teachers need to allow themselves to learn. |
My purpose here is to help students see that air can apparently push upward (on the card) sufficiently to support the card and the water. That is usually one conclusion reached by some students. Early in my use of the activity, however, I was surprised by a student who emptied the water and placed the card over the open end of the inverted glass and concluded, “It’s the stickiness of water that holds the card to the glass.” For a moment I was taken aback, but fortunately other students came to my rescue. They said, “At first we thought it might be because the card just stuck to the wet glass, but then we loaded the card with pennies to see how many pennies the card would hold to the empty glass. We found it would only hold about three pennies before the card would drop off. The water we had in the glass weighs a lot more than three pennies. Stickiness might help, but it is not the main reason the card stays on. The main reason must be the air below the card.”
This was such a nice example of suggesting and testing alternative explanations that I now bring up the possibility of the stickiness being all that is needed if this idea does not come up in the group presentation. More recently, other students have tested the stickiness hypothesis by using a rigid plastic glass with a tiny (~1 mm) hole in the bottom. When they fill the glass, put on the card, and invert the glass, they put their finger over the hole. When they move their finger off the hole, the water and card fall. They conclude that the air rushing in the hole pushes down on the water and that the air pushing from under the card is not providing sufficient support. I now make sure I have plastic cups available in case I need to “seed” the discussion.
After making these observations, students are ready to draw the tentative conclusion that the upward push by the air on the card must be what is supporting most of the weight of the water on the card. They note the water must push down on the card, and since the stickiness of the water is not enough to hold the card, there must be a big push up by the air. This conclusion is reached more easily by more mature students than by middle-level students. The latter need help making sense of this argument. Most are willing to say tentatively that it makes sense that the air pushes up and are more convinced after they see the various directions in which air pushes in the other activities.
Elaboration Activity A3: Inverted Cylinder in a Cylinder of Water. This activity was derived from some students describing observations they had made while hand-washing dishes. They had observed what happened when an inverted glass was submerged in a dishpan of water. In activity A3, a narrow cylinder (e.g., 100 ml graduated cylinder) is inverted and floated in a larger cylinder (e.g., 500 ml graduated cylinder) of water. Again, students are asked to see what they can learn about the directions that air and water can push.
I want students to see that air and water can push up and down, and that the deeper one goes in a fluid, the greater is the push in any direction. While doing this activity, students observe that the farther down one pushes the floating cylinder, the more difficult it is to push. Thus, they conclude that the water is pushing upward on the air in the small cylinder, and the push is greater the deeper one goes. Typically, some students cite as additional evidence the observation that the water level in the small cylinder rises within that cylinder the farther down one pushes the small cylinder, thus compressing the air. I commend these students for their careful observation and suggest that other students observe what happens to the level of the water in the inner cylinder. The more the air is compressed, the harder the water must be pushing upward on the air to compress it, and the more the compressed air must be pushing upward on the inside of the small cylinder.
The students appear to have reached the conclusions I hoped for. Although I primed them with relevant questions, they made the observations and reached the conclusions.
Elaboration Activity A4: Leaky Bottle. This activity, like the others, came from experiences students had suggested helped them with their thinking about fluids. A 2-liter plastic soda bottle with three holes in it at three different heights is filled to the top with water and allowed to leak into a basin. Again, students are asked to see what they can learn about the directions in which air and water can push.
By listening to students’ arguments, the teacher can learn what related experiences make sense to them. |
Here I want students to learn that air and water can push sideways as well as up and down and again, that the push of air and water is greater the deeper one goes. “Suppose there is a tiny drop of water at this opening. In what direction would the air push on it? In what direction would the water in the container push on the droplet?” With some guidance to think about the directions in which air and water push on a tiny droplet right in the opening of one of the holes, the students conclude that the inside water must be pushing outward (sideways) on the droplet, since the droplet comes out. They also observe that the water comes out with different trajectories at the three different-elevation holes. They again see this as evidence that the deeper one goes, the greater is the push by the fluid, in this case sideways. I see some students capping the bottle and observing air going in (bubbles rising) the top hole while water is coming out the lower holes. They conclude that at the top hole, the outside air must push the hypothetical droplet into the water since that is the direction the air goes. Thus, they see that air and water can push sideways and that pressure is greater with depth.
Elaboration Activity A5: Water and Air in a Straw. I think most parents have been embarrassed by their children doing something like this activity while out to dinner. Students place a straw a few centimeters into a container of water and put a finger over the upper end of the straw before withdrawing the straw from the water. Typically, this results in a bit of air in the upper part of the straw and a few milliliters of water staying in the bottom part of the straw. Students are invited to explain.
In science, we strive for the simplest hypothesis necessary to explain the phenomenon. |
Observing that the water stays in the straw, some students conclude that the air below the straw helps support the water. Other students may suggest that the air or vacuum above the water may be “sucking” the water up, an alternative hypothesis. This latter hypothesis is probably cued by the situation because virtually all of these students have experienced sucking on a straw to get liquid to rise. Other students counter by turning the straw over while keeping their finger over the one end, now the bottom end. This leaves the water in what is now the top of the straw, with air in the straw below the water. One student suggests, “The air in the straw is now holding up the water. But, see how the water at the end of the straw now goes down a bit into the straw. That means the weight of the water is causing the air in the straw to be compressed slightly, and if we take our finger away, the air in the straw goes out and the water falls because it is not supported.” Other students chime in with their experiment of making two bits of water in the straw with a bit of air between them. They have a bubble of air in the bottom of the straw with a bit of water next, then a bubble of air, and finally more water in the top of the straw. These students argue that the middle bubble of air is both pushing up on the bit of water above and pushing down on the bit of water below. The sucking hypothesis, although not completely eradicated, seems less necessary. Thus, most students come to the conclusion that air can push up and down at the same time.
The first time I tried these activities, I had planned them as a “circus lab.” After about 10 minutes, I told students to move on to the next station. Most students stayed where they were. It was 40 minutes before I could get the three girls at the straw station to give it up. I now allow students to major in one activity and visit the others. They get engaged in these simple, common activities, and challenged, they need time to come up with and test explanatory ideas. So I now plan for students to have two class periods in which to complete their major activity, briefly visit each of the other activities, and prepare to present their findings to the class. Toward the end of the second period, we may begin class presentations.
On a third day, we finish the presentations and have a class discussion about what we learned. We summarize the similarities and differences in the properties of air and water. Virtually all students now agree that air and water can push up and down and sideways, that is, can push in all directions. Virtually all agree that the deeper one goes in water, the greater is the push in all directions. There is not quite the same strength of agreement that the push by air is greater the deeper one goes. But usually some students will note that the higher one goes up a mountain, the lower is the air pressure. Other students agree with this observation and add their own, such as that this is why airplanes are pressurized. So, they argue, air and water have many similar properties. Students now have sufficient background for me to introduce the technical term “fluid” properties. Both air and water are fluids.
They can exert pressures in all directions, and they appear to have this increase in pressure with depth.
After students have had experiences and come up with ideas to summarize those experiences, it makes sense to introduce a technical term for ease of communication. |
What are the differences? With some guidance, students suggest that air is “squishable” and water is not. They know that water is denser, heavier for the same volume, for we studied density earlier in the year. Students also may talk about the stickiness of water to itself and to other things, like the containers it is in. Since the students have summarized the ideas, I can now introduce the technical terms “cohesion” and “adhesion.” Now they are ready for another elaboration activity that more closely approximates the initial benchmark activity.
Elaboration Activity A6: “Weighing” an Object in a Fluid Medium. In this activity, I weigh a solid cylinder suspended by a string and ask, “What will happen to the scale reading if I attempt to weigh this object while it is under water?” Virtually all students suggest the scale reading will be lower than when the object is weighed out of the water. They are given an opportunity to test their predictions and are then encouraged to explain the results.
When complex explanations involving several factors are needed for their reasoning, students need more time to put the pieces together. |
The scale reading is lower. Some students conclude that the water is pushing up by an amount that is the difference between what the object weighed when out of the water and when in the water. Note, however, that this is going back to the conclusion that water pushes up, with no mention of any downward push. Many textbooks let students off the hook at this point: “This upward force by the water is called the buoyant force.” But this prevents a deeper, more useful understanding involving the resolution of the up and down forces, so I press for more: “Tell me about the pushes by the water on this solid, metal cylinder.” Several students jump in with claims based on their previous experiences. They introduce their earlier conclusion that the water is pushing on the top and sides as well as on the bottom. I probe for more: “In what directions are those pushes?” Now students are even more eager to apply the ideas that have emerged in the last few days.
A few students say, “The water on top pushes down.” Several others add, “The water below pushes up. The water on the sides pushes sideways.”
I now ask, “So, how do we explain the observation that the scale reading is less?” Several students are now constructing an explanation. I give them a few minutes to work on their explanations in small groups and then ask them to share their conclusions: “The water pushes up and the water pushes down. But the push up is greater than the push down, ‘cuz it is deeper.” Some students have it, but others are still struggling. If students do not volunteer consideration of the comparison between the pushes, I may ask the question, “Why should the push from below be larger? Why does that make sense?” Several students respond, “Because the deeper we go the bigger the push.”
At this point, several students have represented the application of our recently derived ideas with words. In the interest of deepening the understanding for all students, I suggest they represent the situation with pictures, using arrows to show the directions of the forces and varying the lengths of the arrows to show the magnitude (size) of the forces. I ask each group to take white board and a marking pen and draw such a picture of the submerged metal cylinder. After a few minutes, we compare diagrams and have members of each group describe their drawings and explain the situation. By now, nearly all the groups have drawn the cylinder with a larger arrow up than down. Each of these arrows, they say, represents the size and direction of the push by the water on that part of the cylinder.
Building an Analogy to Understand the Benchmark Experience
Now that it appears the students understand the weighing-in-water situation, I direct them back to the weighing-in-a-vacuum situation. “So, what does all of this tell us about the situation of weighing under the bell jar? If we had a really accurate instrument, what do you think would have happened to the scale reading and why?” A few students begin to construct an analogy: “Weighing in air would be like the weighing in water.” I ask, “How so?” One student responds, “The air around the world is kind of like an ocean of air. Down here is like being deep in the ocean of air. On a mountain air doesn’t press as hard.” Another adds, “Air can push in all directions, just like water. So if water can push up and down on the cylinder, so can air.” “But air doesn’t push as much [hard], so you don’t get as big a difference,” says another. With some guidance from me, the students build an analogy: “Weighing in the water is to weighing out of the water (in air) as weighing in the ocean of air is to weighing out of the air, that is in a vacuum.” I ask, “So what would happen to the scale reading in the vacuum if we had a very accurate instrument?” One student responds, “The scale reading would be more.”
Another adds, “Just like the scale is more when we take the thing out of the water.”
Building an analogy from a situation students understand to one they do not can build understanding of the new situation. |
Consensus Discussion and Summary of Learning
There are expectations for what students should have learned from the curricular activities performed thus far. Up to this point, I have been attempting to identify students’ understandings about the pushes of the surrounding fluid (water or air). In the class, I now guide a discussion aimed at achieving consensus on what we can conclude about water and air from our observations. On the topical content side, learners should know the following:
-
Water and air have some similar properties.
-
Fluids (at least water and air) can push in all directions, up, down, sideways.
-
The deeper one goes in the fluid, the greater is the push in any direction.
-
-
Water and air have some different properties.
-
Since water is denser than air, the effects of the pushes by water are greater.
-
Water can stick to itself (cohesion).
-
Water can stick to other materials and things (adhesion).
-
Air is more squishable (compressable) than water.
-
The learners should have evidence (results) from the class experiences that they can use to support each of these conclusions.
Students need opportunities to reflect on and summarize what they have learned. |
Learners also have had an opportunity to practice some habits of mind that are consistent with learning and reasoning in science:
-
Inferences come from observations (evidence-based reasoning).
-
Controlled experiments can be used to test most of our ideas.
-
Dialogue in science means questioning for clarity of observations, ideas, and explanations.
-
Dialogue in science means being supportive and encouraging to elicit the ideas of others while at the same time asking critical questions, such as “How do you know?”
-
If we persevere, we will likely be able to understand complex situations.
Students need opportunities to monitor their own learning. |
If habits of reasoning and action are also among our learning goals, we need to make them as explicit as we make our content goals.
Diagnostic Assessment
At some point after the benchmark lesson and the more focused elaboration lessons, and after the class has begun to develop tentative resolutions for some of the issues raised, it is useful to give students the opportunity to check their understanding and reasoning individually. Although I sometimes administer these questions on paper in large-group format, I prefer to allow the students to quiz themselves when they feel ready to do so. They think they understand, but they need opportunities to check and tune their understanding. To address this need for ongoing formative assessment, I use a computerized tool2 that assists the teacher in individualizing the assessment and keeping records on student progress. When students feel they are ready, they are encouraged to work through computer-presented questions and problem appropriate to the unit being studied.
Typical questions related to the key ideas of the preceding activities might juxtapose three situations involving weighing a solid object—the solid object in air, in water, and in a vacuum—each object suspended from a string attached to a spring scale. A first question checks on the students’ recall of the specific results obtained and asks them to put the expected scale readings in order assuming the scale has the precision needed. A second question checks on the students’ reasoning: “What reasoning best justifies the answer you chose?” For this question, I look for responses that suggest “the water pushes up,” “the air pushes down,” or “air has no effect on scale readings.” Have the students fallen back into their preconceptions, or have they made the desired progress?
Other questions extend the students’ application of the ideas to new contexts: “Using the ideas of pushes by air and water, explain how the squeezable plant watering container (with the long curved ‘straw’ on top) works.” Another question suggests a special room wherein the air pressure
can be increased from normal to much higher: “What would happen to the scale reading and why?” Another asks students to predict what would happen to a scale reading if we attempted to weigh the solid object in alcohol, which is less dense than water but more dense than air.
Assessment should help the teacher monitor whether students are still operating on the basis of preconceptions, as well as whether they have attained the learning goal(s). |
For all these questions, I look for evidence to determine whether the students’ ideas have changed or they are still showing evidence of believing their original idea that fluids only push up or down or that the weight is proportional to air pressure. Thus, I aim to move students’ understanding across the gap from their preconceptions to a more scientific understanding. The assessment allows them to monitor their learning. If there is trouble, they get feedback suggesting they rethink their answer and/or reasoning in light of the class experiences. I thus obtain a report of what sort of problematic thinking students have exhibited and what experiences might help them move farther across the learning gap.
Part B: What Is Gravitational Attraction?
Exploring Similarities and Differences Between Actions at a Distance
In the previous subunit (Part A) the class separated the effects of the surrounding medium from the effects of gravity on static objects. We appear to have taken a bit of a detour into understanding more about the effects of air and water and other fluids on objects submerged in the fluid. Later we will need to return to looking at the effects of the surrounding fluid when we explore falling bodies (Parts C and D). First, however, we explore the concept of “action at a distance,” a key notion in understanding gravity.
Students should be able to see science as involving many questions as yet unanswered. |
Although there are still many unanswered questions about gravity, the students do know a great deal about what it does and about the variables on which the strength of the gravity force depends. In Part B, now that the students know about some effects that are not due to gravity, we explore some of the effects that are. Because many effects of gravity are so subtle
and pervasive (we live and deal with them every day), the students need to explore gravity by comparing and contrasting its effects with some similar effects and causes they can investigate first-hand.
Research has shown that many students do not separate gravitational effects from magnetic or electrostatic effects. But the effects are similar in that they are all “actions at a distance”; that is, one object can affect another without touching it. Actions at a distance can act through materials and even across empty space. The first activity (B1) in this subunit is to construct analogies among the various actions at a distance. The goal is for students to see that the situations are similar, but the properties of the objects or materials on which the influencing objects act are different.
Benchmark Lesson: Making a Torsion Bar Do the Twist
In the classroom, several meter sticks are hanging from their center points from strings attached to the ceiling. They should be hanging so that each meter stick is horizontal and free to rotate horizontally. On the two ends of one meter stick are hanging two identical brass spheres. From the ends of another hang two aluminum spheres, from another two wooden spheres, from another two steel spheres, and from another two foam spheres. Each system should hang fairly still with the meter stick horizontal (though this is sometimes difficult with students moving around the room). Each is arranged to be what is called a “torsion balance” or “torsion bar.” The word “torsion” comes from “torque,” which means twist. So, we are going to see whether these bars can be made to twist by bringing something near the objects hanging from the ends without touching the objects (see Figure 11-2). Care must be taken not to bump or even touch the bars except to adjust them to remain still at first. Note that, depending on the maturity and coordination of the students, it will likely be necessary to set up and run the experiment as a demonstration after students have made their predictions individually. Some teachers have found that it helps keep the torsion bars still if movement of students around the room is limited, and even the

FIGURE 11-2 Torsion bar, spheres, and influencing material.
heating/cooling fan for the room is turned off and windows and doors closed. Even quick hand motions near these delicate balances will cause them to move—possible air effects again. Now the problem:
Suppose one end of a magnet is very slowly brought near (but not touching) a sphere on one end of each torsion bar. (Notice that there is a similar situation on the other end of the torsion bar. We will be discussing mainly what happens at one end of the bar, but because of symmetry we can generalize the effect to both ends.)
1. Predict what the torsion bar will do in each case. If you think the bar will twist, tell whether it will go toward or away from the magnet.
When brought near the brass sphere the bar will
_______________.
When brought near the aluminum sphere the bar will
__________________.
When brought near the wooden sphere the bar will
____________________.
When brought near the steel sphere the bar will
____________________.
When brought near the foam sphere the bar will
_________________.
2. Briefly explain how you decided which will twist and in what direction.
If any will not twist, tell why you think they will not.
To keep students thinking, teachers should not give answers but present opportunities for students to test their answers. |
While students are answering the questions individually, I circulate around the room, making sure that they understand the questions and that I am getting a feeling for the sorts of answers and thinking I will hear during the discussion. When it appears most students have finished answering and explaining, I ask them to share their predictions and explanations with others. One student suggests, “I think all the metal ones will move because metal is attracted to magnets.” I ask whether that makes sense to others, and most of the class appears to agree. Another student says he has tried this before and only “silverish” metal things get attracted, not things like gold rings. Another says, “Not all silver things are attracted, ‘cuz I’ve tried to pick up money, and magnets don’t pick up quarters, nickels, or dimes.” A few others agree. After only a few minutes of this discussion, students are ready
to see what happens. So I carefully and slowly bring the magnet near one of the spheres in first one situation and then another until we have tried them all. While doing this, I suggest that the students write down the results of each situation. The results are that only the steel sphere is attracted to the magnet, and none of the others are affected.
In this case, because the students typically know little about various kinds of metals and their properties and because I do not want to lose the focus on actions at a distance, I elect to tell the students about the metals that are attracted to magnets. I suggest that, while most materials have some magnetic properties, only metals containing iron readily show the effects with magnets such as those we are using.
Preinstruction assessment should check for specific preconceptions. |
Next I bring out a styrofoam cup that I have been careful to leave in my desk for several days, so it likely will not be electrostatically charged. I ask the students to predict whether the cup will affect any of the spheres on the torsion balances. There are no clear patterns of prediction. Most students appear to be just trying to guess. I immediately show them what happens: the foam cup does not affect any of the objects (unless the spheres themselves happen to have become charged electrically).
In guided inquiry, the teacher needs to monitor class ideas as they exist initially and as they develop. |
Then I rub the cup across my hair a couple of times and ask the students what they expect will happen now. Some students say they think the cup will attract the steel “because you magnetized the cup.” Others say no, that the cup now has “electrical charge,” so it will attract all the metal pieces because “metal conducts electricity.” Still others say, “Because the metals conduct the charge away, they won’t be affected.” Again, given the confusion and, in some cases, lack of experience with the phenomena, it is time to move quickly to doing the experiments. So I bring the charged cup near each sphere. The results are that every sphere is attracted to the cup. One student facetiously suggests, “That’s static cling.” For now we conclude that all materials are attracted to an object that has been electrostatically charged. In later investigations of electrostatics, I want students to see that there are two kinds of electrostatic charge and the neutral condition, but I elect not to encourage that investigation at this time so we can keep building the action-at-a-distance story.
Next, I “cuddle” the cup in my hands. By gently putting my hands all over the cup and breathing warm, moist air on it, I am discharging it. When I am pretty sure it has been discharged, when I see that it will not pick up a tiny scrap of paper, I go through the test of bringing the cup near but not touching each sphere again, and we find that there is no effect on any of the spheres. (Note that this part of the lesson is tricky, and it takes practice to make sure the cup is no longer charged.) So I cuddle the magnet as well, but it still attracts the steel sphere and no others. I rub the magnet across my hair and test it, again with no effect except with the steel sphere.
Students need opportunities to summarize the big ideas that have been developed by the class. |
It is now time to have students summarize and build consensus. Magnets attract steel pieces without touching them, but do not affect any other materials that we can readily see. And the magnet effect cannot be cuddled off. Static-charged foam cups (and other things such as plastic rulers and inflated latex balloons) attract all kinds of materials without touching them, but the charge can be cuddled off. Thus magnets and static-charged objects are similar in that they both influence other things without touching them, and I suggest this is called “action at a distance.” I continue to point out that the two phenomena are apparently different kinds of action at a distance, since they affect different kinds of material. Magnets affect materials that contain iron, but static-charged things can affect almost anything made out of almost any material. Finally, the electrical charge can be cuddled off, but the magnetic effect cannot.
Technical media can be used to enhance or extend the students’ experience. |
What about gravity? It also acts without touching. The students have heard slogans about gravity making things fall here on earth, holding the moon in orbit, and holding the planets in orbit around the sun, but how can I make that abstraction real for them? What does gravity affect? Can it be cuddled away?
Thinking needs to be challenged whenever passive media are used. |
I show a piece of film that demonstrates a torsion balance experiment similar to what we have been observing during the first half of the class period. In the film, a meter stick is again used as the torsion bar. In this case,
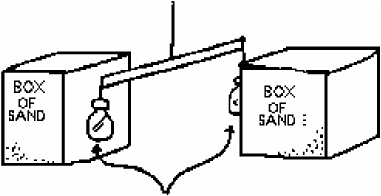
FIGURE 11-3 Experiment illustrating gravitational torsion balance.
quart milk bottles are hung from each end of the bar and adjusted until the bar is horizontal and remaining still. Then a large cardboard box of sand is pulled close (but not touching) to one side of one bottle, and another box of sand is pulled to the other side of the balance but near the other bottle of water (see Figure 11-3). To help students understand the film, I stop it and simulate the situation in one corner of the classroom with bottles and boxes. Then the students watch the film as the bar slowly twists such that the bottles get closer to the boxes. Because the effect is so unbelievable to students and because an indirect measure of the movement of the bar is used in the film, I talk the students through the procedure, the results, and the final conclusion:
Teacher |
Do you understand the procedure? How is it like the procedure we used for the magnet situation and the electric charge situation? |
Student 1 |
There are things hanging from the stick in all of ‘em. |
Student 2 |
The stick could turn if something made it turn, like a magnet or rubbed foam cup. |
Teacher |
What is brought into this situation like the magnet or the charged cup? |
Student 3 |
A box of sand? |
Student 4 |
Two boxes of sand. |
Teacher |
So is there an effect here? |
Student 3 |
No, it is not like the magnet, a box of sand can’t cause the bottle to move. |
Teachers can foster students’ thinking by asking questions, by reflecting students’ comments back to them, and by avoiding expressing judgments about whether those comments are right or wrong. |
|
[Partly because students do not believe there should be an effect, and partly because the film uses a subtle way of detecting the movement, most students fail to see the movement at first. I tape a small piece of mirror to the middle of the meter stick with the bottles and shine a flashlight on the mirror. This is also done in the film. A spot of reflected light hits the wall over my shoulder.] |
One goal of inquiry-based teaching is to get students to be the ones asking the questions and challenging or bringing up apparently conflicting observations. |
Teacher |
What would happen to the spot of light if the meter stick twisted? |
Student 5 |
The spot would move. It’s like when the light hits my watch and makes a spot and then I move my wrist and make the spot hit somebody in the face. [some laughter] |
Teacher |
Yes, good example, although it might be distracting, so please don’t do it in class. In the film they were shining a light on the mirror in the center of the meter stick. What happened to the light? |
Student 5 |
It moved. |
Student 6 |
Yeah. The light went first one way, then the other. |
Student 2 |
But that would mean that something like a magnet made the stick turn. |
Student 4 |
The box of sand pulled on the bottle of water and made the stick turn. |
Student 7 |
No way, Jose! [laughter] Sand can’t pull on water. |
Student 3 |
Yeah maybe they had a magnet or foam cup or something to do it. |
Teacher |
Good question, lets see how they address that in the film. |
|
[I run the film through the part where they show the effect is not an electrical one, and the voice of the physicist on the film concludes that the bottles moved because of the gravitational attraction between the sand-filled boxes and the bottles of water.] |
Student 3 |
But, how come we can’t see that [gravitational attraction] here with our stuff? |
Teacher |
Another good question. The gravitational effect of a box of sand on a bottle of water is so weak that it requires a very delicate setup. Although Sir Isaac Newton, in 1687, suggested every object in the universe pulled on every other object in the universe, it really wasn’t until about a hundred years later that another scientist named Henry Cavendish built a very sensitive torsion balance and was able to see evidence of gravitational attraction happening with ordinary things in a laboratory. |
Providing some information from the history of science can help give students perspective on human involvement in the development of ideas. |
|
[When I was at the university I had a chance to repeat Cavendish’s experiment. The equipment was so delicate that when a truck went by the building I was in, we had to start the experiment over again. It made the equipment shake, even though we could not even feel or hear the truck. Note that in the film, the experiment is conducted in a mostly vacant building, and the torsion bar is hung from the rafters.] |
Teacher |
OK, so this was about the best I could do to show you that any thing that has mass will pull on any and every other thing that has mass. This is part of Newton’s law of universal gravitation. Even ordinary things like boxes of sand and bottles of water exert a gravitational pull on each other, and they do it without touching each other. Gravity is also an action at a distance. Can we rub it off? No, not unless we could get rid of the mass. But, then we would have nothing, because everything has |
|
mass. Let’s summarize in a table [which I write on the board and encourage them to record in their notes for the day]. |
Teacher |
So remember these three different sets of |
Three different kinds of action at a distance: |
|||
Magnetism |
Acts at a distance |
Acts mostly on iron things |
Can’t be cuddled away easily |
Static electricity |
Acts at a distance |
Acts on anything (charged or neutral) |
Can be cuddled away |
Gravity |
Acts at a distance |
Acts on anything (with mass) |
Can’t be cuddled away at all |
|
circumstances associated with three different forces that can all act at a distance, even across empty space. We conclude that they all three are “actions at a distance,” but they act on different materials. Some we can make come and go under certain circumstances (e.g., cuddling, and I will end class with changing a magnet). So far we have no way of making the gravity go away. And we have some evidence that you might encounter in later classes that gravity is the force that holds planets in their orbits and makes dust and gases in the universe come together to form stars. |
If students have had sufficient first-hand experience, short lectures can make sense even to young students. |
Mainly for fun and motivation, I show the students that if I beat on an iron bar with a hammer while holding the bar parallel to the earth’s magnetic field, I can cause the bar to become a magnet. For fun, I have them chant as I beat on the bar: [bang bang] “uwa,” [bang bang] “tafu,” [bang bang] “yiyam.” As I beat the pairs of hits faster and faster, the chant begins to sound more and more like “ohwhat afool Iam.” The bell rings, and the
students leave laughing and agreeing with the chant. That concludes the main lesson showing the various types of actions at a distance.
Humor can enliven the learning experience and help build positive relationships between students and teachers. |
Factors on Which the Magnitude (Size) of Gravitational Force Depends
The purpose of the next series of lessons is to build a case for students to believe that the magnitude (size) of the gravitational force grows as each of the two interacting masses becomes larger, and that the greater the separation distance between the two masses, the smaller is the gravitational force that each exerts on the other. High school physics students and more mathematically capable middle school students may be able to conclude with analogous experiences from magnetism and electrostatics that the dependence on distance of separation is an inverse square law. For middle school students, teachers can be more successful building cases that yield qualitative relationships as opposed to yielding mathematical relations and especially equations. The following activities include first-hand observations, reasoning from results to formulate conclusions, and analogical reasoning from concrete situations to abstractions not readily accessible through classroom experiences. From these more qualitative experiences, later algebraic formulation of the gravitational force law can make more sense to students.
We saw early in this unit that gravity does not depend on air pressure pressing down on an object. From other prior experiences, students know that we can measure the weight of something fairly precisely using a spring scale (see Figure 11-4). The heavier the object, the greater the spring scale

FIGURE 11-4 Common experiences using a spring scale to weigh objects.
reading will be. But what is the cause of that reading if it is not air pressing down? I could just wave my hands and suggest “gravity,” but I want students to have a deeper understanding of gravity by at least understanding the factors on which it depends. I build the case for factors affecting gravity by determining factors that affect magnetic force, and then arguing by analogy about factors affecting gravity.
Students generally love playing with magnets, especially strong ones. I recommend having at least one strong magnet available for each physical science classroom. Among other experiences set up for students’ investigations with magnets, one station has a spring scale firmly attached to a heavy brick on a table. A string is tied to the hook on the spring scale. The other end of the string has a loop on which to hang one or more identical paper clips (see Figure 11-5). Set up properly, the magnet attracts the paper clips and the string pulls on the spring scale, registering a reading even without the magnet touching the paper clips. The teacher might ask, “How can the magnet do that?” Most students from the earlier lesson see that the magnet is exerting a force at a distance.
Answering such questions as “How do you know?” or “Why do you believe?” helps students build understanding of how knowledge in the discipline is constructed. |
Teacher |
What kind of action at a distance is the magnet exerting, and what kind of material does it affect? |
Student 1 |
Magnetic. |
Student 2 |
Magnetism. |
Teacher |
How do you know? |
Student 3 |
‘Cuz the paper clips are made of iron. |
Teacher |
Did any other action at a distance affect iron? |
Student 3 |
Yeah. Electric force. |

FIGURE 11-5 Apparatus for testing factors that affect magnetic force.
Student 4 |
And gravity because the paper clips have a little mass. |
Student 1 |
But, I know in this case it’s magnetic because there is a magnet. |
Student 2 |
Also, when we set it up we touched the paper clips a lot and that’s like cuddling, so there shouldn’t be any electric force. |
Teacher |
Very good. So, we’re pretty sure it is magnetic and not electric force. And we are pretty sure it is not gravitational because gravity force is so weak. |
Student 3 |
Yeah, we know all that without even talking about it. |
Student 2 |
So, what’s the point? |
I see now that I am losing their attention, so I elect to demonstrate the apparatus initially myself. I set it up so there are four paper clips being pulled by but not touching the magnet, and I ask one student to read the spring scale reading and record the reading on the board. I ask another student to carefully measure the distance between the paper clips and the magnet. (Note we are just measuring the separation distance here. With more mature students, we could get into concerns about measuring center-to-center distance as necessary for the force equation.)
I then ask what we might do to make the scale reading lower. Upper elementary and middle school students’ intuition suggests that using a weaker magnet would make the scale reading lower. Some also suggest that if we had fewer paper clips, that might do it, too. Other students suggest we might need more paper clips to lessen the force. Since no one has mentioned the separation distance, I ask how it might make the scale reading lower. I ask the students to answer the question for themselves first without saying their answers out loud, so everyone has a chance to do the thinking. Most students suggest moving the magnet farther away will decrease the force and the scale reading.
Quiet can allow each student time to do his or her own thinking. |
It appears that the students are now ready to test their predictions and hypotheses. We try a weaker magnet and fewer paper clips, but I allow the distance to get smaller as well. The scale reading rises. Although this is confusing, I want to give students an opportunity to notice and suggest the need to control variables without my having to tell them to do so.
Sometimes, there are emergent goals that need to be addressed before returning to the primary instructional goal. For example, teaching about the content may need to move to the background of the instruction while teaching about the processes of science are brought to the foreground, even though both are always present. |
Student 2 |
See, it’s what I thought, less paper clips makes it stronger. |
Student 3 |
No it’s what I said. Smaller distance makes it bigger. |
Student 4 |
We got too many things happening. |
Student 1 |
I’m getting lost. |
Student 3 |
It’s like we studied before about making fair tests. This isn’t a fair test. |
Student 4 |
Oh yeah. |
Teacher |
OK. Why not, Chris? Why isn’t it a fair test? Hang in there Tommy [Student 1]. I think we are about to clear this up. I will have you decide when the argument and results of the experiments make sense to you. The rest of you need to talk to Tommy to convince him of what you are saying. Chris, you were saying? |
Student 3 |
You gotta keep things constant. Like change only one thing and keep other things constant. |
Student 4 |
Oh yeah, like we did before, make a fair test. OK, Tommy? |
Student 1 |
No, I don’t remember anything about a fair test. |
Student 4 |
It’s like when we said we have to keep all the things [a few students are saying “variables”]. Yeah, we have to keep all the variables the same except one. |
Teacher |
But, does that help you, Tommy? |
Student 1 |
Not really. What’s it got to do with this experiment? That was something we did before when we were studying other stuff. |
Student 3 |
In this experiment we have to keep the number of paper clips the same and the strong magnet the same and change the distance. Only change the distance, if we want to see whether |
|
the distance changes the scale reading. Otherwise, if we change other things too, we will not know whether it is distance or something else that made it bigger. |
To become learners, independent of authority, students need opportunities to make sense of experiences and formulate rational arguments. |
Student 1 |
OK. So, what happened? |
Student 3 |
Well, we didn’t keep the other things, variables, the same. So, we need to do that to find out what happens. |
Teacher |
Good, to find out whether that one variable, for example the distance, affects how big the magnetic force is. [At this point, because the apparatus is difficult to control, I demonstrate what does happen when we keep the big magnet and the number of paper clips the same and just decrease the distance between the magnet and paper clips. The scale reading rises.] Now can we tell if varying the distance affects the force? |
Student 2 |
Yeah. It does. |
Teacher |
How does distance affect force, Tommy? Which way does it go? The smaller the distance … |
Student 1 |
The smaller the distance, the bigger the force. Does it get smaller if the distance gets bigger? |
Teacher |
Good question. Let’s try it. [I increase the distance, and the scale reading is lower.] So, what can we conclude now? |
Student 1 |
The bigger the distance the smaller the scale, and the smaller the distance, the bigger the force scale. |
Teacher |
Good. Now, what do we need to do to test whether the number of paper clips makes a difference in the force? |
Student 1 |
Would we change the paper clips or keep them the same? |
Student 2 |
If you want to test the paper clips, you change the number of paper clips and see if that changes the force. |
Student 1 |
Is that right? Oh! Oh! I get it. So to see if one thing affects the other thing, you change the one thing and see what happens to the other. |
The teacher’s questions to clarify students’ statements help the students become clearer about what they know. |
Teacher |
That’s sounding like you’ve got the idea of fair test or what is sometimes called “controlling variables,” but could you say it again and say what you mean by the word “thing,” which you used several times. |
Student 1 |
OK. To see if paper clips affect the scale, the force, you change the number of paper clips and see if the force changes. Right? |
Teacher |
Yes, good. Now suppose you wanted to see if the strength of the magnet affected the force. What would you do? |
Student 1 |
Change the magnet and see if the force changed. |
Teacher |
What would you do about the other variables? |
Student 2 |
I’d keep … [At this point I interrupt to let Tommy (Student 1) continue his thinking. Meanwhile, other students are getting restless, so I let them go ahead with the apparatus and see what they can find out, which I charge them with demonstrating later for the rest of us. Meanwhile, I continue with Tommy and anyone else who admits to needing some help here.] |
All students can learn, but some need more assistance than others, and some need more challenge than others. |
Teacher |
So, Tommy. What are the factors that we want to investigate here? |
Student 1 |
See if bigger magnets have a bigger force. |
Teacher |
OK. Anything else? |
Student 1 |
See if more paper clips makes the force reading bigger. |
Student 5 |
And see if distance makes the force bigger or smaller. |
Student 1 |
We already saw that one. |
Teacher |
If we changed the number of paper clips and we changed the magnet, would we know whether one of these affected the force? |
Student 6 |
Not if we changed both. If we changed both, one or both might be changing the force. |
Teacher |
So, what do we need to do, Tommy? |
Student 1 |
Oh, do we need to only change one thing, like change the strength of magnet we use and don’t change the paper clips? |
Student 6 |
And we’d need to keep the distance the same too right, else that might be changing the force too? |
Teacher |
Good. So, we think that strength of magnet, the number of paper clips, and the distance might all change the magnetic force. So we just change one of those variables at a time and keep the others constant and see if the force changes and in what direction. |
Assuming all the students are familiar with the equipment, sometimes it is more important to help some students focus on the argument while others wrestle with the details of manipulating the equipment. |
In a while, I bring the whole class together. I help the students summarize the ideas they have developed and how the controlled experiments helped test those ideas. The group that had the challenge to test factors demonstrates the apparatus and the procedures they used to obtain the following results:
-
The more paper clips, the higher the scale reading (keeping magnet and distance constant).
-
The stronger the magnet, the higher the scale reading (keeping number of paper clips and distance of separation constant).
-
The greater the distance of separation, the lower the scale reading (keeping number of paper clips and strength of the magnet constant).
Students need assistance in differentiating between results and conclusions. Results are specific to the experiment, while conclusions generalize across situations. |
From these results we conclude that the magnetic force grows larger with more magnetic “stuff” (paper clips containing iron), with a stronger magnet, or with closer distance of separation between the big magnet and the iron pieces.
Building a Bridge from Understanding Magnetic Action at a Distance to Understanding Gravitational Action at a Distance
Analogies can help bridge from the known to the unknown and from the concrete to the abstract. |
I now illustrate two situations on the front board. One is something like the situation we have just investigated, with a large magnet pulling on an iron object and stretching a spring scale. Since this diagram is a bit different from the previous one, I ask students to discuss the similarities and differences. When they appear to see that the situations just seem to be different representations of the same conclusions we drew, I move on to the second diagram. It looks like the first, except that a large sphere represents the earth, and the object is anything that has mass (see Figure 11-6). The spring scale is the same. I ask students how this situation is similar and different from the weighing of a fish depicted in Figure 11-4.
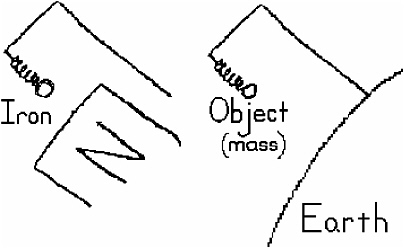
FIGURE 11-6 Diagramming an analogy between magnetism and gravity.
Student 5 |
Oh, it’s like, the earth pulls on the object like the magnet pulls on the piece of iron. |
Student 7 |
They are both actions at a distance. |
Student 4 |
So what. We already knew that. |
|
[So the students appear to recognize the analogous situations. Now comes the difficult part.] |
Teacher |
From our previous experiments you know on what factors the magnetic force depends. Right? |
|
[There is a chorus of “yes,” but I don’t trust it because we now have a different diagram, and I want to know if the students are transferring what they know about the previous situation. Students recite the list: “how much iron,” “how big (strong) the magnet is,” “how far apart they are.” Now reasonably assured, I move on.] |
Teacher |
What are some possible factors on which gravitational force might depend, if it acts similarly to magnetism? |
Student 2 |
Oh. Maybe it depends on the separation distance? |
Student 8 |
Maybe on the mass of the thing, ‘cuz that would be like the number of paper clips. |
Student 1 |
Maybe on the strength of the magnet. |
Student 3 |
No, there is no magnet in the gravity situation. |
Teacher |
OK. Hang on. Tommy [Student 1], there is no magnet in this situation [pointing to the gravitational case], but what might be similar to the strength of the magnet? |
Student 1 |
The strength of the earth? |
To build deep understanding of ideas, students need opportunities to transfer the ideas across contexts. Teachers need to check on this transfer of knowledge to new situations. |
Teacher |
It is kind of like the strength of the earth isn’t it. Just like the magnetic force depends on how big and strong the magnet is, the gravitational force might depend on how big, how much mass there is in the earth. Just like the more magnet we have, the bigger the force; the more mass the earth has, the bigger the force. I cannot easily show you, with experiments, on what factors the gravity force depends. But by what is called an “analogy,” we can make a good guess at the factors gravity depends on. If gravity action at a distance acts like magnetic action at a distance, it should depend on how much there is of each of the two objects interacting and on how big the separation distance is. By careful experiments with sensitive apparatus like the Cavendish torsion balance we saw before, scientists have verified that the guesses we just made work out in experiments. That is, the gravity force, evidenced by the spring scale reading, would be smaller if the mass of the earth were smaller, if the mass of the ball being held near the earth were of less mass, or if the ball were placed farther away from the earth. |
Parts C and D: What Are the Effects of Gravity?
Explaining Falling Bodies
Part A was about “what gravity is not.” That is, the effects of the surrounding fluid are not the cause of weight or gravity. But we ended up seeing that fluids such as air and water can have an effect on scale readings when we attempt to weigh objects. Part B was about the nature of gravitational force being one of the actions at a distance. And by analogy we concluded that the magnitude of the gravitational force depends on the masses of the two interacting objects and on the separation distance between them. Investigations into the nature of forces could stop here or could continue and focus on gaining a better understanding of the effects of gravity.
Subsequent investigations in my classes involve explaining the phenomena of falling bodies. Part of a rich understanding of falling bodies is to understand the effects of air (or fluid) resistance as well those of gravity.
Activities in these subunits are more consistent with what is presently suggested in curricula, so they are not described here. But students’ preconceptions, such as “heavier falls faster,” need to be addressed. More mature students can also quantify the acceleration of freely falling bodies and arrive at equations describing the motion in free fall. But younger students can gain a qualitative understanding of free fall as speeding up uniformly, and they can gain some understanding of factors affecting air resistance.
Explaining Motion of Projectiles
Next investigations, especially for older students, can involve understanding the motion of projectiles. Preconceptions, including “horizontal motion slows the vertical fall,” will need to be addressed. Understanding the independence of horizontal and vertical motions is a learning goal. Again those activities are not discussed in detail here. Suffice it to say that additional investigations into the nature and effects of gravity will build a stronger relationship between ideas and increase the likelihood that what is learned will be understood and remembered.
SUMMARY
In this chapter, we have tried to make real the principles of How People Learn by writing from our experience and the experience of other teachers, researchers, and curriculum developers. The sequence of activities described is not the only one that could foster learning of the main ideas that have been the focus here. Likewise, the dialogues presented are just examples of the many conversations that might take place. Teaching and learning are complex activities that spawn multiple problems suggesting multiple solutions. What we have discussed here is just one set of solutions to exemplify one set of generalizations about how students learn.
That having been said, the activities described are ones that real teachers are using. But this chapter has not been just about activities that teachers can take away and use next week. Our main purpose is to give teachers and curriculum developers an idea of what it looks like when assessment, curriculum, and teaching act as a system consistent with the principles of How People Learn. We have tried to give the reader the flavor of what it means to teach in a way that is student-centered, knowledge-centered, and assessment-centered. By looking at the teacher’s decision making, we have attempted to provide a glimpse of what it is like to be a teacher or a learner in a learning community that is respectful of members of the community while at the same time being critical of the ideas they voice. Students are encouraged to question each other by asking, “What do you mean by that?” “How
do you know?” But they are also guided to listen and allow others in the community to speak and complete their thoughts.
Students’ preconceptions are identified and addressed, and subsequent learning is monitored. This means assessment is used primarily for formative learning purposes, when learning is the purpose of the activities in the classroom. By listening to their students, teachers can discern the sorts of experiences that are familiar and helpful in fostering the learning of other students.
Learning experiences need to develop from first-hand, concrete experiences to the more distant or abstract. Ideas develop from experiences, and technical terms develop from the ideas and operations that are rooted in those experiences. When terms come first, students just tend to memorize so much technical jargon that it sloughs off in a short while. Students need opportunities to see where ideas come from, and they need to be held responsible for knowing and communicating the origins of their knowledge. The teacher should also allow critical questions to open the Pandora’s box of issues that are critical to the content being taught. The better questions are those that raise issues about the big ideas important to deep understanding of the discipline. Some of the best questions are those that come from students as they interact with phenomena.
Students need opportunities to learn to inquire in the discipline. Teachers can model the sorts of questions that the students will later ask themselves. Free inquiry is desirable, but sometimes (e.g., when understanding requires careful attention and logical development) inquiry is best guided, especially when the teacher is responsible for the learning of 30 or more students. But the teacher does not need to tell students the answers; doing so often short-circuits their thinking. Instead, teachers can guide their students with questions—not just factual questions, such as “What did you see?”, but the more important questions that foster student thinking, such as those that ask students to provide explanations or make sense of the phenomena observed. By listening respectfully and critically to their students, teachers can model appropriate actions in a learning community. Through questions, teachers can assist learners in monitoring their own learning. Finally, teachers also need the freedom to learn in their classrooms—to learn about both learning and about teaching.
NOTES
2. |
The computer-based Diagnoser assessment system described is available on the web through www.FACETInnovations.com. Thus, it is accessible to teachers and students anytime from a computer with web access and appropriate browser. The concept and program were developed by the authors, Minstrell and Kraus, Earl Hunt, and colleagues at the University of Washington, FACET Innovations, Talaria Inc., and surrounding school districts. It includes sets of questions for students, reports for teachers, and suggested lessons to address problematic facets of thinking. |