4
Remedial Investigation Assessment
INTRODUCTION
Superfund activities began in the Coeur d’Alene River basin in 1983 with the listing of the Bunker Hill Mining and Metallurgical Complex on the National Priorities List (NPL). This site, commonly referred to as the Bunker Hill “box,” encompasses a 21-square-mile area including the historic smelter and ore-processing operations in the heart of the Coeur d’Alene River basin. The site was divided into two operable units (OUs) for which records of decision (RODs) were issued in 1991 and 1992.1
In 1998, the U.S. Environmental Protection Agency (EPA) extended Superfund activities and undertook a remedial investigation/feasibility study (RI/FS) of mining-related contamination in the Coeur d’Alene River basin outside the box. This is the third operable unit of the site (OU-3, commonly termed the “basin”). The geographic area includes the Coeur d’Alene River, associated tributaries, Lake Coeur d’Alene, and the Spokane River that drains from Lake Coeur d’Alene and crosses from Idaho into Washington. Within this geographic scope are residential communities; recreational areas; active and inactive mining facilities; parts of the Coeur d’Alene Indian Reservation; the Spokane Indian Reservation; parts of Kootenai, Benewah, and Shoshone counties of northern Idaho; and parts of Stevens, Lincoln,
and Spokane counties in eastern Washington (see Figure 3-1 in Chapter 3 of this report).
The RI report (URS Greiner, Inc. and CH2M Hill 2001a) was prepared by contractors for EPA Region 10 based on EPA’s guidance document for conducting RI/FS studies (EPA 1988) through the RI process set forth in the National Oil and Hazardous Substances Pollution Contingency Plan (NCP, 40 CFR Part 300) (URS Greiner, Inc. and CH2M Hill 2001b, p. 1-2). The information in the RI report is used to evaluate risks to human health and the environment and potential remedial alternatives.
In this chapter, the RI of the Coeur d’Alene River basin (URS Greiner, Inc. and CH2M Hill 2001a) is assessed with respect to the following:
-
Adequacy and application of EPA’s own Superfund guidance for RIs
-
Consistency with best scientific practices
-
Validity of conclusions
Additionally, this chapter evaluates the scientific and technical aspects of the following:
-
EPA’s determination of the geographic extent of areas contaminated by waste-site sources
-
Types of data and analyses used to assess the extent of contamination
-
Approaches used to collect and analyze the data that resulted in conclusions
-
Considerations of contaminant chemical speciation and transport
Human health aspects of the RI are primarily evaluated in Chapter 5, “Human Health Risk Assessment in the Coeur d’Alene Basin.” The Human Health Risk Assessment (HHRA), undertaken concurrent with the RI, characterizes heavy-metal contamination in relation to potential human health risks.
EPA’S RECOGNITION OF THE BASIN SYSTEMS AND THEIR INTERACTIONS
The Coeur d’Alene River basin is a large-scale, complex system with extensive anthropogenic overprints that have increased the multiple complexities and interacting processes at work throughout the basin. This vast, mountainous river system has a long history of mining, logging, fishing, trading, and tourism (see Chapters 2 and 3). The high precipitation and high-flow events, which are characteristic of the Coeur d’Alene basin, have distributed mining wastes over many miles. The size and complexity of the basin combined with the highly variable nature of the mine wastes render site characterization a formidable task.
Systems Approach and the Conceptual Site Model
One way of characterizing the Coeur d’Alene basin for the purpose of remedial planning is to use a “systems approach” (see Box 4-1). This “system” is logically defined by watershed2 boundaries. Within the Coeur d’Alene system, relevant aspects are considered, including the geology, hydrology, ecologic communities, climate, human factors, and mining-related wastes. Under the systems approach, subwatershed boundaries are used for looking at smaller, more-manageable units while maintaining an awareness of interconnectedness between those units and the entire system.
EPA’s process for investigating a Superfund site calls for the creation of a “conceptual site model” (CSM) at the beginning of the RI. This model is intended to guide the way the RI is conducted and establishes a conceptual framework for the rest of the Superfund cleanup process. The CSM developed for the basin is largely based on geographic characteristics of the stream valleys and hydrologic characteristics of water bodies and is tantamount to looking at the overall Coeur d’Alene system in terms of more manageable subwatersheds. The basin was subdivided into five CSM units that correspond with Chapter 3’s description of the basin’s topography.3 The description of each CSM unit in the RI is accompanied by a complex “process model” diagram, characterizing the multifarious interactions that may take place in each unit. Figure 4-1 shows the process model for the Canyon Creek watershed.
One aspect of a systems approach only nominally considered in the development of these models is the amount of variability that exists in the basin—particularly with respect to the climatic and hydrologic systems. As evidenced by the large floods experienced in the basin and their tremendous impact on contaminant transport, these events are a critical element in the basin’s hydrologic system. The conceptual models, and therefore the definition of possible remedies, seemingly are based primarily on average conditions, and the committee believes that variations in the basin’s systems, particularly flood events, may have a significant impact on the effectiveness of the proposed remedies.
In addition, in carrying out assessments of the individual geographical components of the basin, the RI appears to have lost sight of the broader interactions within this complex system. Based on a systems approach, the RI should look at the watershed boundaries defining the basin system and then develop a flux-reservoir model of where each metal of importance
BOX 4-1 Systems Approach “In the context of water resources the essential function of a systems approach is to provide an organized framework that supports a balanced evaluation of all relevant issues (e.g., hydrologic, geomorphic, ecologic, social, economic) at appropriate scales of space and time. Within a systems framework, multiple stressors can be identified and quantified, multiple goals can be investigated, trade-offs among competing objectives can be evaluated, potential unintended consequences can be identified, and the true costs and benefits of a project can be examined in a context that incorporates the interest of all those with any substantial stake…. The merits of a systems approach are broadly endorsed … throughout the water resources community, and in several NRC reports (NRC 1999a,b, 2000, 2001)…. A systems framework supports a balanced consideration of all relevant aspects of water resources problems at all relevant time and space scales.” Source: NRC 2004. |
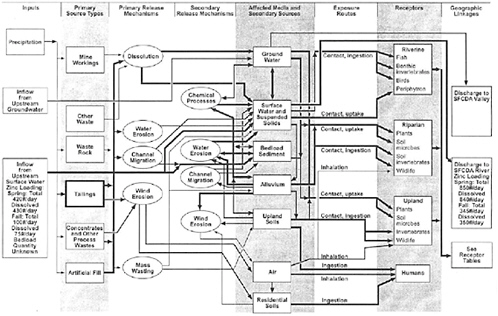
FIGURE 4-1 Process model for Canyon Creek Watershed (CSM Unit 1). …… low importance, ____ medium importance, high importance. SOURCE: URS Greiner, Inc. and CH2M Hill 2001b, p. 2-22.
resides and where that metal is transported at the established flux. The RI should consider the roles that geology, hydrology, geomorphology, geochemistry, forest management practices, infrastructure, etc. all play as components of the system. In fact, a similar approach was recommended in an EPA report (Hornig et al. 1988) that looked at the water quality monitoring in the Coeur d’Alene River basin:
A whole basin environmental management approach to the Coeur d’Alene system should also address the relative importance of habitat degradation and other factors (for example, nonpoint impacts from agricultural or forestry practices) in the prevention of full potential of aquatic resources. The dynamics of cadmium and lead in the ecosystem also needs to be further addressed, including the relative importance of the contribution of present South Fork loadings of these metals to the downstream sediments and biota.
EPA made preliminary steps toward looking at the Canyon Creek watershed using a systems approach. However, this approach appeared to be less in evidence in other parts of the basin, particularly regarding the box which is excised from consideration in the basin’s RI and subsequent documents. A systems approach would consider the contaminant sources and pathways within the box along with those stemming from upstream portions of the South Fork of the Coeur d’Alene River and also consider their potential to serve as contaminants in downstream areas.
Operable Unit Designation
Operable Units 1 and 2
As mentioned, OUs 1 and 2 are the populated and nonpopulated areas, respectively, of the 21-square-mile box. OU-3, the subject of this review, includes all the rest of the basin from the headwaters west into eastern Washington. In some cases, defining separate OUs may facilitate an earlier start on cleanup of a more-contaminated area. This was the situation for OU-1 and OU-2 because cleanup of these units began well before the RI for OU-3 was initiated. While this segmentation may have been appropriate at the time based on the severity of contamination in the box, it currently creates technical issues regarding implementation of remedies for protecting ecologic health downstream of the box.
These technical difficulties arise, for instance, in efforts to protect fish downstream of the box. In this stretch of the river, the major source of dissolved zinc comes from groundwater discharges to the river that occur within the box but apparently cannot be addressed in remedies considered
for OU-3.4 It is not clear whether there are cost-effective remedies for controlling these sources, but it makes no technical sense to ignore this possibility entirely. The manner in which the Superfund site was segmented has also created public perception problems. For example, privately-owned properties on different sides of the dividing line could have similar levels of contamination, but properties outside the box had to wait a decade before becoming part of the Superfund site and be considered for remediation.5
Operable Unit 3
EPA has substantial flexibility under the NCP in establishing what areas or actions will constitute an OU at a site.6 However, the guidance does state that “sites should generally be remediated in operable units when … phased analysis and response is necessary or appropriate given the size or complexity of the site, or to expedite the completion of total site cleanup.” Certainly, the Coeur d’Alene River basin is such a site though the entire basin (minus the box) was considered a single OU. The committee’s evaluation suggests that a different segmentation approach to OU-3 might have been preferable. There is a remarkable independence between protecting human health and protecting the environment. None of the remedies undertaken for human health protection will have any discernable impact on the protection of fish and wildlife (see Chapter 8). Similarly, EPA identifies only limited human health benefits that would result from the remedies being considered for protecting environmental resources (EPA 2002,
4 |
EPA states that they intend to integrate actions selected in the ROD with those implemented in the box (EPA 2002, p. 4-6). However, exactly what EPA intends to do is not yet clear. The agency has postponed implementing any efforts to cleanup groundwater seeping through the CIA until it sees how successful the cap on this facility will be in reducing groundwater contamination. The following is provided in the 5-year review for OU-2: “For groundwater, the cleanup levels specified in the ROD for site-wide groundwater were maximum contaminant levels (MCLs) and MCL goals for arsenic, copper, lead, mercury, PCBs [polychlorinated biphenyls], selenium, silver, zinc, and nitrate as identified under the Safe Drinking Water Act. The ROD further defined contingency measures to be implemented if these cleanup goals were not capable of being met” (EPA 2000, p. 5-2). |
5 |
Public perception problems also stem from the fact that the agency seems to have reversed its original position, which was to deal with the environmental problems outside of the box using programs other than Superfund (see Chapters 1 and 2 for further discussion). |
6 |
The NCP states that “Operable units may address geographical portions of a site, specific site problems, or initial phases of an action, or may consist of any set of actions performed over time or any actions that are concurrent but located in different parts of a site” (40 CFR § 300.5[2004]). |
Table 12.2-1). These remedies include limiting exposures associated with recreational activities at mine-waste sites or riverbanks.7
A more rational segmentation might have been to make one OU the protection of human health (or even several OUs based on subwatersheds of the basin, or addressing, for example, residential properties, public use areas, and other human health risks), and the second OU the protection of environmental resources (or perhaps several OUs based on the subwatersheds of the basin).8 This approach would have had some clear technical advantages in allowing the agency to analyze risks more systematically and in considering remedial alternatives more effectively, because of the more manageable size and differing characteristics of the smaller OUs.
In addition, such an approach probably would reduce the pall that so many residents believe will shadow the basin for decades to come, for the human health protection remedies in the basin will be completed relatively quickly. When this occurs, the basin could be declared to be cleaned up with respect to human health, although further work would be required to protect the environmental resources. To the extent that the designation of the basin as a Superfund site affects its economic prospects, such a distinction might well have reduced these negative effects.
It is probably too late to make such a change, but the agency might consider such an approach at other large sites where some of the cleanup activities will take long periods to complete.
SAMPLING AND ANALYSIS
Samples Collected
Some 7,000 samples had been collected in the Coeur d’Alene River basin between 1991 and 1999 by the Idaho Department of Environmental Quality, the U.S. Geological Survey (USGS), mining companies, and EPA under other regulatory programs (URS Greiner, Inc. and CH2M Hill 2001b,
p. 4-8). These historical samples, obtained from sediments, surface waters, groundwater, and soils, had been collected to support investigations with different objectives than those set forth for the RI. Nevertheless, a decision was made by the EPA to rely on data from these 7,000 historical samples already collected, although the quality assurance and quality control (QA/ QC) procedures varied among the various studies, and the results from the several data sets were generated from multiple methods of analysis. Because the levels of metal contamination from these studies were large in comparison to the levels considered problematic, the EPA was less concerned with the uncertainties associated with the QA/QC and analytical methodologies used. Based on review of the data from the 7,000 historical samples, EPA made the decision to collect additional samples and developed a Draft Technical Work Plan (URS Greiner, Inc. and CH2M Hill 1998a), which considered the EPA’s Data Quality Objective (DQO) process (EPA 1994). The Draft Technical Work Plan was used to develop field sampling plan addenda (FSPAs) (URS Greiner, Inc. and CH2M Hill 2001b, pp. 4-10 to 4-29), each with a specific purpose and scope, for collection of an additional 10,000 samples to characterize source areas. These samples were collected from sediments, sediment cores, adits, seeps, creek surface waters, soils, drinking water (wells, residential, and school/daycare), indoor dust, vacuum cleaner bags, lead-based paint, and groundwater. Two types of sampling were conducted: judgmental and probabilistic. Judgmental sampling (that is, nonprobabilistic) entailed sampling specific areas to confirm the existence of contamination. The committee did not assess EPA’s DQO process, Draft Technical Work Plan, FSPAs, or the methodology used by EPA to review and incorporate data from the 7,000 historical samples.
The 17,000 samples, collected over the large basin area, perhaps represent less than a dozen samples per square mile (although a much higher density of samples exists in the contaminated floodplain). The Bureau of Land Management identified approximately 1,080 mining-related source areas in the basin. Source areas were identified as either primary or secondary. Primary sources, mostly present in the upper basin (that is, the area characterized by high-gradient tributaries to the South Fork Coeur d’Alene River), include mine workings, waste rock, tailings, concentrates and other process wastes, and artificial fill. Secondary sources, principally located in the lower segments of upper basin tributaries, the middle basin (Wallace to Cataldo), and the lower basin (Cataldo to Harrison), include affected media (for example, groundwater, floodplain deposits, and bottom sediments) that may act as sources of metals to other media or receptors.
EPA points out that of the approximately 1,080 sources, samples were collected from about 160 (15%) with fewer than five samples collected from most of these source areas (URS Greiner, Inc. and CH2M Hill 2001b, p. 4-36). These areas range in size from less than an acre to hundreds of
acres and are listed in Appendix I of the RI. Major tailings, waste rock, and floodplain sources of metal contaminants were identified by EPA as to location and area. Sample locations and data collected were documented. Sources with an area greater than 5 acres were surface sampled; few samples were collected at a depth of greater than 1 foot. Not all sources were systematically characterized in terms of thickness. Greater effort was expended to document contamination in the floodplains of the Coeur d’Alene River. The USGS mapped, measured thickness and surface extent, and analyzed floodplain sediments in upper basin tributaries, the South Fork of the Coeur d’Alene River, and the lower basin (Box et al. 1999, 2001; Bookstrom et al. 2001, 2004; Box and Wallis 2002; Box et al. in press). It will be important to incorporate data from these analyses that was not considered in the RI in remedial planning within the basin.
In addition to collecting samples from only 15% of the sources identified by the Bureau of Land Management, the agency made no effort to characterize groundwater “source terms.”9 The committee learned from EPA’s written response to submitted questions that leachability data per se, which would characterize the source term, were not available and therefore were not used in the analyses and estimates of loading (see the section “Analyzing Sample Data” for a discussion of metal loading). Very simply, localized areas of high (or low) leachability were inferred from what are considered to be sources (such as nearby floodplain tailings) and measured increases in dissolved metal loadings in streams (EPA 2004 [June 23, 2004]).
Nonetheless, the committee believes that the large number of samples collected and analyzed provides information on contaminant locations and trends related to contaminant transport and fate in the basin, especially for surface water. Much new information has become available since the ROD was issued (EPA 2002), and EPA is commended by the committee for its cooperative, scientific relationships with sister agencies and others. The agency is urged to proceed with more-thorough identification of specific sources contributing dissolved or particulate metals to surface waters before proceeding with cleanup to ensure the location, magnitude, and disposition of contaminant sources and their contribution to the system.
TABLE 4-1 COPCs and Affected Media for the ERA
Chemical |
Ecologic COPC |
||
Soil |
Sediment |
Surface Water |
|
Antimony |
|
|
|
Arsenic |
* |
* |
|
Cadmium |
* |
* |
* |
Copper |
* |
* |
* |
Iron |
|
|
|
Lead |
* |
* |
* |
Manganese |
|
|
|
Mercury |
|
* |
|
Silver |
|
* |
|
Zinc |
* |
* |
* |
SOURCE: URS Greiner, Inc. and CH2M Hill 2001b, Table 5.1-1. |
Nature of Contamination
Chemicals of Potential Concern
Based on preliminary results of the ecologic risk assessment (ERA), ten chemicals of potential concern (COPCs)10 were identified by EPA for inclusion and evaluation in the RI. These initial COPCs were evaluated, and those that met the data evaluation requirements and screening against applicable risk-based screening criteria were incorporated. Applicable risk-based screening levels were compiled from available federal numeric criteria (for example, national ambient water-quality criteria), regional preliminary remediation goals, regional background studies, and other guidance documents. Table 4-1 lists these initial ten COPCs and affected media considered for the ERA. COPCs not carried forward in the ERA were antimony, iron, and manganese, because they did not meet the applicable risk-based screening criteria (URS Greiner, Inc. and CH2M Hill 2001b, p. 5-1). Groundwater data were screened against surface-water screening levels to evaluate the potential for impacts to surface water from groundwater discharge (URS Greiner, Inc. and CH2M Hill 2001b, p. 5-2).
The two chemicals of ecologic concern (COECs) receiving the most attention from EPA for the Coeur d’Alene River basin system are lead and
zinc. The environmental chemistry of these two metals is appreciably different. Lead is primarily present and transported in the basin as a particulate and is a major concern because waterfowl ingest lead-contaminated sediment (see Chapter 7) and children are exposed to lead through lead-contaminated soil or dust (see Chapters 5 and 6). Dissolved lead concentrations are low because lead is quite insoluble under the chemical conditions of the basin. Zinc is transported primarily in dissolved form (Beckwith et al. 1997, p. 6) and is a toxicant for fish and aquatic invertebrates (see Chapter 7), but zinc is also significantly transported in particulate form especially during floods (Beckwith 1996; Box et al. in press). Other COECs have been compared with total lead and dissolved zinc in the RI. EPA uses dissolved zinc concentrations as an indicator of the behavior of each dissolved chemical of concern and total lead concentrations as an indicator of the behavior of each total chemical of concern to avoid having to consider each chemical of concern separately (URS Greiner, Inc. and CH2M Hill 2001c, p. 4-11).
Of the dissolved COECs, zinc is the principal dissolved metal of concern, and EPA reports using zinc as an indicator metal for the following reasons (URS Greiner, Inc. and CH2M Hill 2001c, Section 4.2.1; URS Greiner, Inc. and CH2M Hill 2001d, p. 1-8):
-
Zinc is the most ubiquitous of the metals.
-
Zinc occurs at the highest measured concentrations and has the highest ratios of average measured concentration to ambient water-quality criteria or, equivalently, average measured load to total maximum daily-load loading capacities.
-
Zinc is relatively mobile compared with other metals.
-
Dissolved metals generally correlate with dissolved zinc.
In the South Fork of the Coeur d’Alene River, zinc accounts for about 96% of the dissolved heavy-metal load, and zinc is the main dissolved metal as the Coeur d’Alene River flows into Lake Coeur d’Alene at Harrison (Woods 2001). EPA discussed the correlation of zinc with other metals (URS Greiner, Inc. and CH2M Hill 2001c), and although cadmium appears to correlate well with dissolved zinc throughout the basin, other COEC metals (copper, mercury, silver, and arsenic) exhibit various degrees of correlation with dissolved zinc. The committee clarifies that arsenic and antimony behave similarly but these two elements should not be expected to correlate with either zinc or lead, because their chemistries are substantially different. Arsenic and antimony occur in water as oxyanions (with negative charges), whereas zinc and lead are positively charged cations. Furthermore, the aqueous mobilities of arsenic and antimony are affected by redox changes and depend on the redox conditions of the water, whereas
zinc and lead undergo no redox reactions. Aqueous arsenic and antimony are derived from the oxidative weathering of sulfide minerals, such as arsenopyrite (FeAsS), enargite (Cu3AsS4), and tetrahedrite [(Cu,Ag)10 (Fe,Zn)2(As,Sb)4S13], which are all found in some of the mineralized areas of the basin. Although it is reasonable to consider zinc as the principal dissolved metal of concern, care must be taken in correlating zinc with other metals.
Groundwater Considerations
Groundwater is the primary source of dissolved metals in the surface water of the basin. As stated by EPA (EPA 2004 [June 23, 2004]),
Except under very high-flow flood events, the majority of the zinc load, and particularly the dissolved zinc load, in the CDA [Coeur d’Alene] River at Harrison is contributed by groundwater…. Except for direct loading from adit discharges and storm water discharges from waste piles, zinc loading to streams is from affected groundwater in the floodplains.
The committee notes that investigations documenting aqueous concentrations of dissolved metals within the basin focused primarily on monitoring surface-water concentrations. A more-limited campaign to sample groundwater was undertaken that included establishing monitoring wells in Canyon Creek (Houck and Mink 1994; MFG 1995, 1998; Ridolfi 1998; Barton 2000; URS Greiner, Inc. and CH2M Hill 2001c). In the middle basin between Wallace and Pinehurst, other studies (Dames and Moore 1991; Barton 2000, 2002; CH2M Hill 2004a,b) evaluated the complex relationship between surface water and the shallow groundwater aquifer that can lead to losses of dissolved metals to the aquifer in some reaches and dissolved metal gains from others.
The committee found there to be limited information on groundwater contamination in the main stem and lower Coeur d’Alene River (Spruill 1993; Balistrieri et al. 2000, 2002; URS Greiner, Inc. and CH2M Hill 2001e, p. 4-8). Groundwater-contaminant contribution is suspected where it discharges to the river from contaminated bank and floodplain sediments, and groundwater may be a continuing source of contaminants in the lateral lakes area. Little information is available on metal transport in groundwater around Lake Coeur d’Alene and along the upper Spokane River (URS Greiner, Inc. and CH2M Hill 2001e, p. 4-8).
Because groundwater is the primary source of dissolved zinc to the system, the committee believes that developing a more-thorough understanding of the metal concentrations, dynamics, and specific source areas and media is necessary. Understanding this dynamic undeniably will require
additional characterization. The committee acknowledges that groundwater characterization studies are expensive and draw from limited funds potentially used for remediation projects (generally source removals), which attempt to directly reduce the flux of water through contaminated surficial aquifers. However, it is necessary to characterize source areas and media contributing dissolved metals to groundwater (which is later discharged to surface waters) to accurately define remedial strategies, particularly source removals, intended to curtail zinc contributions to surface water. Tracer injections and synoptic sampling can be combined to understand and quantify metal loading to stream reaches impacted by mining, an approach developed in part by the USGS Toxic Substances Hydrology Program (Kimball 1997; Kimball et al. 2002). These studies simultaneously sample metals and a tracer (for example, lithium or bromide injected upstream) in surface water to permit high-resolution determinations of metal loading along a stream. These cost-effective techniques can be used to define source areas and metal contributions from groundwater, guide future cleanup efforts, and ascertain the effectiveness of remedial actions (Kimball 1997). This approach could be used as part of a site-characterization strategy in the Coeur d’Alene River basin.
Analyzing Sample Data
EPA relies on mass loading to describe the amounts and types of contaminant constituents in surface waters and identify sources, particularly secondary sources, of contamination. Mass loading is the mass of a constituent passing a given point per unit time; in the RI, mass loading is expressed in pounds per day. To measure mass loading, stream gauging is conducted to determine stream discharge in cubic feet per second. Chemical analyses of water samples are carried out, and the constituent concentrations are expressed in micrograms per liter (μg/L). Mass loading is the product of stream discharge and constituent concentration, converted to pounds per day.11
Mass loading is evaluated by two different methods, although these methods are not mutually exclusive. One method calculates “point estimates of mass loading” from discrete discharge and concentration data (URS Greiner, Inc. and CH2M Hill 2001b, pp. 5-6, 5-7). That is, mass loading is determined at a single USGS gauging station at one point in time. The second method, “estimated average mass loading,” uses a combined data set and a probabilistic model (URS Greiner, Inc. and CH2M Hill
2001b, p. 5-24) (1) to predict metal concentrations in the stream, (2) to predict metal loading in the stream (how much metal is flowing in the stream), and (3) to quantify the uncertainty associated with the predictions. Estimated average mass loadings are derived by taking all the historical data from all points in time at a USGS gauging station, plotting it, and obtaining from the plotted data a measure of central tendency—the “expected value.” Estimated average mass loading data in the RI refer to current conditions in the basin. These data are presented in parts 2-6, section 5, of the RI (URS Greiner, Inc. and CH2M Hill 2001a) and are used to characterize dissolved metals, total lead, and sediment for the entire basin excluding Lake Coeur d’Alene.12
The committee found that the analyses provided for zinc and lead are useful for understanding the contributions of various tributaries and large-scale geographic areas to metal loadings in the basin by providing a central estimate at each gauging location. This “estimated average mass loading,” with appropriate application of standardization methods to accommodate stream flows, as a methodology to describe dissolved constituents and sediment in surface waters provides an overall depiction of dissolved and particulate contaminants moving through the river system over time. Further, the committee found this method adequate to demonstrate that surface-water concentrations of dissolved zinc are substantially elevated compared to water-quality criteria and to show that large amounts of metals are transported through the system. It is a method for evaluating the total input of metal to the system, but the committee emphasizes that the method does not provide the location of sources or underscore the high concentrations of toxic metals that may occur in the system at any one time. The committee cautions that averaged data can be misleading in several ways:
-
The highest concentrations of dissolved metals, especially zinc, occur during low-flow events. Therefore, low-flow events have the greatest impact on the aquatic ecosystem. This fact could render inadequate certain remedial decisions made with averaged mass loading data.
-
The highest suspended sediment loads, which can contain particulate lead and zinc, occur during high-flow events, when the erosive ability of the river is greatest. High mass loadings of lead-containing sediment are transported during high-flow events to wetlands, marshes, and the lateral lakes inhabited by waterfowl. Use of averaged sediment mass loadings to arrive at remedial alternatives may result in unanticipated recontamination during
12 |
In the FS, the probabilistic model is used to make quantitative estimates of the potential remedial performance associated with each remedial alternative selected (URS Greiner, Inc. and CH2M Hill 2001b, p. 5-24). This use of the probabilistic model and mass loading is discussed in Chapter 8. |
-
high-flow events. This issue is discussed in greater detail in Chapter 8 of this report.
DETERMINING BACKGROUND CONCENTRATIONS
For the purpose of identifying areas within the Coeur d’Alene and Spokane River basins that are contaminated by mining wastes, EPA (URS Greiner, Inc. and CH2M Hill 2001f, p. 1-1) defines “background” as follows:
For the purpose of the RI/FS, background is considered to be the concentration of a substance in environmental media that are not contaminated by the sources being assessed. Background concentrations are due to naturally occurring substances and other anthropogenic metal sources unrelated to mining (for example, leaded gasoline emissions from cars).
The committee considers this definition of background concentrations to be vague and open to interpretation but focused on the derivation of the values that were ultimately used. Background concentrations are determined primarily for two purposes: first, to estimate the extent of contamination (that is, where contamination levels in various media exceed background levels); and, second, to assist in the selection of remedial goals or target cleanup levels when used in conjunction with risk-based values determined through risk assessments. The process for establishing these background concentrations is described in a technical memorandum (URS Greiner, Inc. and CH2M Hill 2001f). Section 104(3)(a) of Comprehensive Environmental Response, Compensation, and Liability Act (CERCLA) provides the regulatory basis for determining background concentrations of metals (and other naturally occurring hazardous substances at CERCLA sites) and states in part that
The President shall not provide for a removal or remediation action under this section in response to a release or threat of a release of a naturally occurring substance in its unaltered form, or altered solely through natural occurring processes and phenomena, from a location where it is naturally found.
CERCLA uses various strategies to estimate baseline metal levels at Superfund sites. CERCLA guidance for site-specific evaluation of baseline levels of metals in soils is not applicable to nonsoil media (for example, surface water), which tend to be more dynamic and are more likely to be influenced by upstream and distal sources. Assessment of background levels for nonsoil environmental media requires more complex spatial and temporal sampling strategies, analysis of releases and transport, and different ways of combining and analyzing data.
For the RI (URS Greiner, Inc. and CH2M Hill 2001a), EPA estimated background concentrations for ten COPCs (antimony, arsenic, cadmium, copper, iron, lead, manganese, mercury, silver, and zinc) for three environmental media (soils, sediments, and surface water) affected by mining activities (URS Greiner, Inc. and CH2M Hill 2001f, Table ES-1). Background concentrations were not determined for groundwater.
In view of the large geographic area and geologic diversity of the basin, EPA used a range of concentrations rather than a single-point estimate in the characterization of background for this site (URS Greiner, Inc. and CH2M Hill 2001f, p. 1-3). Because of the differing mineralization and erosion/deposition characteristics of the basin, background concentrations for the COPCs were developed separately for geographic areas: the upper basin (high-gradient tributaries to the South Fork), the middle basin (Wallace to Cataldo), the lower basin (Cataldo to Harrison), and the Spokane River Basin from the city of Coeur d’Alene to Lake Roosevelt on the Columbia River. EPA included Lake Coeur d’Alene in the lower basin, justifying this because the lake is part of the Coeur d’Alene River complex that supplies metal contaminants to downstream ecosystems. This section assesses the derivation of upper limits of background concentrations for COPCs reported by EPA (URS Greiner, Inc. and CH2M Hill 2001f) and related issues.
Background Concentrations of Metals in Coeur d’Alene and Spokane River Basin Soils and Sediments
EPA reviewed existing literature and concluded sufficient information was available to define background-concentration ranges for the COPCs in the upper and middle basin soils. However, the agency concluded that existing studies were not adequate to establish background ranges for all ten metals in sediments of the upper, middle, and lower Coeur d’Alene River basin and the Spokane River. The background ranges and summary statistics for sediments in these areas were derived by EPA from upper and lower basin sediment data collected for the RI/FS and Spokane River Basin soil data collected by the Washington State Department of Ecology (EPA 2004 [June 14, 2004]).
Background concentrations of the 10 COPCs in soils in the upper and middle basin were based on the data reported by Gott and Cathrall (1980) from 8,700 soil samples collected from approximately 300 square miles of the Coeur d’Alene mining district. From this database, the 90th percentile metal concentration was used as the background soil concentration for the ten COPCs (URS Greiner, Inc. and CH2M Hill 2001f).
Background-concentration ranges of COPCs for upper and middle basin sediments were estimated based on samples from monitoring well bore-
holes located largely in Canyon Creek but also included samples from Ninemile Creek and Pine Creek (URS Greiner, Inc. and CH2M Hill 2001f). EPA was aware that these upper basin tributaries are the most highly mineralized drainages and, as such, that samples from these areas may overestimate background metals concentrations in sediments for the entire upper and middle basin. Metal concentrations in sediments at various depths in the boreholes were assembled into a single database for analysis. The committee believes this database (URS Greiner, Inc. and CH2M Hill 2001f, Table C-1) is limited by the wide sampling interval in the boreholes, small number of subsurface samples, and, likely, the varying depth to background at different locations.13 The background concentrations of metals in sediments in the upper basin were based on 12-30 sample values, depending on the COPC being considered. According to EPA, plots of sediment COPC concentrations versus depth in the core material showed a discontinuity indicative of the onset of mining impacts in the metal profile. Further, the transition in the COPC profile was confirmed by a combination of visual and statistical techniques as described in the technical memorandum (URS Greiner, Inc. and CH2M Hill 2001f, p. 3-1). Essentially, the analysis differentiates between two populations of samples, background and contaminated, and describes the background sediments as those below a certain depth. For some metals, this was 10 feet; for others it was 15 feet (URS Greiner, Inc. and CH2M Hill 2001f, Table 4-1). EPA found that the background concentrations of the COPCs generally were much higher (90th percentile comparison) in the upper/middle basin soils than in the sediments (URS Greiner, Inc. and CH2M Hill 2001f).
Background concentrations of COPCs in lower basin sediments were derived from core samples (URS Greiner, Inc. and CH2M Hill 1998b) collected for the RI/FS (URS Greiner, Inc. and CH2M Hill 2001a). Unlike the analysis for the upper and middle basin, EPA did not use concentration versus depth profiles to identify the threshold depth for background concentrations of COPCs in the lower basin. The reason given is that sediment thicknesses in this region are highly variable. The range in background concentration for each COPC was estimated by using, in the committee’s opinion, a complicated and subjective ten-step process developed by EPA (URS Greiner, Inc. and CH2M Hill 2001f). Although EPA considers this approach a reliable means of estimating background concentrations, the committee believes that the subjective nature of the agency’s method poten-
tially can produce results outside the range that objective methods would provide (see Appendix B for a review of this method). Nevertheless, the lower basin background concentrations derived from the ten-step method appear consistent with previous studies, and, based on the committee’s review of data from various coring studies, the background concentrations for metals with limited mobility, such as lead, appear reasonable. For example, in another study, the USGS determined the background concentrations for lead in lower basin sediments as 26 mg/kg (median concentration) and 31 ± 19 mg/kg (mean ± standard deviation) (Bookstrom et al. 2004) compared with 47.3 mg/kg (90th percentile) resulting from this analysis (EPA 2002, Table 7.2-7).
However, the committee has concerns regarding the data set and sampling methodology of the study used to determine background concentrations. In this analysis, data from multiple cores were assembled into a single database from which background concentrations were mathematically derived. However, large numbers of these cores did not penetrate through the lead-enriched sediments to uncontaminated background sediments. In addition, samples taken along the length of many of the cores were widely spaced.14 It is possible that the limitations of this data set made it necessary to compile all the data and mathematically determine a background concentration. The background technical memo (URS Greiner, Inc. and CH2M Hill 2001f) does not comment on this issue. Bookstrom et al. (2001) noted that sample intervals crossing the contact between the lead-rich sediment and the underlying lead-poor sediment will dilute and underestimate the lead content of the lead-rich segment. The use of wide sampling intervals is particularly problematic in parts of the lower basin, where the lead-rich sediments are less than 1 foot thick and EPA’s sampling interval ranged up to several feet.
Coring studies are useful techniques capable of sampling historic sediments deposited before a particular event in time; in this case, the onset of mining. To define background concentrations, it is more reliable to sample cores with high vertical resolution (many samples along the length of the core) and to such a depth that the onset of premining background sediments can be defined instead of relying on mathematic and graphical techniques. Independent measures, such as time-stratigraphic markers and radioactive isotopes (for example, 137Cs), should be used to determine that sediments originate from premining times.
EPA included Lake Coeur d’Alene with the lower basin for background estimation. Lake Coeur d’Alene receives sediments from nonmineralized drainages and EPA stated that “its inclusion with the lower Coeur d’Alene River is expected to result in the selection of background COPC concentration ranges that may be biased high.” (URS Greiner, Inc. and CH2M Hill 2001f, p. 1-3).
However, in a coring study on Lake Coeur d’Alene, Horowitz et al. (1995) estimated the background concentrations of lead in sediment to be 33 mg/kg. This average is partially derived from cores taken in the St. Joe arm of Lake Coeur d’Alene. Regardless, all these concentrations are quite similar, especially in contrast to the high concentrations of metals detected in contaminated sediments, which are orders of magnitude higher.
EPA derived the background concentrations for the COPCs in the sediments of the Spokane River basin with data for 27 soil samples, collected to depths of up to 3 feet (San Juan 1994). EPA believes that sampling was designed to exclude the impacts of mining. Summary statistics for the background data were derived with the Model Toxics Control Act background computer module and were plotted as cumulative frequency distributions (CFDs) to calculate additional summary statistics as necessary (URS Greiner, Inc. and CH2M Hill 2001f). EPA recognizes that the values adopted were biased low, because the background samples were taken from areas that historically were not exposed to the Coeur d’Alene drainage (URS Greiner, Inc. and CH2M Hill 2001f, p. 5-2).
Background Concentrations of Metal in Coeur d’Alene River Basin Surface Water
EPA used existing surface-water data collected by the USGS, EPA, and Idaho Department of Environmental Quality to estimate the background concentrations for the COPCs. For this analysis, the entire basin was divided into three subareas: the tributaries to the South Fork (upper basin), the Page-Galena Mineral Belt area (corresponding to the middle basin), and the Pine Creek drainage basin. According to EPA, the background sampling locations were from unaffected upstream reaches in watersheds affected by mining and watersheds known to have relatively minor mining impacts. EPA asserts that these locations were chosen on the basis of their similarities to the contaminated areas in terms of watershed characteristics including geology, hydrology, and extent of mineralization as described in Stratus (2000). Background concentrations for surface waters in each of the three areas were determined and then pooled to get estimates for the entire upper and middle basin. According to EPA, consideration of the effects of surface expression of ore veins and the surrounding metalliferous rocks suggested that the background concentrations are biased high when applied to the Coeur d’Alene Basin as a whole (URS Greiner, Inc. and CH2M Hill 2001f,
p. 4-6). The relation of mineralogical features to each of the sampling locations was not considered by the committee. EPA accepts that the statistics reported for background concentrations of the COPCs were influenced by the large number of water samples with metal concentrations below the analytical detection limits. EPA’s approach to these samples was to use one-half of the detection limit to represent the value for the metal in the sample (URS Greiner, Inc. and CH2M Hill 2001f, p. 5-3).
Background Metal Concentrations for Groundwater
Background metal concentrations were not determined for groundwater. The technical memorandum establishing background concentrations (URS Greiner, Inc. and CH2M Hill 2001f) states that
Affected or potentially affected media types include soil, sediment, surface water, and groundwater. Of these media types, soils, sediments, and surface water are of primary concern because of the potential for exposure to human and ecologic receptors.
Are the Background Determinations Adequate for Their Use?
The committee observed that the Superfund decision documents developed for the Coeur d’Alene River basin frequently use background concentrations as a comparative measure to assess the extent of contamination in various environmental media. The ROD (EPA 2002) has numerous such uses. With the exception of the Spokane River, background determinations were not used appreciably for the second purpose, which was to assist in selecting remedial goals or target cleanup levels when used in conjunction with risk-based values. Yard remediation in the box and basin is triggered at levels well above background. The same is true for remedies intended to protect ecologic receptors. For example, the background lead concentration of soil and sediment in the lower basin is estimated to be 47.3 mg/kg (EPA 2002, p. 12-39), whereas the lead concentrations in affected areas at this location are 3,500-4,000 mg/kg, and the site-specific benchmark cleanup criterion is 530 mg/kg.
EPA addresses the background determinations in a manner consistent with the agency’s established guidelines for assessing background concentration in soils and sediments at Superfund sites. The agency is commended for attempting to determine background rather than simply using national or regional numbers. For water, soils, and sediments in the tributaries of the South Fork of the Coeur d’Alene River (upper basin) and sediments in the Spokane River basin, the committee concludes that the background determinations are reasonable but limited by the issues presented in this section.
CHEMICAL SPECIATION AND TRANSPORT OF METALS
The mobilization of metals from sources; the movement of metals through environmental media (soil, sediment, and water); the changes that metals undergo in response to interactions with air, water, soil, sediment, and rock; and the transformation of metals by microorganisms are collectively referred to as “chemical speciation and transport.” In the Coeur d’Alene system, metals are transported in both dissolved and particulate form. Many of the metals defined as COPCs that are present in the tailings, waste rock, water, and other materials and discharged to the waters of the Coeur d’Alene system undergo chemical and microbiological changes as they are transported downstream and encounter various environmental conditions (URS Greiner, Inc. and CH2M Hill 2001a).
The chemical speciation and transport of metals are not only central to understanding the bioavailability and toxicity of metals to receptors but are important in selecting remedies that mitigate risk.
This segment of the report summarizes and evaluates EPA’s findings and conclusions, reported in the RI (URS Greiner, Inc. and CH2M Hill 2001a), on chemical speciation and transport of metals in the Coeur d’Alene River system, Lake Coeur d’Alene, and the Spokane River. This discussion focuses on EPA’s studies specifically related to understanding the chemistry and movement of the metals in the Coeur d’Alene system and summarizes information on sediment transport.
EPA’s Approach to Chemical Speciation and Transport Evaluation
A CSM, described earlier in this chapter, was provided in the RI to convey in abstract the sources of contamination, mechanisms of contaminant release, pathways of transport, and ways in which humans and ecologic receptors are exposed (URS Greiner, Inc. and CH2M Hill 2001b, pp. 2-1 to 2-19). The CSM developed by EPA for Canyon Creek is shown in Figure 4-1. Geochemical and hydrological conditions and mechanisms that EPA said (URS Greiner, Inc. and CH2M Hill 2001b, pp. 5-16 to 5-23) were considered in chemical speciation and transport of metals were flow events, pH, water chemistry, effect of iron concentration on metal concentrations, adsorption/dissolution/precipitation phenomena, amounts and types of atmospheric precipitation, erosion, and sediment movement.
Chemical Speciation
In response to the committee’s request for information on speciation and bioavailability in basin soils and sediments, EPA indicated (EPA 2004 [June 14, 2004]) that these issues were addressed in the responsiveness
summary of the ROD (EPA 2002, Part 3). This section of the ROD comments on the presumed speciation of the sediments but contains no indication that speciation was determined:
Prior to 1968, large masses of mine-related releases were discharged to local streams or floodplain locations in predominantly lead sulfide form. However, oxidized ores were also likely released because milling and extraction practices were primarily designed to capture galena from sulfide ore. Oxidized lead minerals present in the original ores also were likely discharged to tributaries of the Coeur d’Alene River…. During movement and weathering, the lead in mill tailings was subject to physical and chemical transformation through abrasion, pH changes, and exposure to the atmosphere and aerobic hydrologic environments. These conditions promoted decreased particle size and increased surface area, and enhanced oxidation and the transition from lead sulfide to oxidized species.
That section of the ROD (EPA 2002) also addresses soils and states “It is unlikely that all smelter-related soil and dust lead is in an oxide form and equally unlikely that the soil and dust particles ingested by children, that originated as mining releases, are purely a sulfide form,” and that the conclusion was consistent with results from other regions. Again, it is not apparent to the committee that speciation work was conducted.15 The importance of speciation to bioavailability and toxicologic considerations is considered for humans in Chapter 5 and 6 of this report and for ecologic receptors in Chapter 7. The need for this type of information has been long understood; in 1988, EPA concluded the following:
Research efforts should be encouraged that elucidate how the specific physical, chemical and biological characteristics of the Coeur d’Alene River and Lake system may affect the availability and toxicity of Silver Valley metal pollutants to different components of the ecosystem. (Hornig et al. 1988)
Sediment Transport
Most of the sediment transport data presented in the RI (URS Greiner, Inc. and CH2M Hill 2001a) were for water year 1999. Although the spring runoff for water year 1999 was higher than normal, the committee notes that there was no significant flood event—a phenomenon that significantly
affects sediment transport. As a consequence, the committee believes that information in the RI likely provides an incomplete picture of sediment transport and metal mobility associated with sediment transport in the Coeur d’Alene system. Further, the committee notes that the geographic extent of various stage floods (10 year, 100 year, etc.) is not defined (EPA 2004 [June 23, 2004]), although understanding the flood regimes is essential in characterizing the system and especially in developing durable remedial strategies. Since the RI was issued in 2001, the USGS has provided a more comprehensive understanding of sediment transport in the Coeur d’Alene system (Clark 2003; Bookstrom et al. 2004; Box et al. in press). EPA is urged by the committee to consider this information in subsequent steps of the CERCLA process.
For the RI (URS Greiner, Inc. and CH2M Hill 2001a), suspended sediment and bedload samples were not analyzed for total metals. Rather, mass loading of metals in sediments was estimated from the total metal concentration of unfiltered water and the dissolved metal content of filtered surface-water samples (URS Greiner, Inc. and CH2M Hill 2001c, p. 5-33; URS Greiner, Inc. and CH2M Hill 2001g, p. 5-13). The committee believes that this methodology would be expected to exclude the metal load associated with bedload sediments, which are those particles transported along the stream bed by rolling or sliding. The amount of bedload material would be expected to be higher in high-gradient streams, such as those in the upper basin, as opposed to more sluggish streams. Also, as for suspended sediment load, bedload would be expected to be greater in high-flow events than at low flow. The bedload may contain, for example, highly enriched jig tailings, coarse particles with high surface areas, or some high-density minerals (for example, galena and cerrusite) that would tend to concentrate in the bedload. Consequently, it is unclear whether measurements made on suspended sediments accurately reflect sediment-associated metal transport even for the 1999 water year evaluated.
Surface Waters
Given the large variations in flow and metal content, EPA decided that, rather than using a mechanistic or deterministic model, transport of metals in surface waters through the system could be dealt with by using a probabilistic model. As described above, the probabilistic model is a mathematical model based on monitoring data collected for zinc, lead, and cadmium in surface waters at various sampling locations. Some tributaries or stream reaches did not have sufficient data to use the probabilistic analysis (sampling locations required a minimum of ten data points). For example, Big Creek, a tributary with historical mining activities that enters the South Fork just upstream of the box, had two data points for lead and zinc from
which the loading was determined. In this case, the RI presents these two points as the lowest and highest loadings and concludes that the limited data set shows “small but significant contributions of metals from Big Creek to the South Fork” (URS Greiner Inc and CH2M Hill 2001h, p. 5-2). Other sampling locations have substantially more data, and the probabilistic model is used to determine an “expected” concentration and load. For example, Canyon Creek and Ninemile Creek were well characterized with multiple samples associated with a range of flows along the length of the tributaries. The South Fork and main stem of the Coeur d’Alene River had extensive surface-water sampling. Dissolved zinc concentrations and loading at the station near the mouth of the Coeur d’Alene River and Harrison was estimated from approximately 100 surface-water samples16 from a wide range of flows.
EPA used the probabilistic model to predict metal concentrations and metal loadings in streams and quantify the uncertainty associated with these predictions. As discussed earlier in this chapter, the committee emphasizes that this model does not incorporate geochemical mechanisms describing chemical speciation of metals (URS Greiner, Inc. and CH2M Hill 2001b, pp. 5-24 to 5-32). The probabilistic model also does not make a distinction among metals associated with suspended load, bedload, and dissolved load, all of which may transport metals differently in the stream. The ability of the model to predict postremediation changes is addressed in Chapter 8 of this report.
Chemical Speciation and Sediment Transport in the Upper Basin (CSM Unit 1)
Chemical Speciation
The RI reported on water samples from mine adits, seeps, and surface waters in the upper basin (URS Greiner, Inc. and CH2M Hill 2001a). Data generally included temperature, pH, conductivity, dissolved oxygen, alkalinity, flow, and total acid-soluble and dissolved major and trace ion concentrations (Balistrieri et al. 1998). These data are important for understanding the sources of dissolved metals and provide some information to
16 |
It is unclear to the committee how many surface-water samples were actually considered in this analysis. The RI states that 102 samples were collected and analyzed in this reach, yet data for 100 samples are presented for dissolved zinc in surface water at this location (URS Greiner and CH2M Hill 2001i, attachment 2, data summary tables). Data presented for dissolved zinc at this location (LC-60) for the probabilistic analysis show a summary statistic of N (number of samples) = 91, but only 38 data points are presented (URS and CH2M Hill 2001a, Appendix C). |
ascertain chemical speciation. In Canyon Creek and Ninemile Creek, pH measurements of surface water varied from slightly acidic for some adits and seeps to slightly alkaline for in-stream measurements. Metals are anticipated to be mobilized from the minerals by the slightly acidic conditions and oxidizing environment; these processes are governed by the mineralogy of the area (for greater detail see Balistrieri et al. 1999).
A diffuse-layer model (Dzombak 1986) was used to evaluate the adsorption of dissolved metals (cadmium, lead, and zinc) onto ferric oxyhydroxides17 (typically colloidal particles) in Canyon Creek surface waters. Results indicated minimal adsorption of zinc and cadmium at low flows suggesting that these metals are largely transported in the dissolved phase. Lead, on the other hand, is quite insoluble under these chemical conditions and is transported as a particulate or adsorbed onto ferric oxyhydroxides at high and low flows (URS Greiner, Inc. and CH2M Hill 2001c, p. 5-6). EPA used total and dissolved concentrations for each metal to evaluate the prediction of this model. However, the committee notes that these measurements are not capable of describing actual associations between the metals and iron oxyhydroxides.
EPA used an equilibrium speciation model (MINTEQA2) to estimate the precipitation and dissolution of metals in Canyon Creek and Ninemile Creek surface waters. The results of this model suggested that generally, cadmium, lead, and zinc are undersaturated in solution and not expected to precipitate (URS Greiner, Inc. and CH2M Hill 2001c, p. 5-7; URS Greiner, Inc. and CH2M Hill 2001g, p. 5-11).
Groundwater chemistry determinations in the upper basin tributaries consisted of measuring pH, salinity and specific conductance, oxidation-reduction (redox) potential, turbidity and sulfur species (namely, sulfide and sulfate). Monitoring wells in Ninemile Creek indicated freshwater conditions, near-neutral pH, low turbidity, oxidizing conditions, and sulfate concentrations ranging from 19,000 to 488,000 μg/L (URS Greiner, Inc. and CH2M Hill 2001g, p. 2-11). Similar results were reported for groundwater in Canyon Creek (URS Greiner, Inc. and CH2M Hill 2001c, p. 2-13 and 2-14), although slightly acidic (pH 4.5-6.5) groundwater was noted in the Woodland Park area. Such data provide some information from which inferences about metal chemistry and speciation can be drawn.
As discussed above, areas of tailings, waste rock, other process wastes, artificial fill, alluvium, and sediment generally greater than 5 acres were surface sampled, with few samples greater than 1 foot deep collected. Samples were analyzed for metals, but limited (if any) metal-speciation studies (for example, mineralogy) were performed on the samples collected. Surface samples are generally more oxidized, which can increase the mobil-
ity of the metal, and therefore surface sampling does not provide a complete picture of metal locations, concentrations, speciation, or potential mobility throughout the entire source.
Sediment Transport
The USGS measured suspended and bedload sediment transport and stream discharge data for the water year 1999 at four gauging stations in the upper basin (Clark and Woods 2001). Cumulative transport curves were indirectly derived for the RI from the USGS transport curves (Clark and Woods 2001). These derived curves, presented in the RI (for example, see URS Greiner Inc. and CH2M Hill 2001c, Fig. 3.2-1) were developed from instantaneous measurements of discharge and sediment and, as such, were rating curves. EPA applied these rating curves to mean daily discharge to obtain daily sediment transport and the resultant cumulative curves. Annual loads for the upper basin tributaries appear to be derived from the cumulative transport curves. These annual loads and cumulative loads normalized to drainage area are reported in the RI (for example, see discussion for Canyon Creek in URS Greiner Inc. and CH2M Hill 2001c, pp. 3-2 to 3-4) and are tabulated in Table 4-2.
The sediment transport data for Ninemile Creek are unclear. Different values of annual sediment transport loads are reported in the RI for water
TABLE 4-2 Water Year 1999 Sediment Transport Loads for Upper Basin Watersheds
Watershed |
Sediment Transported in Water Year 1999 |
|
Tons |
Tons/Square Mile |
|
Canyon Creek |
1,440 |
62 |
Beaver Creek |
No data available |
No data available |
Big Creek |
1,400 (estimated from Canyon and Ninemile Creeks) |
No data available (46, estimated from watershed area) |
Moon Creek |
No data available |
No data available |
Ninemile Creek |
397, 400, 500, and 1,350 (See text for explanation) |
34 |
Prichard Creek |
No data available |
No data available |
Upper South Fork, Coeur d’Alene River |
2,400 (estimated from Canyon and Ninemile Creeks) |
48 (estimated from Canyon and Ninemile Creeks) |
Pine Creek |
2,900 |
37 |
North Fork, Coeur d’Alene River |
25,400 |
28 |
SOURCE: URS Greiner, Inc. and CH2M Hill 2001a. |
year 1999; values stated are 500 tons (URS Greiner, Inc. and CH2M Hill 2001g, p. 3-1), 400 tons or 34 tons per square mile (URS Greiner, Inc. and CH2M Hill 2001g, p. 3-13), 397 tons (URS Greiner, Inc. and CH2M Hill 2001e, p. 4-15), and 1,350 tons (URS Greiner, Inc. and CH2M Hill 2001g, p. 5-11). The 400-tons/year and 397-tons/year numbers appear to come from adding the suspended sediment and bedload values in the two cumulative transport curves (URS Greiner, Inc. and CH2M Hill 2001g, Figs. 3.2-4 and 3.2-5). The 500-tons/year estimate may have been derived by relating 1999 discharge (18.7 cubic feet per second) to the USGS rating curves; that method would have yielded daily values that had to be multiplied by 365, resulting in overestimation. The 1,350-tons/year value appears to be an error (P.F. Woods, USGS, personal comm., December 20, 2004).
No sediment transport data are available for the Upper South Fork of the Coeur d’Alene River and Big Creek because no gauging stations were located on these segments. EPA estimated annual sediment transport loads for these watersheds based on 1 year of sediment transport gauging data available for Canyon Creek and Ninemile Creek drainages (URS Greiner, Inc. and CH2M Hill 2001j, p. 3-2). Given the discrepancies of sediment load in Ninemile Creek for water year 1999, it is not clear to the committee which value EPA used to estimate sediment transport in the Upper South Fork of the Coeur d’Alene River and Big Creek. However, adequate estimates for these two tributaries probably could be made with the cumulative load normalized to drainage area for Ninemile Creek (34 tons per square mile).
The RI identified likely sources of sediment mobilization in various segments of each tributary based on reconnaissance with aerial photographs and topographic maps. It is not evident whether this was followed up by drainage walk-through evaluations. In any event, these eroding reaches would be potential candidates for bank, hillside, and/or channel stabilization to mitigate erosion and sediment transport. Examination of some historical records indicated that sediment transport in some tributaries was less in water year 1999 than in some previous years. EPA attributed this to remedial actions that have been undertaken in some watersheds (URS Greiner, Inc. and CH2M Hill 2001g, p. 3-1). However, another reason may be that in 1999 there was no notable flood event, which may have been responsible for greater sediment transport in some previous years when records were available. Characterization of sediment transport is provided by limited but useful monitoring data. While only one water year is focused on, the analyses provide useful information on sediment transport from watersheds, particularly in those watersheds where sediment data was actually collected (compared to those where sediment transport was only modeled). Analysis of historical aerial photographs to evaluate stream channel dynamics and sources of sediments is
also a reasonable approach to generate information on sediment transport but cannot replace on-site evaluations for determining contributing sources.
Chemical Speciation and Sediment Transport in the Middle Basin (CSM Unit 2)
Chemical Speciation
Information on groundwater chemistry in the middle basin was based primarily on samples from monitoring wells in the box. Some historical data were used as well as data from more recent quarterly sampling of wells. Data presented for some groundwater samples included concentrations of dissolved metals, temperature, pH, conductivity, and major ions. In some cases, sufficient data were collected to evaluate chemical speciation (for example, URS Greiner, Inc. and CH2M Hill 2001k, Table 2.2-5). However, chemical speciation information for groundwater (or other media) was not reported in the RI.
Sediment Transport
Sediment transport in the middle basin (Wallace to Pinehurst) along the South Fork of the Coeur d’Alene River was measured at two USGS gauging stations, Silverton and Pinehurst, for water year 1999. Approximately 7,200 tons of sediment were transported past the Silverton gauge station and 22,000 tons at Pinehurst (URS Greiner, Inc. and CH2M Hill 2001k, p. 5-11). Suspended sediments and bedload samples were not analyzed for total metals, so mass loadings of metals were estimated from total and dissolved surface-water data (URS Greiner, Inc. and CH2M Hill 2001k, p. 5-13). The RI presented the following on sediment sources in this reach (URS Greiner, Inc. and CH2M Hill 2001k, p. 5-12):
Based on interpretation of aerial photographs from 1984, 1991, and 1998, the majority of sediment supplied to the South [Fork] appears to be from remobilization of floodplain sediment that has entered the South Fork from tributary watersheds.
Much new information on the source of sediments, sediment transport, and deposition (Bookstrom et al. 2004; Box et al. in press) has been developed and reported since the RI was published. The interpretations of sediment sources (for example, floodplain, riverbed, or river banks) and transport based on aerial photographs should be revisited in light of more recent data.
Chemical Speciation and Sediment Transport in the Lower Basin (CSM Unit 3)
Chemical Speciation
For the RI, chemical data that could be used to assess chemical speciation and transport mechanisms in groundwater were limited for the lower basin (URS Greiner, Inc. and CH2M Hill 2001i, pp. 2-10 and 2-11). Spruill (1993), who monitored four wells on the north side of the river upstream of Killarney Lake, reported chemically reducing conditions at a neutral pH. From these data, it was proposed that reductive dissolution of iron and manganese oxyhydroxides would occur and in turn release sorbed trace metals to groundwater (URS Greiner, Inc. and CH2M Hill 2001i, p. 2-11).
Additional testing of interstitial pore water and solids from contaminated, water-saturated levees and marshes in the lower Coeur d’Alene River area (Balistrieri et al. 2000)18 corroborated the work of Spruill (1993). The pH of pore water from all the sources tested was lower than the pH of river water, dissolved manganese and iron concentrations were elevated, and sulfate concentrations were below detection, all suggesting suboxic to anoxic conditions.
The fate of zinc under the oxidizing and reducing zones has important implications in remediation. In leaching studies that simulated dredging, Balistrieri et al. (2000) found that exposure of dredged riverbed sediments to water and air is highly likely to enhance zinc dissolution, making it necessary to consider treatment of water draining from the dredged sediment (see Chapter 8). EPA has stated (EPA 2004 [June 14, 2004]) the following:
… [remedial] alternative development was based on typical conceptual designs (TCDs). The TCDs were not considered sensitive to the issue of speciation; rather speciation data developed was a level of detail more appropriate to post-ROD detailed design work.
This statement is incorrect because zinc mobilization during dredging has significant water treatment cost implications that the ROD (EPA 2002) should address (see Chapter 8 for further discussion).
After the RI was issued, Balistrieri et al. (2002) summarized findings on metal speciation and mobility of metals in the Coeur d’Alene River basin that were more extensive than the information available at the time the RI
was issued. Analyses based on sequential extractions of samples were used to infer the speciation of metals in soils and sediments in the Coeur d’Alene River basin. Mineralogical analyses of samples were also reported. This study indicates that river sediments appeared to form authigenic sulfides near the surface.19 The levee region, which alternates between wet and dry conditions, contains both oxidizing zones (an area where sulfide minerals may be converted to oxide and carbonate minerals) and reducing zones (an area where oxygen is limited and minerals begin to lose any associated oxygen). The oxidizing zones contain oxide-coated sediment grains, whereas the reducing zones contain detrital (particulate material of organic origin) and authigenic carbonate and sulfide phases. Balistrieri et al. (2000, 2002) also reported that detrital sphalerite was found in oxidized levee samples, leading them to conclude that a fraction of the sphalerite was resistant to oxidation. The mechanism for rendering this fraction of the material resistant to oxidation is unclear, although it could have occurred by armoring (coating) of the sphalerite grains with other materials.
Researchers at the University of Wisconsin-Eau Claire have also completed considerable work on chemical speciation (Morrison et al. 1999; Rowe et al. 1999; Hooper and Mahoney 2000, 2001; Thornburg and Hooper 2001; Plathe et al. 2004; Strumness et al. 2004). Because these collective works focus heavily on chemical conditions that mobilize and immobilize metals in the various environments of the lower basin, this information should play an important role in EPA’s design phase for remedial action.
Sediment Transport
For water year 1999, the USGS used two gauging stations, one at Rose Lake and one at Harrison, to measure sediment transport (Clark and Woods 2001). Sand (material coarser than 65 μm) and fine (material finer than 65 μm) fractions were calculated separately and summed to determine the total suspended sediment discharge. Cumulative discharge for the year was calculated by summing the mean daily sediment discharges (URS Greiner, Inc. and CH2M Hill 2001i, p. 3-3). At the Rose Lake station, about 29,700 tons of suspended sediment (6,700 tons of sand plus 23,000 tons of fines) was transported. About 51,000 tons of suspended sediment was transported past Harrison in water year 1999, or about 34 tons per year per square mile, based on a drainage area of 1,475 square miles. Most of the sediment transport occurred during high-flow events, as would be expected. Suspended sediment and bedload samples were not analyzed for total metals,
and mass loading was estimated from total and dissolved surface-water data (URS Greiner, Inc. and CH2M Hill 2001i, p. 5-8).
To estimate sediment transport in the years before water year 1999, the calculated discharges from the gauges were integrated with sediment transport relationships developed in water year 1999 (see URS Greiner, Inc. and CH2M Hill 2001i, Tables 3.2-1 and 3.2-2). For the RI, photographs of various locations throughout the main stem of the Coeur d’Alene River were used in an attempt to estimate erosion rates and sources of sediments (URS Greiner, Inc. and CH2M Hill 2001i, pp. 3-6, 5-9).
Since the release of the RI in 2001, much new information on sources, deposition, and transport of sediments and lead concentrations of the sediments on the bed, banks, natural levees, and flood basins of the main stem of the Coeur d’Alene River has been developed and documented by the USGS. This information, which has been compiled by Bookstrom et al. (2004) along with the implications for remedial design, greatly advances the understanding of sediment transport and fate in the lower basin and should serve as an excellent guide for EPA in the remedial design process.
Chemical Speciation and Metal Transport in Lake Coeur d’Alene (CSM Unit 4)
The chemical speciation and transport of soluble metals, particularly zinc, in Lake Coeur d’Alene are complex phenomena governed by multiple interactions that are not completely understood. The amount of dissolved zinc within the lake is regulated by imported and exported quantities, diffusive flux from sediments because of changes in speciation, and interactions with other biotic and abiotic components in the water column and sediments. For instance, there may be scavenging mechanisms whereby soluble zinc stemming from the Coeur d’Alene River may associate with phytoplankton (that is, become sorbed to the organic matrix of organisms’ cells or incorporated into the silica of diatom frustules), which upon dying will fall out of the water column. At this point, the zinc may be sequestered (bound) in the sediments or may be liberated after the phytoplankton decompose. The liberated zinc may interact in situ with other components to sequester again, or it may migrate into the water column to undergo further interactions or be exported. The multitude of biotic and abiotic interactions includes complexation (binding) with dissolved, colloidal, or particulate organic matter, and association with other inorganic species, particularly with reactive iron and manganese oxyhydroxides. In addition, there may be seasonal or daily variations in hydrologic, geochemical, and biological interactions that drive these reactions, and the reactions may vary over the large and diverse area of the lake. Overall, the dynamics will remain difficult to monitor and predict with certitude.
Import/Export of Metals in Lake Coeur d’Alene
Although the dominance and interplay of these multiple chemical interactions will be difficult to ascertain, a more general picture of metal dynamics within the lake is accessible. Owing to a large data-collection effort conducted primarily by the USGS in conjunction with the Coeur d’Alene tribe, a tremendous amount of information exists to evaluate relative input and output of dissolved metals into Lake Coeur d’Alene. With these data, metal loads have been derived for a series of water years 1992-1997 and 1999. On an annual basis, more metals enter the lake than leave, and this attenuation is more pronounced for lead than zinc. The analysis shows that for the available years a median of 32% of dissolved zinc input was retained in the lake and 92% total lead was retained (URS Greiner, Inc. and CH2M Hill 2001l, p. 5-4). Upon considering the input and output on a monthly basis, the picture is less clear. During most of the year, zinc inputs exceed output; however, in the spring of the year, the reverse is true (Figure 4-2). It should be noted that data are used to estimate this one year; however, the dynamics could have large interyear differences, and the uncertainty of these monthly estimates is not depicted in this figure derived from the RI.
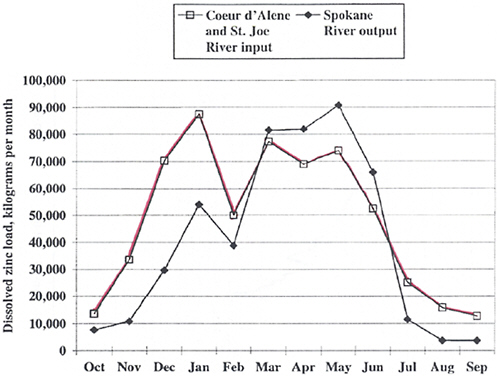
FIGURE 4-2 Measured dissolved zinc load input (Coeur d’Alene and St. Joe Rivers) and output (Spokane River) for Coeur d’Alene Lake, water year 1999. SOURCE: URS Greiner, Inc. and CH2M Hill 2001l.
Mass-Balance Modeling for Lake Coeur d’Alene
Within the RI, EPA developed a mass balance for various metals within the lake (see Figure 4-3 for dissolved zinc). The mass-balance model for dissolved zinc is based on measured and estimated components. The riverine inputs and Lake Coeur d’Alene output are derived from USGS water monitoring from water year 1999 (Woods 2001), the benthic input is estimated from USGS studies (Kuwabara et al. 2000), and the transformation percentage is essentially a factor devised to accommodate the excess 445,000 kg/year input.
The benthic flux estimate was derived from USGS work conducted to evaluate the significance of releases of metals from the sediments within the lake compared with inputs from the Coeur d’Alene River (Kuwabara et al. 2000) (essentially to determine whether the sediment is serving as a source of dissolved metals by emitting the constituent or as a sink by consuming it). Three techniques were tested, but the in situ benthic-flux chamber method was chosen as being most representative for these calculations (URS Greiner, Inc. and CH2M Hill 2001l, p. 5-19). This study used a benthic lander, a rectangular acrylic chamber that isolates 1,500 cubic centimeters of lake-bottom sediment surface and the overlying water. Deployment is for anywhere from a half day to 2 days and water is sampled throughout the deployment. Figure 4-4 presents results from this study, illustrating an increase in dissolved zinc and a decrease in dissolved oxygen over time in the benthic chamber. These results indicate that the sediments do serve as a source for zinc under the conditions tested.
The benthic lander data for zinc were obtained from two or three deployments at two locations (Table 4-3). These data were further win-
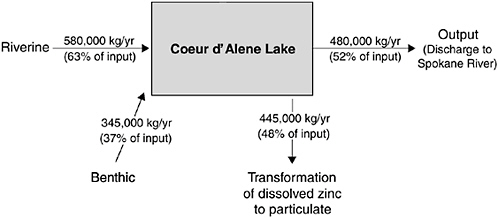
FIGURE 4-3 Dissolved zinc mass balance of Lake Coeur d’Alene. SOURCE: Adapted from URS Greiner, Inc. and CH2M Hill 2001l, Table 5.6-1.
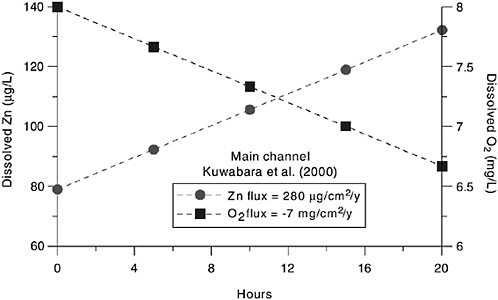
FIGURE 4-4 Zinc and oxygen concentrations over time within the benthic lander chamber. SOURCES: Balistrieri 2004; data from Kuwabara et al. 2000.
nowed in the RI where results from the two sites are averaged20 to estimate the annual flux from the entire lake by multiplying the flux estimate by the surface area of the lake bottom. The derived number represents a major contribution of soluble zinc to the lake in the mass-balance model (37%; see Figure 4-3).
Considering the large surface area of contaminated sediments in the lake, estimated at 42 square miles (URS Greiner, Inc. and CH2M Hill 2001l, Table 5.6-3), the spatial coverage of the lander experiments is necessarily limited. The temporal component is also limited considering the experimental time period spans only a couple of days in August 1999. Zinc dynamics could have a strong seasonal component, yet this insight is not available from the benthic flux studies. Indeed, these limitations are recognized and stated by EPA (URS Greiner, Inc. and CH2M Hill 2001l, p. 5-24):
The benthic fluxes were less robust because of their limited spatial and temporal resolution; benthic flux was measured only during mid-August of the 1999 water year and at only two locations. No information
20 |
Actually, the flux data for zinc at each of the two sites are averaged, and then the average of these two averages is used as the overall benthic flux estimate. |
TABLE 4-3 Benthic Flux Results
Deploy # |
Station Location |
Zn Flux (μg/cm2/y) |
2 |
Mica Bay |
451.0 ± 100.2 |
4 |
Mica Bay |
243.4 ± 33.4 |
1 |
Main Channel |
348.4 ± 71.6 |
2 |
Main Channel |
198.1 ± 28.6 |
3 |
Main Channel |
295.9 ± 45.3 |
SOURCE: Kuwabara et al. 2000. |
is available on the magnitude of temporal variations of benthic flux in Coeur d’Alene Lake.
Overall, although the benthic flux work is important and interesting, it is quite limited spatially and temporally; consequently, the present database has limited utility in the evaluation of annual benthic flux for the entire lake.
Another limiting component of the mass balance presented in Figure 4-3 relates to estimates of the dissolved zinc converted to particulate form. This parameter is derived from the other components of the mass balance to compensate for the differences between the estimated input and the measured output of the lake. Figure 4-3 indicates that 48% of the estimated input is converted into particulate form. This value is estimated simply by subtracting the measured output of the lake from the estimated input (measured riverine loading + estimated benthic flux). There have been no measurements or sediment trapping studies to estimate or verify this value even though the large removal mechanism is a central component describing the lake’s mass balance. Indeed, the magnitude of this mass-balance estimate is dependent on the benthic flux estimate because the benthic flux estimate represents a large portion of the lake’s loading. Again, the RI states these limitations: “The removal of dissolved zinc from the water column was assumed to be due to the transformation to the particulate fraction. However, there are no sediment data to support this assumption” (URS Greiner, Inc. and CH2M Hill 2001l, p. 5-35).
To move beyond an annual mass-balance model, a model depicting zinc loads imported and exported to the lake through time was developed. However, this model has the same limitations as the annual mass balance. Here, the benthic flux input parameter, as designated in the RI, results from the same work described above and has the same limitations regarding spatial and temporal resolution. And, again, the “transformation parameter” (in this model, a “scavenging coefficient”) is solely a fitting factor designed to comport the model’s output with the measured zinc concentrations in Lake Coeur d’Alene discharge. In this case, the scavenging param-
eter varies by an order of magnitude throughout the year so that the model output will comport with measured data (URS Greiner, Inc. and CH2M Hill 2001l, p. 5-34). Although there may be biological and geochemical processes responsible for removing dissolved zinc that are of this magnitude and variability, no studies exist to support the conclusion (URS Greiner, Inc. and CH2M Hill 2001l, p. 5-35).
In general, the mass-balance models do not reflect a firm understanding of the lake’s metals dynamics, considering that close to 40% of the input is derived from useful, but very limited, benthic flux data, the mechanism driving 50% of the removal has not been measured or monitored, and the removal mechanism is not understood.
Inflow Plume Routing in Lake Coeur d’Alene
Preferential routing of metals-rich discharges from the Coeur d’Alene River through the lake is another phenomenon believed to affect the disposition of metals (URS Greiner, Inc. and CH2M Hill 2001l, p. 5-9). As water enters the lake from the river, uniform mixing may not occur. Temperature differences between river and lake waters are believed to affect density to such an extent that warmer river waters will spread as a buoyant plume over the top of colder lake waters (overflow) with limited mixing, or colder river waters will move to the bottom of the warmer water in the lake (underflow) again with limited mixing. In overflow conditions with substantial flows, there may be preferential transport of dissolved and suspended constituents to the outlet of Lake Coeur d’Alene into the Spokane River, and this may explain how particulate lead reaches the Spokane River. Researchers (Brennan et al. 2000; P.F. Woods, unpublished material, USGS, 2000, as cited in URS Greiner, Inc. and CH2M Hill 2001l) from the USGS monitored this phenomenon in June 1999. Their results documented a layer of warmer water above cooler water; the warmer water contained elevated concentrations of total lead, decreased zinc concentrations, and decreased light transmission. This profile of physical and chemical constituents is similar to the presumed riverine sources. From a fate and transport perspective, the implication is that the preferential routing of overflow will carry constituents (principally particulate lead) through Lake Coeur d’Alene into the Spokane River instead of the lake serving as a settling basin where particulate-bound lead can settle from the water column. Indeed, the USGS data (Brennan et al. 2000; P.F. Woods, unpublished material, USGS, 2000, as cited in URS Greiner, Inc. and CH2M Hill 2001l) from this event indicated elevated lead concentrations transported through the lake. There is a concern in the Spokane River about accumulation of lead-enriched sediments in eddy areas and beaches where humans may recreate. Consequently, the monitoring and understanding of these events are important in compre-
hending the dynamics of pollutant transfer in the lake. The USGS has continued its efforts to document and understand this phenomenon through additional sampling. These efforts will remain important in understanding transport of contaminated sediments through the system.
The RI (URS Greiner, Inc. and CH2M Hill 2001l, p. 5-9) further attempts to document the overflow phenomenon by comparing water temperatures from the Coeur d’Alene and St. Joe Rivers with temperatures at the deepest point in the lake. This text states that
overflow was the most common mode of inflow plume routing, occurring in about 60% of the comparisons. Interflow or underflow each occurred in about 20% of the comparisons. Overflow was present in all months except October, November, and December.
However, it appears difficult to make these statements about an overflow or underflow condition with the information provided. Indeed, this conclusion apparently is drawn by noting a difference of only a few degrees Celsius between the rivers and a mid-lake station located several miles away, and it does not present evidence that the upper water column is preferentially enhanced in chemical constituents derived from riverine sources. Although overflow or underflow conditions may have been occurring, and the month-by-month breakdown may make sense in terms of expected seasonal water temperatures, existence of the overflow/underflow phenomena during these months is not established by the data presented. The aforementioned USGS monitoring will be important to document the ubiquity of the overflow phenomenon.
Thermal Stratification
While the implications of overflow and underflow of the Coeur d’Alene River plume through Lake Coeur d’Alene are discussed in the RI, little discussion is provided regarding the effect of thermal stratification and turnover on metals dynamics in the lake. According to the RI (URS Greiner, Inc. and CH2M Hill 2001j, p. 5-9), Lake Coeur d’Alene is dimictic (thermal stratification breaks down and the water column undergoes mixing, or turnover, in the fall and spring). During stratification, which typically occurs in the summer months, the lower water column (hypolimnion) does not mix with upper water column (epilimnion). Constituents (for example, dissolved metals) that build up in the hypolimnion during this period of thermal stratification can be released during turnover and affect the release of metals from the lake. The RI provides data suggesting that the hypolimnion contains elevated dissolved zinc compared to the epilimnion during July and August when the lake would be expected to be stratified (URS
Greiner, Inc. and CH2M Hill 2001l, Tables 5.7-9 and 5.7-10). However, the potential for stratification and turnover to affect metals distribution and discharge is not examined.
Water-Quality Study of Lake Coeur d’Alene: Are Nutrients a Problem?
An extensive water-quality study of Lake Coeur d’Alene was initiated in 1991 by the Idaho Department of Environmental Quality (IDEQ), Coeur d’Alene tribe, and USGS with three objectives:
-
Determine the lake’s ability to receive and process nutrients (phosphorus and nitrogen) to devise measures that will prevent water-quality degradation.
-
Determine the potential for releases of heavy metals from lakebed sediments into the overlying lake water.
-
Develop information to support a lake management plan that will identify actions needed to meet water-quality goals.
Woods and Beckwith (1997) report on this study and provide an evaluation of the nutrient and trace-metal balance of the lake. A nutrient load/lake response model was used to simulate Lake Coeur d’Alene’s limnologic21 responses to alterations in water and nutrient loads delivered to the lake. The empirical mathematical model simulated the following eutrophication-related variables22: concentrations of total phosphorus, total nitrogen, and chlorophyll a; secchi-disc transparency;23 and hypolimnetic dissolved oxygen deficit. The model was calibrated with 1991 data. After calibration, the model’s applicability to Lake Coeur d’ Alene was verified with 1992 data. After calibration and verification, the model was used to simulate the lake’s responses to various nutrient-management scenarios. The following two principal questions were addressed:
-
Would large increases in nutrient loads cause the lake’s hypolimnion to become anoxic?
-
Would the lake’s water quality be substantially improved by large reductions in nutrient loads?
Woods and Beckwith (1997) found that much more than a quadrupling of nutrient input would be required for the northern portion of the lake to become anoxic (devoid of oxygen)—a very unlikely event. Nutrient reduction from wastewater treatment plant discharges to the lake was predicted to produce the greatest improvement in water quality as measured by chlorophyll a and secchi-disc transparency.
The Coeur d’Alene Lake Management Plan Update (IDEQ 2004) sets forth the present status of actions for the management of the lake. The technical basis for the lake management plan largely remains based on these studies, as well as more recent water-quality monitoring conducted by USGS, the Coeur d’Alene tribe, and IDEQ.
Speciation of Metals in Lake Sediments
A number of studies have been conducted to determine zinc speciation in the lake sediments, but none is without associated possible error due to sampling, sample handling, or analysis factors. Two commonly used procedures to infer the chemical speciation of metals in aquatic sediments are the Tessier sequential fractionation procedure (Tessier et al. 1979) and the acid volatile sulfide–simultaneously extracted metal (AVS-SEM) procedure (Allen et al. 1993). The Tessier procedure is based on (1) extracting a sediment sample with extractants of increasing strength and (2) determining the metal that is released with each extractant. These releases are related to operationally defined geochemical phases. The AVS-SEM analysis is based on adding cold hydrochloric acid to a sediment sample and trapping the volatilized hydrogen sulfide. The molar amount of sulfide released is compared with the sum of the moles of trace metal, excluding iron, dissolved in the acid. If the amount of sulfide (AVS) exceeds that of the metal (SEM), it can be concluded that there is a sufficient amount of sulfide for the metals (in this case lead, zinc, cadmium, and copper) to be present as sulfides rather than as more soluble oxyhydroxides, carbonates, or sulfates. Pyrite is not detected in this procedure. The iron dissolved in the acid is not included in the comparison because it is much more soluble than are the sulfides of the trace metals. Thus, FeS (iron sulfide) acts as a reservoir to maintain sulfur for precipitation of these trace metals.
Horowitz et al. (1993) conducted an extensive sampling of the surficial sediments, which they found to be enriched in a number of trace elements. Samples were freeze-dried before analysis, resulting in the oxidation of the more labile (readily broken down) sulfide compounds. A number of the samples were subjected to a two-step procedure to partition the trace met-
als into an iron oxide phase and an organic/sulfide phase. The acidic first step would dissolve not only the metal oxides but also many of the metal sulfides in the same manner as the AVS-SEM procedure, which uses acid to release the metal and the sulfide. Therefore, the presence of metal sulfides in the sediment cannot be ruled out.
Harrington et al. (1998) used the Tessier sequential fractionation procedure (Tessier et al. 1979) to characterize the phase associations of trace metals in core samples taken from the lake. However, as noted by Horowitz et al. (1999), the cores were sectioned at 8 cm intervals and Harrington et al. (1998) reported the redox boundary24 to be at approximately 2 cm. The first section would have a mixture of oxidized and reduced sediment present. At least a portion of any zinc sulfide present would have been dissolved in the acidic oxalate solution that is designed to characterize the metal oxide fraction. Harrington et al. (1999) used the AVS-SEM procedure, indicating that a substantial amount of the zinc may be present as zinc sulfide. However, the fact that the samples were from cores that were sectioned at 8 cm intervals and AVS-SEM assayed at 4 cm intervals casts doubt on the association of the metals at the water-sediment interface.
Lake Coeur d’Alene Studies: The Bottom Line
What can be easily understood from evaluating the complex phenomena in Lake Coeur d’Alene is that the better the data sets, the more thorough the understanding and ability to make informed statements about metals dynamics in the lake. The committee has found that there are large amounts of high-quality monitoring data that have been collected on the lake, particularly by the USGS, the Coeur d’Alene tribe, and IDEQ. The use of this information permits an understanding of the overall behavior of the variety of metal contaminants within the lake and, for example, elucidates the likelihood that overflow events can preferentially transport materials through the lake under certain conditions. However, data documenting metal interactions, internal cycling, and benthic flux are limited.
In future studies of metals in the sediment of the lake, more attention should be given to certain aspects of sampling, analysis, and interpretation. In particular, the depth of the oxidized layer will vary seasonally as a consequence of oxidation (breakdown) of algal detritus. The seasonality of the thickness of the oxidized layer should be evaluated along with the concurrent changes in the sulfide contained in the sediment. Coring studies on the lake provide great insight to the historical depositional pattern on
metals-enriched sediments (Horowitz et al. 1995; Woods 2004). These cores could also provide a useful diagnostic on the long-term trends of the metal content and amount of deposited sediments and potentially on the effect of the basin’s remedial activities on sediment transport and deposition in Lake Coeur d’Alene (A. Horowitz, USGS, Atlanta, GA, unpublished material, June 17, 2004).
To comprehend the lake dynamics and the effect of various management practices on phytoplankton production and metal fluxes from the sediment, additional experiments will be necessary. For example, water-column sedimentation trap experiments would be useful to elucidate the removal of dissolved metals from the water column by phytoplankton. The USGS is planning to develop a model that predicts the flux of metals from the sediment to the overlying water such as those discussed by Di Toro (2001). Such a model would use nutrient input to compute primary production. Appropriate sampling, including seasonal sampling of sediments for the analysis of AVS and SEM, will be needed for model calibration. The committee particularly notes the potential for nutrient management actions to affect the zinc concentrations in the lake water.
The committee recognizes that some studies are ongoing and supports further monitoring and modeling studies to understand the interplay between the hydrologic, geochemical, and biological phenomena driving the disposition of metals within the lake. The committee’s understanding has benefited from the available basic information on hydrologic and chemical data (particularly metals) and suggests a continued development of such data in order to assess long-term trends.
Chemical Speciation and Sediment Transport in the Spokane River (CSM Unit 5)
Chemical Speciation
It appears that few chemical speciation studies have been conducted in the Spokane River basin. As provided by Kadlec (2000) in an ecologic risk analysis, Bailey and Saltes (1982) demonstrated that most of the zinc is in soluble form in the Spokane River. Johnson et al. (1990) reported that 73% of the zinc was in the dissolved phase (<0.45 μm diameter) in Lake Roosevelt, and Pelletier (1994) reported the ratio of dissolved to total fractions to be 69% for cadmium, 18% for lead, and 83% for zinc. Naturally occurring organic and inorganic solids did not appear to influence the bioavailability of these metals (Bailey and Saltes 1982; Kadlec 2000). Lead was reported as being mostly associated with suspended particles (Kadlec 2000).
Subsequent to publication of the RI, studies by Box and Wallis (2002) and Box et al. (in press) indicate that zinc in the Spokane River is mostly in
dissolved form during low flows, but during high-flow events, zinc in the sediments is mobilized and a significant portion of the zinc load is in particulate form.
Sediment Transport
The largest sources of sediment (URS Greiner, Inc. and CH2M Hill 2001m, p. 5-9) to the Spokane River are remobilization of channel bed material, bank erosion, and tributary channels. Lake Coeur d’Alene is a source of the smallest and lightest particles, as discussed in the preceding section of this chapter. The fine-grained sediments in the Spokane River are contaminated with lead and zinc. Metal concentrations generally decrease from upstream to downstream (URS Greiner, Inc. and CH2M Hill 2001m, p. 5-1). Sediment transport is controlled by dams and reservoirs on the Spokane River, with large amounts of sediment deposited in the reservoirs; however, fine-grained sediments appear to be transported through the reservoirs (URS Greiner, Inc. and CH2M Hill 2001m, p. 5-9).
CONCLUSIONS AND RECOMMENDATIONS
Conclusion 1
The EPA did not fully consider the importance of the interacting processes of surface- and groundwater flow, metal flux, metal storage in sediments, and metal-bearing sediment transport and deposition with relevant aspects (fish habitat, forest management, climatologic variability, etc.) of the Coeur d’Alene River basin system. Because the basin has not been considered in the framework of a system and inadequate attention has been devoted to hydrologic and climatic variabilities, in particular, the CSMs seemingly are based primarily on average conditions.
Because characterization of the CSMs and the conclusions and decisions that stem from these models are based on average conditions, these decisions—for example, the definition of possible remedies—may not be fully protective of aquatic species or robust enough to withstand severe events. Extreme events are more important than averages because organisms respond to extreme events. Solid-phase contaminants are often transported during high flow (an extreme event), and concentrations of dissolved-phase contaminants are often highest during low flow (an extreme event).
Conclusion 2
The way EPA has compartmentalized the basin into OUs for remediation is inconsistent with a “systems approach” (see Box 4-1) to investi-
gating the basin, and this compartmentalization has created some serious technical difficulties and public perception problems for EPA.
The current OU structure may have made sense in the beginning of the Superfund investigations, but it is inconsistent with the natural hydrologic and chemically linked systems operating within the basin. A systems approach based on watershed boundaries is a more appropriate means of properly characterizing contaminant sources and paths of contaminant transport. Although the committee recognizes that the OU approach was adopted by EPA to prioritize human health risks, the artificial constraints have created problems for EPA in protecting fish downstream of the box, because a large portion of the dissolved zinc (modeled at 41%) comes from sources that apparently cannot be addressed by OU-3 actions. Public perception problems arise from the fact that the agency seems to have reversed its original position, which was to deal with the environmental problems outside of the box using programs other than Superfund. This reversal undermined the public’s trust and confidence.
Conclusion 3
The total number of samples collected from the entire basin area was small in relation to the large area extent of the basin and the complexity of the site, and source terms25 were not well defined; nevertheless, trends related to contaminant transport and fate, especially for surface water, were definable from the samples that were collected.
17,000 samples were collected throughout the basin, and 1,080 mining-related source areas were identified. Approximately, 160 (15%) of these source areas were sampled with about five surface and near-surface samples collected from most tailings and sediment sources of 5 acres or more. Because the basin is such a large and chemically and hydrologically complex site—and contaminant distribution can be very heterogeneous with hot spots being less than an acre in size—this number of samples, although large, is insufficient to quantify the source terms. Leachability data were not obtained to support OU-3 decision making. Measured increases in dissolved metal loadings in streams were used to infer sources, such as nearby floodplain sediments and tailings.
Conclusion 4
Estimated average mass loading of metals to the Coeur d’Alene River and Lake adequately depict an overall description of contaminants moving through the basin, but such data should not be substituted for comprehensive source characterization and remedy design for worst-case conditions.
The committee commends the agency for cooperating with other federal and state entities in conducting a variety of new studies that will provide new and improved interpretations of contamination in the basin and can be used in the next steps of the Superfund process.
Conclusion 5
Understanding the dynamics of groundwater movement, the incorporation of dissolved metals from the aquifer materials, and the complex relationship between surface water and the shallow groundwater aquifer will require comprehensive study and is necessary because groundwater is the primary source of dissolved metals into the surface water of the basin.
The investigations conducted to document concentrations of dissolved metals within the basin focused primarily on monitoring surface-water concentrations. A more limited campaign to sample groundwater was undertaken. Yet most of the zinc load in the basin is contributed by groundwater. Understanding the dynamics of groundwater movement and the incorporation of dissolved metals from the aquifer will undeniably require additional characterization.
Conclusion 6
Selecting lead and zinc as indicators of COPCs is reasonable, but caution is advised in extrapolating the behavior of these metals to other contaminants.
Zinc accounts for about 96% of the dissolved metal loading to Lake Coeur d’Alene. Lead is primarily transported as a particulate and is also a metal of major concern. Zinc, which is cationic, may have different transport characteristics from arsenic, which is anionic and undergoes redox transformations under the environmental conditions of the basin.
Conclusion 7
EPA addressed background determinations in a manner consistent with the agency’s established guidelines and is commended for determining site-specific background concentrations of COPCs. The background concentrations developed for the ROD were reasonable, but these background concentrations were not used appreciably, with the exception of the Spokane
River, to select remedial goals or select target cleanup levels when used in conjunction with risk-based values. This decision is appropriate because of the disparity between the cleanup levels and the background levels.
EPA followed guidelines, as understood by the committee, for determining background concentrations for soils, sediments, and surface waters in the various basin areas. Background concentrations typically are determined to estimate the extent of contamination and to assist in selecting remedial goals or target cleanup levels. The agency compared contaminant levels with background. However, background was not used appreciably, except for the Spokane River, for the latter purpose, because under the interim cleanup, achieving background is irrelevant. There is a large disparity between the contaminant levels and background concentrations, particularly for soils and sediments. Although coring studies and techniques for background were appropriate, aspects of the sampling and background derivation methodologies were problematic. However, this has little practical effect because proposed remedial actions are not governed by background concentrations.
Conclusion 8
Owing to the complexity of metals dynamics in Lake Coeur d’Alene, additional supporting technical information is needed to develop an effective lake management plan.
The relationship between eutrophiciation and metals release is not completely understood. Zinc transport through the lake is a complex and dynamic process with seasonal variations, and the understanding of this process is continuing to evolve.
Conclusion 9
Information on chemical speciation of contaminants is limited and was not considered to any significant extent in decision making in the ROD. Recently available information on the sources, deposition, and transport of metals and sediments will be especially important in the design phase of the Superfund process.
Understanding the chemical speciation of metals is important for understanding the dissolution of metals from sources, such as tailings and floodplain sediments, and their bioavailability. Some chemical speciation studies of metals were undertaken in Canyon Creek and Ninemile Creek, and similarly important studies were conducted to estimate dissolution of zinc during dredging in the lower basin. RI sediment-transport studies were limited to water year 1999, but extensive studies by USGS have been ongo-
ing in the lower basin and will provide much needed information for remedial design.
Recommendation 1
EPA is encouraged to incorporate in remedial planning new data that have been made available by USGS, Coeur d’Alene tribe, U.S. Fish and Wildlife Service, IDEQ, and others since issuance of the ROD. Furthermore, the agency is urged to proceed, as planned, with more-thorough source identification before proceeding with cleanup to ensure the location, magnitude, and disposition of contaminant sources.
Recommendation 2
An understanding of dissolved metals, particularly zinc, that accounts for the delivery to and from groundwater and surface waters needs to be developed. The chemical and hydrological components need to be sufficiently rigorous to permit use of the information to evaluate the consequences of alternative remedial actions to the input of dissolved metals to the basin.
REFERENCES
Allen, H.E., G. Fu, and B. Deng. 1993. Analysis of acid-volatile sulfide (AVS) and simultaneously extracted metals (SEM) for the estimation of potential toxicity in aquatic sediments. Environ. Toxicol. Chem. 12(8):1441-1453.
Bailey, G.C., and J. Saltes. 1982. Fishery Assessment of the Upper Spokane River. Pub. No. 82-e01. WA-57-1010. Washington Water Research Center, Washington State University, Pullman, WA. June [online]. Available: http://www.ecy.wa.gov/biblio/82e01.html [accessed Feb. 7, 2005].
Balistrieri, L.S. 2004. Benthic Fluxes in Lake Coeur d’Alene. Presentation at the Third Meeting on Superfund Site Assessment and Remediation in the Coeur d’Alene River Basin, June 17, 2004, Coeur d’Alene, ID.
Balistrieri, L.S., A.A. Bookstrom, S.E. Box, and M. Ikramuddin. 1998. Drainage From Adits and Tailings Piles in the Coeur d’Alene Mining District, Idaho: Sampling, Analytical Methods, and Results. USGS Open-File Report 98-127. Menlo Park, CA: U.S. Department of the Interior, U.S. Geological Survey. 19 pp.
Balistrieri, L.S., S.E. Box, A.A. Bookstrom, and M. Ikramuddin. 1999. Assessing the influence of reactive pyrite and carbonate minerals on the geochemistry of drainage in the Coeur d’Alene mining district. Environ. Sci. Technol. 33(19):3347-3353.
Balistrieri, L.S., S.E. Box, M. Ikramuddin, A.J. Horowitz, and K.A. Elrick. 2000. A Study of Porewater in Water Saturated Sediments of Levee Banks and Marshes in the Lower Coeur d’Alene River Valley, Idaho: Sampling, Analytical Methods and Results. Open-File Report 00-126. Menlo Park, CA: U.S. Department of the Interior, U.S. Geological Survey. 62 pp.
Balistrieri, L.S., S.E. Box, A.A. Bookstrom, R.L. Hooper, and J.B. Mahoney. 2002. Impacts of historical mining in the Coeur d’Alene River Basin. Pp. 1-34 in Pathways of Metal Transfer from Mineralized Sources to Bioreceptors: A Synthesis of the Mineral Resources Program’s Past Environmental Studies in the Western United States and Future Research Directions, L.S. Balistrieri, L.L. Stillings, R.P. Ashley, and L.P. Gough, eds. U. S. Geological Survey Bulletin 2141. Reston, VA: U.S. Department of the Interior, U.S. Geological Survey [online]. Available: http://geopubs.wr.usgs.gov/bulletin/b2191/ [accessed Dec. 1, 2004].
Barton, G. 2000. Feasibility Study Report, Final. Appendix D (as cited in URS Greiner, Inc. and CH2Hill 2001c).
Barton, G.J. 2002. Dissolved Cadmium, Zinc and Lead Loads from Ground-Water Seepage into the South for Coeur d’Alene River System, Northern Idaho, 1999. Water-Resources Investigations Report 01-4274. Boise, ID: U.S. Department of the Interior, U.S. Geological Survey. 130 pp [online]. Available: http://purl.access.gpo.gov/GPO/LPS39228 [accessed Dec. 1, 2004].
Beckwith, M.A. 1996. Water-Quality Data Collected During Floods in the Coeur d’Alene River, Northern Idaho, February 1996. U.S. Geological Survey Fact Sheet FS-96-219. Reston, VA: U.S. Geological Survey. 4 pp.
Beckwith, M.A., P.F. Woods, and C. Berenbrock. 1997. Trace-Element Concentrations and Transport in the Coeur d’Alene River, Idaho, Water Years 1993-94. Open-File Report 97-398. Boise, ID: U.S. Geological Survey.
Bookstrom, A.A., S.E. Box, J.K. Campbell, I. Foster, and B.L. Jackson. 2001. Lead-Rich Sediments, Coeur d’Alene River Valley, Idaho: Area, Volume, Tonnage, and Lead Content. Open-File Report 01-140. Menlo Park, CA: U.S. Department of the Interior, U.S. Geological Survey [online]. Available: http://geopubs.wr.usgs.gov/open-file/of01-140/ [accessed Nov. 29, 2004].
Bookstrom, A.A., S.E. Box, R.S. Fousek, J.C. Wallis., H.Z. Kayser, and B.L. Jackson. 2004. Baseline and Historical Depositional Rates and Lead Concentrations, Floodplain Sediments: Lower Coeur d’Alene River, Idaho. U.S. Geological Survey Open-File Report 2004-1211. U.S. Department of the Interior, U.S. Geological Survey, Spokane, WA [online]. Available: http://pubs.usgs.gov/of/2004/1211/ [accessed June 23, 2005].
Box, S.E., and J.C. Wallis. 2002. Surficial Geology along the Spokane River, Washington and Its Relationship to the Metal Content of Sediments (Idaho-Washington Stateline to Latah Creek Confluence). Open File Report 02-126. Spokane, WA: U.S. Department of the Interior, U.S. Geological Survey [online]. Available: http://geopubs.wr.usgs.gov/open-file/of02-126/ [accessed March 21, 2005].
Box, S.E., A.A. Bookstrom, and W.N. Kelley. 1999. Surficial Geology of the Valley of the South Fork of the Coeur d’Alene River, Idaho, Draft Version, U.S. Geological Survey, Spokane, WA. October 4, 1999. (Document ID 1110378 in Bunker Hill Basin-Wide Remedial Administrative Record, Data CD8. U.S. Environmental Protection Agency, Region 10, September 2002.)
Box, S.E., A.A. Bookstrom, M. Ikramuddin, and J. Lindsay. 2001. Geochemical Analyses of Soils and Sediments, Coeur d’Alene Drainage Basin, Idaho: Sampling, Analytical Methods, and Results. Open-File Report 01-139. Spokane, WA: U.S. Department of the Interior, U.S. Geological Survey [online]. Available: http://geopubs.wr.usgs.gov/open-file/of01-139/ [accessed Feb. 7, 2005].
Box, S.E., A.A. Bookstrom, and M. Ikramuddin. In press. Stream-Sediment Geochemistry in Mining-Impacted Streams: Sediment Mobilized by Floods in the Coeur d’Alene-Spokane River Drainage, Idaho and Washington. USGS Scientific Investigation Report SIR 2005-5011. U.S. Department of the Interior, U.S. Geological Survey.
Brennan, T.S., A.M. Campbell, A.K. Lehmann, and I. O’Dell. 2000. Water Resources Data, Idaho, Water Year 1999, Vol. 2. Upper Columbia River Basin and Snake River Basin Below King Hill. Water-Data Report ID-99-2. U.S. Department of the Interior, U.S. Geological Survey, Denver, CO. 462 pp.
CH2M Hill. 2004a. Dissolved Metal Loading from Groundwater to the South Fork of the Coeur d’Alene River, Bunker Hill Superfund Site, Idaho, Draft Final Report, June, 2004. Work Assignment No. 015-TA-TA-10X9. CH2M Hill Project No. 152210.ET.23. Prepared for U.S. Environmental Protection Agency, Region 10, Seattle, WA, by CH2M Hill, Spokane, WA.
CH2M Hill. 2004b. High-Flow Surface Water Sampling Event, March 2003, Bunker Hill Superfund Site. Final Technical Memorandum for Cami Grandinetti, EPA, from Steve Hicks and Craig Sauer, CH2M Hill. June 7, 2004.
Clark, G. 2003. Occurrence and Transport of Cadmium, Lead, and Zinc in the Spokane River Basin, Idaho and Washington, Water Years 1999-2001. Water-Resources Investigations Report 02-4183. Boise, ID: U.S. Department of the Interior, U.S. Geological Survey [Online]. Available: http://id.water.usgs.gov/PDF/wri024183/index.html [accessed Dec. 1, 2004].
Clark, G.M., and P.F. Woods. 2001. Transport of Suspended and Bedload Sediment at Eight Stations in the Coeur d’Alene River Basin, Idaho. Open-File Report 00-472. Boise, ID: U.S. Department of the Interior, U.S. Geological Survey [online]. Available: http://purl.access.gpo.gov/GPO/LPS46003 [accessed Dec. 1, 2004].
Dames and Moore. 1991. Bunker Hill RI/FS Report, Task 3, Revised Final Hydrogeologic Assessment, Vol. 1. Prepared for U.S. Environmental Protection Agency, Region 10, by Dames and Moore, Denver, CO. June 11, 1991.
DiToro, D.M. 2001. Sediment Flux Modeling. New York: Wiley.
Dzombak, D.A. 1986. Toward a Uniform Model for the Sorption of Inorganic Ions on Hydrous Oxides. Ph.D. Thesis, Massachusetts Institute of Technology, Cambridge, MA.
EPA (U.S. Environmental Protection Agency). 1988. Guidance for Conducting Remedial Investigations and Feasibility Studies under CERCLA, Interim Final. EPA 540/G-89/004. OSWER 9355.3-01. Office of Emergency and Remedial Response, U.S. Environmental Protection Agency, Washington, DC [online]. Available: http://www.epa.gov/superfund/resources/remedy/pdf/540g-89004.pdf [accessed Jan. 11, 2005].
EPA (U.S. Environmental Protection Agency). 1994. Guidance for the Data Quality Objectives Process, EPA QA/G-9. EPA/600/R-96/055. Office of Research and Development, Washington, DC. September [online]. Available: http://www.epa.gov/correctiveaction/resource/guidance/qa/epaqag4.pdf [accessed July 25, 2005].
EPA (U.S. Environmental Protection Agency). 2000. First Five-Year Review of the Non-Populated Area Operable Unit, Bunker Hill Mining and Metallurgical Complex, Shoshone County, Idaho [online]. Available: http://www.epa.gov/r10earth/offices/oec/First%205-Year%20Review%20Non-Pop.pdf [accessed Nov. 29, 2004].
EPA (U.S. Environmental Protection Agency). 2002. The Bunker Hill Mining and Metallurgical Complex: Operable Unit 3, Record of Decision. U.S. Environmental Protection Agency, Region 10. September 2002 [online]. Available: http://yosemite.epa.gov/.../cbc45a44fa1ede3988256ce9005623b1/$FILE/ATTBRN4D/Part%201%20Declaration.pdf [accessed Dec. 1, 2004].
EPA (U.S. Environmental Protection Agency). 2004. EPA Responses to NAS Questions (different dates).
Gott, G.B., and J.B. Cathrall. 1980. Geochemical-Exploration Studies in the Coeur d’Alene, Idaho and Montana. U.S. Geological Survey Professional Paper 1116. Washington, DC: U.S. Government Printing Office.
Harrington, J.M., M.J. LaForce, W.C. Rember, S.E. Fendorf, and R.F. Rosenzweig. 1998. Phase associations and mobilization of iron and trace elements in the Coeur d’Alene Lake, Idaho. Environ. Sci. Technol. 32(5):650-656.
Harrington, J.M., S.E. Fendorf, B.W. Wielinga, and R.F. Rosenzweig. 1999. Response to comment on “Phase associations and mobilization of iron and trace elements in Coeur d’Alene Lake, Idaho.” Environ. Sci. Technol. 33(1):203-204.
Hooper, R.L., and J.B. Mahoney. 2000. Constraining Contaminant Transport: Lead and Zinc Speciation in Fluvial Subenvironments, Lower Coeur d’Alene River Valley, Idaho. Geological Society of America Abstracts with Programs (Annual Meeting, Nov. 12-16, 2000, Reno, NV). 32(7):A125.
Hooper, R.L., and J.B. Mahoney. 2001. Metal transport, heavy metal speciation and microbial fixation through fluvial sub-environments, Lower Coeur d’Alene River Valley, Idaho. EOS Trans. AGU 82(47):F199-F200.
Hornig, C.E., D.A. Terpening, and M.W. Bogue. 1988. Coeur d’Alene Basin EPA Water Quality Monitoring (1972-1986). EPA 910/9-88-216. PB89-217962. U.S. Environmental Protection Agency, Region 10, Seattle, WA. September.
Horowitz, A.J., K.A. Elrick, and R.B. Cook. 1993. Effect of mining and related activities on the sediment trace element geochemisty of Lake Coeur d’Alene, Idaho, USA. Part I: Surface Sediments. Hydrol. Process. 7:403-423.
Horowitz, A.J., K.A. Elrick, J.A. Robbins, and R.B. Cook. 1995. Effect of mining and related activities on the sediment trace element geochemistry of Lake Coeur d’Alene, Idaho, USA. Part II: Subsurface sediments. Hydrol. Process. 9(1):35-54.
Horowitz, A.J., K.A. Elrick, and R.B. Cook. 1999. Comment on “Phase associations and mobilization of iron and trace elements in Coeur d’Alene Lake, Idaho.” Environ. Sci. Technol. 33(1):201-202.
Houck, J.C., and L.L. Mink. 1994. Characterization of a Shallow Canyon Aquifer Contaminated by Mine Tailings and Suggestions for Constructed Wetlands Treatment. Prepared for the Trustees for the Idaho Natural Resources Damage Trust Fund. March 1994. 20 pp.
IDEQ (Idaho Department of Environmental Quality). 2004. Coeur d’Alene Lake Management Plan Update. Coeur d’Alene Regional Office, Idaho Department of Environmental Quality, Coeur d’Alene, ID. June 2004.
Johnson, A., D. Norton, B. Yake, and S. Twiss. 1990. Transboundary metal pollution of the Columbia River (Franklin D. Roosevelt Lake). Bull. Environ. Contam. Toxicol. 45(5): 703-710.
Kadlec, M. 2000. Ecological Risk Analysis of Elevated Metal Concentrations in the Spokane River, Washington. Contract C0000233. Prepared for the State of Washington Department of Ecology, Toxics Cleanup Program, Olympia, WA. November 2000.
Kimball, B.A. 1997. Use of Tracer Injections and Synoptic Sampling to Measure Metal Loading from Acid Mine Drainage. Fact Sheet FS-245-96. U.S. Department of the Interior, U.S. Geological Survey, Utah Water Science Center [online]. Available: http://ut.water.usgs.gov/usgsabout/fs245/FS_245_96.pdf [accessed May 12, 2005].
Kimball, B.A., R.L. Runkel, K. Walton-Day, and K.E. Bencala. 2002. Assessment of metal loads in watersheds affected by acid mine drainage by using tracer injection and synoptic sampling: Cement Creek, Colorado, USA. Appl. Geochem. 17(9):1183-1207.
Kuwabara, J.S., W.M. Berelson, L.S. Balistrieri, P.F. Woods, B.R. Topping, D.J. Steding, and D.P. Krabbenhoft. 2000. Benthic Flux of Metals and Nutrients into the Water Column of Lake Coeur d’Alene, Idaho: Report of an August, 1999, Pilot Study. U.S. Geological Survey Water-Resources Investigations Report 2000-4132. Denver, CO: U.S. Department of the Interior, U.S. Geological Survey [online]. Available: http://purl.access.gpo.gov/GPO/LPS7104 [accessed Feb. 9, 2005].
MFG (McCulley, Frick & Gilman, Inc.). 1995. Engineering Evaluation/Cost Analysis for the Canyon Creek Site. Prepared by MFG, Boulder, CO, for Trustees for the Natural Resource Damage Trust Fund. July 21, 1995 (as cited in URS Greiner and CH2Hill 2001c).
MFG (McCulley, Frick & Gilman, Inc.). 1998. 1997 Annual Groundwater Data Report, Woodland Park. Prepared by MFG, Osburn, ID, for Silver Valley Natural Resource Trustees. January 1998 (as cited in URS Greiner and CH2Hill 2001c).
Morrison, J.M., R.L. Hooper, J.B. Mahoney, and C.E. Rowe. 1999. Heavy metal partitioning in heavily contaminated lead/zinc mine tailings: What really happens during sequential extractions? Abstract No. 52066. Geological Society of America Abstracts with Programs (Annual Meeting, Oct. 24-29, Denver, CO). 31(7):A409.
NRC (National Research Council). 1999a. New Strategies for America’s Watersheds. Washington, DC: National Academy Press. 328 pp.
NRC (National Research Council). 1999b. New Directions in Water Resources Planning for the U.S. Army Corps of Engineers. Washington, DC: National Academy Press. 120 pp.
NRC (National Research Council). 2000. Clean Coastal Waters: Understanding and Reducing the Effects of Nutrient Pollution. Washington, DC: National Academy Press. 428 pp.
NRC (National Research Council). 2001. Compensating for Wetland Losses Under the Clean Water Act. Washington, DC: National Academy Press. 348 pp.
NRC (National Research Council). 2004. River Basins and Coastal Systems Planning Within the U.S. Army Corps of Engineers. Washington, DC: The National Academies Press.
Pelletier, G.J. 1994. Cadmium, Copper, Mercury, Lead, and Zinc in the Spokane River: Comparisons with Water Quality Standards and Recommendations for Total Maximum Daily Loads. Pub. No. 94-99. Olympia, WA: Washington State Department of Ecology, Environmental Investigations and Laboratory Services Program.
Plathe, K.L., R.L. Hooper, J.B. Mahoney, and L.A. Strumness. 2004. Arsenic metal speciation in mine contaminated lacustrine sediment using TEM/HR-ICPMS and calibrated sequential extraction. EOS Trans. AGU 85(17): Jt Assem. Suppl. Abstract H41E-05.
Ridolfi (Ridolfi Engineers and Associates, Inc.). 1998. Draft Restoration Plan Part A’s for the Coeur d’Alene Basin NRDA. Prepared for the Coeur d’Alene Tribe, U.S. Department of the Interior, U.S. Department of Agriculture, by Ridolfi Engineers and Associates, Inc., Seattle, WA. November 9, 1998 (as cited in URS Greiner, Inc. and CH2Hill 2001h).
Rowe, C.E., J.B. Mahoney, R.L. Hooper, and J.M. Morrison. 1999. Heavy metal partitioning and transport in the Coeur d’Alene River valley, northern Idaho. Abstract No. 52096. Geological Society of America Abstracts with Programs (Annual Meeting, Oct. 24-29, Denver, CO) 31(7):A410.
San Juan, C. 1994. Natural Background Soil Metals Concentrations in Washington State. Publication No. 94-115. Olympia, WA: Toxic Cleanup Program, Department of Ecology, Washington State. October 1994.
Spruill, T.B. 1993. Preliminary Evaluation of Hydrogeology and Ground-Water Quality in Valley Sediments in the Vicinity of Killarney Lake, Kootenai County Idaho. Water-Resources Investigations Report 93-4091. Boise, ID: U.S. Department of the Interior, U.S. Geological Survey. 41 pp.
Stratus Consulting. 2000. Report of Injury Assessment and Injury Determination: Coeur d’Alene Basin Natural Resource Damage Assessment. Prepared for U.S. Department of the Interior, Fish and Wildlife Service, U.S. Department of Agriculture, Forest Service and Coeur d’Alene Tribe, by Stratus Consulting Inc., Boulder, CO. September 2000.
Strumness, L.A., R.L. Hooper, and J.B. Mahoney. 2004. Contaminant pathways and metal sequestration patterns in the lower Coeur d’Alene River Valley, Idaho: Mechanics of trace metal mobility. EOS Trans. AGU 85(17), Jt. Assem. Suppl. Abstract No. H41E-04.
Tessier, A., P.G.C. Campbell, and M. Bisson. 1979. Sequential extraction procedure for the speciation of particulate trace metals. Anal. Chem. 51(7):844-851.
Thornburg, K.L., and R.L. Hooper. 2001. Transmission Electron Microscopy of Grain and Biocoatings from Lead and Zinc Contaminated Sediments in the Lower Coeur d’Alene River Valley. Abstract for Rocky Mountain and South-Central Sections, Geological Society of American Jooint Annual Meeting, April 29-May 2, 2001, Albuquerque, NM.
URS Greiner, Inc., and CH2M Hill. 1998a. Draft Technical Work Plan for the Bunker Hill Basin-Wade RI/FS, Panhandle Region of Idaho Including Benewah, Kootenai, and Shoshone Countries. Prepared for U.S. Environmental Protection Agency, Region 10, Seattle, WA, by URS Greiner, Inc., Seatlle, WA, and CH2M Hill, Bellevue, WA. June 12, 1998.
URS Greiner, Inc., and CH2M Hill. 1998b. Sediment Contamination in the Lower Coeur d’Alene River Basin (LCDARB): Geophysical and Sediment Coring Investigations in the River Channel, Lateral Lakes, and Floodplains. Bunker Hill Facility Basin-Wide RI/FS Data Report. Contract No. 68-W-98-228. Prepared for U.S. Environmental Protection Agency, Region 10, Seattle, WA, by URS Greiner, Inc., Seattle, WA, and CH2M Hill, Bellevue, WA. October 1998.
URS Greiner, Inc., and CH2M Hill. 2001a. Final (Revision 2) Remedial Investigation Report, Remedial Investigation Report for the Coeur d’Alene Basin Remedial Investigation/ Feasibility Study. URSG DCN 4162500.6659.05a. Prepared for U.S. Environmental Protection Agency, Region 10, Seattle, WA, by URS Greiner, Inc., Seattle, WA, and CH2M Hill, Bellevue, WA. September 2001.
URS Greiner, Inc., and CH2M Hill. 2001b. Final (Revision 2) Remedial Investigation Report, Remedial Investigation Report for the Coeur d’Alene Basin Remedial Investigation/ Feasibility Study, Vol. 1. Part 1. Setting and Methodology. URSG DCN 4162500. 6659.05a. Prepared for U.S. Environmental Protection Agency, Region 10, Seattle, WA, by URS Greiner, Inc., Seattle, WA, and CH2M Hill, Bellevue, WA. September 2001.
URS Greiner, Inc., and CH2M Hill. 2001c. Final (Revision 2) Remedial Investigation Report, Remedial Investigation Report for the Coeur d’Alene Basin Remedial Investigation/ Feasibility Study, Vol. 2. Part 2. CSM Unit 1, Canyon Creek Watershed. URSG DCN 4162500.6659.05a. Prepared for U.S. Environmental Protection Agency, Region 10, Seattle, WA, by URS Greiner, Inc., Seattle, WA, and CH2M Hill, Bellevue, WA. September 2001.
URS Greiner, Inc., and CH2M Hill. 2001d. Probabilistic Analysis of Post-Remediation Metal Loading Technical Memorandum (Revision 1). URSG DCN 4162500.06778.05.a. Prepared for U.S. Environmental Protection Agency, Region 10, Seattle, WA, by URS Greiner, Inc., Seattle, WA, and CH2M Hill, Bellevue, WA. September 20, 2001.
URS Greiner, Inc., and CH2M Hill. 2001e. Final (Revision 2) Remedial Investigation Report, Remedial Investigation Report for the Coeur d’Alene Basin Remedial Investigation/ Feasibility Study, Vol. 1. Part 7. Summary. URSG DCN 4162500.6659.05a. Prepared for U.S. Environmental Protection Agency, Region 10, Seattle, WA, by URS Greiner, Inc., Seattle, WA, and CH2M Hill, Bellevue, WA. September 2001.
URS Greiner, Inc., and CH2M Hill. 2001f. Final Technical Memorandum (Rev. 3) Estimation of Background Concentrations in Soil, Sediment, and Surface Water in the Coeur d’Alene and Spokane River Basins. URSG DCN 4162500.6790.05a. EPA Site File No. 2.7. Prepared for U.S. Environmental Protection Agency, Region 10, Seattle, WA, by URS Greiner, Inc., Seattle, WA, and CH2M Hill, Bellevue, WA. October 2001.
URS Greiner, Inc., and CH2M Hill. 2001g. Final (Revision 2) Remedial Investigation Report, Remedial Investigation Report for the Coeur d’Alene Basin Remedial Investigation/ Feasibility Study, Vol. 3. Part 2. CSM Unit 1, Upper Watersheds Ninemile Creek. URSG DCN 4162500.6659.05a. Prepared for U.S. Environmental Protection Agency, Region 10, Seattle, WA, by URS Greiner, Inc., Seattle, WA, and CH2M Hill, Bellevue, WA. September 2001.
URS Greiner, Inc., and CH2M Hill. 2001h. Final (Revision 2) Remedial Investigation Report, Remedial Investigation Report for the Coeur d’Alene Basin Remedial Investigation/ Feasibility Study, Vol. 2. Part 2. CSM Unit 1, Big Creek Watershed. URSG DCN 4162500.6659.05a. Prepared for U.S. Environmental Protection Agency, Region 10, Seattle, WA, by URS Greiner, Inc., Seattle, WA, and CH2M Hill, Bellevue, WA. September 2001.
URS Greiner, Inc., and CH2M Hill. 2001i. Final (Revision 2) Remedial Investigation Report, Remedial Investigation Report for the Coeur d’Alene Basin Remedial Investigation/ Feasibility Study, Vol. 4. Part 4. CSM Unit 3, Lower Coeur d’Alene River. URSG DCN 4162500.6659.05a. Prepared for U.S. Environmental Protection Agency, Region 10, Seattle, WA, by URS Greiner, Inc., Seattle, WA, and CH2M Hill, Bellevue, WA. September 2001.
URS Greiner, Inc., and CH2M Hill. 2001j. Final (Revision 2) Remedial Investigation Report, Remedial Investigation Report for the Coeur d’Alene Basin Remedial Investigation/ Feasibility Study, Vol. 3. Part 2. CSM Unit 1, Upper South Fork Watershed. URSG DCN 4162500.6659.05a. Prepared for U.S. Environmental Protection Agency, Region 10, Seattle, WA, by URS Greiner, Inc., Seattle, WA, and CH2M Hill, Bellevue, WA. September 2001.
URS Greiner, Inc., and CH2M Hill. 2001k. Final (Revision 2) Remedial Investigation Report, Remedial Investigation Report for the Coeur d’Alene Basin Remedial Investigation/ Feasibility Study, Vol. 4. Part 3. CSM Unit 2, Midgradient Watersheds, South Fork Coeur d’Alene River. URSG DCN 4162500.6659.05a. Prepared for U.S. Environmental Protection Agency, Region 10, Seattle, WA, by URS Greiner, Inc., Seattle, WA, and CH2M Hill, Bellevue, WA. September 2001.
URS Greiner, Inc., and CH2M Hill. 2001l. Final (Revision 2) Remedial Investigation Report, Remedial Investigation Report for the Coeur d’Alene Basin Remedial Investigation/ Feasibility Study, Vol. 4. Part 5. CSM Unit 4, Coeur d’Alene Lake. URSG DCN 4162500. 6659.05a. Prepared for U.S. Environmental Protection Agency, Region 10, Seattle, WA, by URS Greiner, Inc., Seattle, WA, and CH2M Hill, Bellevue, WA. September 2001.
URS Greiner, Inc., and CH2M Hill. 2001m. Final (Revision 2) Remedial Investigation Report, Remedial Investigation Report for the Coeur d’Alene Basin Remedial Investigation/ Feasibility Study, Vol. 4. Part 6. CSM Unit 5, Spokane River. URSG DCN 4162500. 6659.05a. Prepared for U.S. Environmental Protection Agency, Region 10, Seattle, WA, by URS Greiner, Inc., Seattle, WA, and CH2M Hill, Bellevue, WA. September 2001.
Villa, C.J. 2003. Superfund vs. Megasites: The Coeur d’Alene river basin story. Columbia J. Environ. Law 28(2):255-324.
Woods, P.F. 2001. Concentrations and Loads of Cadmium, Lead, Zinc, and Nutrients Measured During the 1999 Water Year Within the Spokane River Basin, Idaho and Washington. Open-File Report 00-441. Boise, ID: U.S. Department of the Interior, U.S. Geological Survey [online]. Available: http://purl.access.gpo.gov/GPO/LPS45894 [accessed Feb. 10, 2005].
Woods, P.F. 2004. Sediment and Lead Transport in the Coeur d’Alene River, Idaho. Presentation at the Third Meeting on Superfund Site Assessment and Remediation in the Coeur d’Alene River Basin, June 17, 2004, Coeur d’Alene, ID.
Woods, P.F., and M.A. Beckwith. 1997. Nutrient and Trace-Element Enrichment of Coeur d’Alene Lake, Idaho. U.S. Geological Survey Water-Supply Paper 2485. Washington, DC : U.S. Department of the Interior, U.S. Geological Survey. 93 pp.