1
Introduction
RADON AND LUNG CANCER: AN OVERVIEW
Radon-222, a noble gas resulting from the decay of naturally occurring uranium-238, was the first occupational respiratory carcinogen to be identified. As early as the 1500s, Agricola chronicled unusually high mortality from respiratory disease among underground metal miners in the Erz Mountains of eastern Europe (Hoover and Hoover 1950). In 1879, Harting and Hesse (1879) described autopsy findings that documented pulmonary malignancy in miners in that region and by early in the 20th century the malignancy was shown to be primary carcinoma of the lung (Arnstein 1913). The finding of high levels of radon in mines in the region and in the nearby mines of Joachimsthal in Czechoslovakia, where miners also had high lung-cancer rates, led to the hypothesis that radon was the cause of the lung-cancers (Ludwig and Lorenser 1924; Pirchan and Sikl 1932; also, see Jacobi 1994 and Proctor 1995 for a historical review). That hypothesis was not uniformly accepted until the findings of epidemiologic studies of other groups of radon-exposed underground miners were reported during the 1950s and 1960s (see Lorenz 1944 for an early view of the evidence and Proctor 1995 for a review of the controversy; NRC 1988 summarizes the epidemiologic evidence). Radon has now been classified as a human carcinogen by the International Agency for Research on Cancer (IARC 1988).
Radon is an alpha-particle emitter that decays with a half-life of 3.8 d into a short-lived series of progeny that have been referred to historically as radon daughters but are now more often termed radon-decay products or radon progeny (Figure 1-1). Radon itself was initially considered to be the direct cause of the lung-cancer in the miners. However, Harley proposed in his doctoral thesis at

FIGURE 1-1 The radon-decay chain. An arrow pointing downward indicates decay by alpha-particle emission; an arrow pointing to the right indicates decay by beta-particle emission. The historical symbols for the nuclides are in parentheses below the modern symbols. Most decay takes place along the unbranched chain marked with thick arrows. The negligible percentage of decay along the thin arrows is shown at critical points. The end of the chain, lead-206, is stable, not radioactive. Half-lives of each isotope are shown as seconds (s), minutes (m), days (d), or years (y). Modified from NRC (1988).
Rensselear Polytechnic Institute that it was the decay products of radon, and not radon, that delivered the pertinent dose to lung cells (Harley 1952, 1953, 1980). Bale learned of this thesis when he visited the Health and Safety Laboratory of the U.S. Atomic Energy Commission, where Harley had done his work, and he confirmed the calculations of lung dose from radon and thoron. Alpha particles released by 2 radioisotopes in the radon-decay chain, polonium-218 and polo-
nium-214, deliver to target cells in the respiratory epithelium the energy that is considered to cause radon-associated lung-cancer (NRC 1991).
Evidence on radon and is now available from about 20 epidemiologic studies of underground miners, including 11 studies that provided quantitative information on the exposure-response relationship between radon and lung-cancer risk (Lubin and others 1995). Those studies and several epidemiologic findings before them, continue to support the implementation of regulatory programs to reduce exposures of underground miners to radon and to provide compensation for occupational lung-cancer (Samet 1992).
Although the progeny of radon are now a well-recognized cause of lung-cancer, radon itself has again become a topic of controversy and public-health concern because it has been found to be a ubiquitous indoor air pollutant to which all persons are exposed (Cole 1993 and Proctor 1995 review the controversy). Radon was found to be present in indoor air as early as the 1950s, but the potential health implications received little attention until the late 1970s (Proctor 1995). In Scandinavia, housing surveys in the 1970s documented the presence of relatively high radon concentrations in homes built with materials containing medium-rich alum shale. Sparse data from the United States provided a similar indication of contamination of indoor air with radon. In 1984, a man triggered the radiation detector on entering the nuclear power plant where he worked. His home was found to have radon concentrations well beyond those permitted in underground mines. Other homes with high concentrations were identified later, and an enlarging database on indoor radon concentrations showed that the problem was widespread (Nero 1986).
The evidence on radon and lung-cancer is now extensive. Initially, research was driven by the need to characterize the risks faced by underground miners so that exposure limits that would keep risks to an acceptable level could be set. The work emphasized epidemiologic studies of the uranium and other underground miners exposed to radon, but animal studies were also conducted to address the modifying effects of such factors as the presence of ore dust and diesel exhaust, cigarette-smoking, and dose rate. Models of the respiratory tract were developed to characterize the relationship between exposure to radon progeny and dose of alpha energy delivered to target cells in the respiratory epithelium. In the last decade, research has reflected the need to improve the understanding of the risks posed to the general population by indoor radon. Epidemiologic studies have been conducted to assess the general population's risk of lung-cancer associated with indoor radon and complementary animal and laboratory studies have been carried out to address uncertainties in assessment of the risks associated with indoor radon. As a result, we have gained a rich body of evidence on radon and lung-cancer that addresses all facets of the problem within the framework of exposure, dose, and response (Figure 1-2). The new techniques of cellular and molecular biology also have brought new insights into how alpha particles injure the genetic material of cells and cause cancer (NRC 1994).

FIGURE 1-2 Factors influencing the relationship between radon exposure and lung-cancer risk. Modified from NRC (1991).
During the past 3 decades, a series of studies have been conducted within the operation of the National Academy of Sciences' National Research Council to evaluate the risks to human health following exposure to ionizing radiations. This series of risk assessments by committees on the Biological Effects of Ionizing Radiations, or BEIR, included a BEIR IV report in 1988 (NRC 1988) which focused on health effects of radon and other alpha-particle emitters.
The context for the work of the 6th Committee on the Biological Effects of Ionizing Radiations (BEIR VI), in the National Research Council's Board on Radiation Effects Research (BRER), has been set by concern about the risk posed to the public by indoor radon. As a basis for developing public policy on indoor radon, the risks associated with indoor radon across the range of residential exposures received by the population must be characterized. That range extends from exposures at the higher end comparable to those received by miners found to be at increased risk lung-cancer for down to exposures at an average indoor concentration of about 50 becquerels/cubic meter, or 50 Bqm-3 (1.4 picocuries/liter, or 1.4 pCiL-1), which are much lower than the exposures of most of the miners included in the epidemiologic studies. The degree of uncertainty in the risk estimates increases from the high end of the exposure range where risks are
directly measured, to the low end where risks must be extrapolated; the degree of uncertainty at the low end of the range has contributed to persistent questioning of the appropriateness of directing risk-management strategies uniformly across the full range of population exposures. In the United States, for example, the Environmental Protection Agency (EPA) has called for the voluntary measurement of indoor radon concentration in single-family residences and mitigation of radon in homes with average annual concentrations above 148 Bqm-3 (4 pCiL-1) (USEPA 1992c).
The BEIR VI committee thus faced the task of characterizing the risks associated with indoor radon across the full range of exposures and providing an indication of the uncertainty to be attached to risk estimates across that range. At the higher end of the range, exposures of the general population overlap those received by miners, and the extensive findings on risks in miners provide a reasonably accurate picture of the risks likely to be sustained by the population. At the lower end of the range, risks are estimated by extrapolating from the miner data and are consistent with the results of a meta-analysis of domestic-exposure studies (Lubin and Boice 1997). The extrapolation requires assumptions about the relationship between exposure to radon and lung-cancer risk and a careful exploration of the comparative doses from alpha particles delivered to the lung in the mining and indoor environments. The extrapolation also requires assumptions on the potential modifying effects of cigarette-smoking, age at exposure, and sex. These assumptions are a source of uncertainty in estimates of the risk of indoor radon, but choices can be supported by epidemiologic and experimental data. In preparing this report, the BEIR VI committee considered the entire body of evidence on radon and lung-cancer, integrating findings from epidemiologic studies with evidence from animal experiments and other lines of laboratory investigation.
Radon, of course, is only one of the causes of lung-cancer (Table 1-1). In fact, the epidemic of lung-cancer in the United States and many other countries largely reflects trends in cigarette-smoking, the dominant cause of lung-cancer (USDHHS 1989; Burns 1994; Mason 1994). Of the approximately 170,000 lung-cancer cases in 1996, most will be in cigarette smokers and thus avoidable in principle through smoking prevention and cessation. Synergism between radon and tobacco smoking implies heightened risks from radon in ever-smokers but cases caused by the joint effect of smoking and radon can be prevented by avoidance of smoking. Thus, risk projections of the number of lung-cancer cases attributable to radon should be interpreted with acknowledgment that most lung-cancer cases and deaths can be prevented by eliminating smoking.
PRIOR REPORTS ON THE RISK ASSOCIATED WITH RADON
There have been numerous assessments of the risks posed by radon, in both mining and indoor environments. Some have been generated by previous BEIR
TABLE 1-1 Risk factors for lung-cancer
• Active cigarette-smoking • Passive cigarette-smoking • Radon • Occupational carcinogens: |
Arsenic Asbestos Chromates Chloromethyl ethers Nickel |
• Polycyclic aromatic hydrocarbons • Family history • Fibrotic lung disorders • Ambient air pollution |
committees and others by committees of the National Council on Radiation Protection and Measurements (NCRP) and the International Commission on Radiological Protection (ICRP). The BEIR IV report (NRC 1988) reviewed the principal risk models published through the 1980s. Those models were based on either the dosimetric or the epidemiologic approach. In the dosimetric approach, a model is used to estimate the dose from alpha-particles delivered to the lung; the lung-cancer risk is then estimated with a risk coefficient based on risks in populations exposed to low linear-energy-transfer (low-LET) radiation, such as the Japanese atomic-bomb survivors, and adjusted for the greater potency of alpha radiation by using a quality factor for the relative biologic effectiveness (RBE) of alpha radiation in causing cancer, historically assumed to be the same as the ICRP quality factor of 20. The epidemiologic approach employs a risk model based on the studies of underground miners. The BEIR IV committee analyzed data from 4 cohorts of underground miners, using regression methods to develop a model that described the relationship between excess relative risk and exposure to radon progeny during 3 temporal periods of time since exposure. The model also incorporated an age dependence of the effect of radon on lung-cancer risk.
After the BEIR IV (NRC 1988) report, 2 new risk models were reported, both based on the epidemiologic approach. ICRP addressed exposures at home and at work in its Publication 65: Protection Against Radon-222 at Home and at Work (ICRP 1994a). That report used a model for excess relative risk that incorporated time dependence of the effect of radon; the risk coefficient was that reported by the BEIR IV committee. The 2nd model was based on an extensive pooled analysis of data on 11 cohorts of underground miners (Lubin and others 1995). The general analytic approach followed that of the BEIR IV committee. The models for excess relative risk incorporated exposure during windows defined by time since exposure and included age-dependence of the effect of radon. The rate at which exposure was received also had an important effect on lung-
cancer risk: risk increased as exposure rate decreased. Two models were described, one describing the exposure-rate effect by variables for the duration of exposure and the other by variables for exposure rate.
Early in its deliberations, the committee critically assessed the various possible approaches to risk estimation from exposure to low levels of radon. The possible methodologies for generating such risk estimates are summarized in Figure 1-3, and are discussed in chapter 3. In addition to the previously used dosimetric and epidemiologic approaches, statistical models can also be applied that are based on a biologic construct of the effect of radon.
The dosimetric approach focuses on computing the doses deposited in lung target cells by alpha particles emitted from radon progeny deposited in various compartments of the lung. The approach relies on applying risk estimates from

FIGURE 1-3 Possible methodologies for generating risk estimates for low exposures to radon.
populations exposed to other radiations, such as from the atomic-bomb survivors in Hiroshima and Nagasaki who were exposed to primarily gamma rays and neutrons, to the doses estimated in the lung cells which are believed to become lung-cancers.
The biologically-motivated approach would use data from molecular, cellular, and animal studies to generate parameters which could be used to refine models of carcinogenesis. The miner data could be used in conjunction with the models to see if effects observed in nonhuman systems might be altered by responses in the intact human.
Finally, the empirical approach would use the state-of-the-art statistical methodologies to analyze epidemiologic data from miner and residential studies. In brief, of the three data sources (miner studies, domestic radon exposure studies, and populations exposed to γ rays), use of data from γ-ray exposed groups (particularly A-bomb survivors) was rejected due to the many assumptions needed to generate risk estimates for prolonged localized exposure to densely ionizing radiation, based on risks for acute whole-body exposures to γ rays. Use of data from studies of residential radon exposure was rejected for the primary risk estimation due to the very limited statistical power available in these studies. However, the residential data were used as validation and support for the low-dose risk estimates, in that the results were compared with, and shown to be consistent with, risk estimates extrapolated from analyses of the miner data.
Of the three modeling approaches possible for analyzing the epidemiologic data (dosimetric approach, biologically-motivated models, empirical approach), the dosimetric approach was rejected for the same reasons as was the use of A-bomb survivor data—the uncertainties involved in extrapolating from the effects of acute whole-body γ-ray exposures to prolonged localized exposure to densely ionizing radiation. The use of biologically-motivated models—such as the two-stage clonal expansion model—was rejected due to our limited knowledge of the complete mechanisms involved in radon-induced carcinogenesis. As described in chapters 2 and 3, however, mechanistic information was used to guide specific parts of the empirical modeling, whenever possible.
In summary, the committee chose to follow the overall methodology both of BEIR IV and the pooled analysis of Lubin and others (1994a), and to base risk estimates on an empirically-based analysis of miner data. This approach provided the committee with the invaluable starting point of existing data bases and methodologies, some well-characterized and others with deficiencies and limitations as described in chapter 3.
POPULATION EXPOSURE TO RADON
The principal place of exposure to radon is the home; the predominant contribution of exposure in the home reflects the amount of time spent there and the general pattern of radon concentrations in buildings (Harley 1991; NCRP 1991;
Samet and Spengler 1991). The major source of the radon in a home is the soil gas that enters from beneath and around the structure (NCRP 1984). Radon-contaminated water and radium-rich building materials can also contribute radon. Under some circumstances, water can contribute a substantial amount of the radon in air, but water is a relatively minor source of population exposure to radon progeny.
Surveys of indoor radon concentrations in the United States and many other countries have shown that radon is ubiquitous indoors, typically at a concentration only one hundredth to one-tenth that found in the underground mines and shown to be associated with lung-cancer. For the survey conducted by the U.S. Environmental Protection Agency, the distribution of concentrations is roughly lognormal; the arithmetic mean concentration is about 46.25 Bqm-3 (1.25 pCiL-1) (Figure 1-4). On the basis of the survey information, mean exposure of the general population is about 0.0007 Jhm-3 (0.2 WLM) per year or about 0.049 Jhm-3 (14 WLM) in a lifetime (NCRP 1984, 1991).
THE COMMITTEE'S APPROACH
The BEIR VI committee was constituted after a BEIR VI phase 1 committee determined that sufficient evidence had become available since the 1988 publication of the BEIR IV report to justify a new study (NRC 1994). The charge extended to the BEIR VI committee was broad (Table 1-2). Its principal goals were to examine evidence of effects of low-level exposure to radon progeny and to develop a mathematical model for the lung-cancer risk associated with radon. In developing this model, the committee was to address key uncertainties, including the combined effect of smoking and radon, the effect of exposure rate, the effects of exposures to agents other than radon in the mines, and the consequences of errors in exposure estimates. The committee was also asked to review relevant evidence from radiobiologic studies, to reassess exposure-dose relations, and to evaluate the potential utility of the case-control studies of indoor radon.
The multidisciplinary BEIR VI phase 2 committee worked in topic-oriented groups that addressed exposure and dosimetry; molecular and cellular aspects of radon carcinogenesis, including the findings of in vitro approaches and animal studies; epidemiologic studies of miners; case-control studies in the general population; and risk modeling. When the committee began its work, it was recognized that the data on the 11 cohorts of miners would be essential for the development of a new risk model; the committee obtained the cooperation of the principal investigators for the individual cohort studies so that additional analyses of the data could be undertaken to develop a risk model. During the course of deliberations by the committee, it became apparent that a new meta-analysis of exposure in homes was powerful enough to yield useful risk estimates associated with domestic exposures.
The committee also recognized that its work could not be artificially sepa-
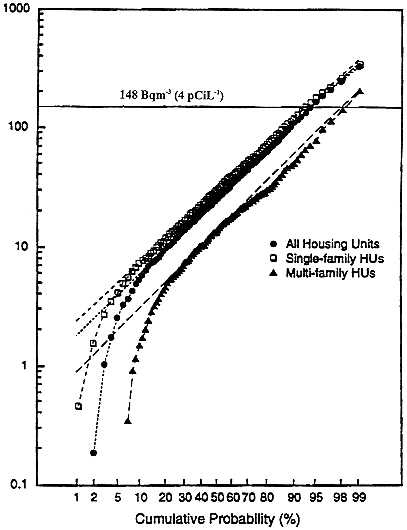
FIGURE 1-4 Distribution of radon concentrations in U.S. homes (modified from the National Residential Radon Survey; USEPA 1994).
rated from the context set by controversy and debate over the management of the risk posed by indoor radon. In the United States, the EPA has pursued a broad national strategy to reduce radon risks through voluntary testing of homes and mitigation of radon in those with an annual average above 148 Bqm-3 (4 pCiL-1). EPA finds a basis for risk management in its projections of 7,000-30,000 lung-cancer deaths per year attributable to residential radon exposure (Page 1993).
TABLE 1-2 Charge to the BEIR VI committee
Radiation Biology and Carcinogenesis |
• Evaluate experimental evidence on inverse dose-rate relations and its implications. • Attempt to correlate risks of radon-induced lung tumors with those of tumors induced by external exposure to low-LET radiation. • Examine in more detail the induction and repair of molecular changes after exposure to alpha particles. |
Exposure-Dose Relations |
• Gain access to additional information that is relevant to radon dosimetry from the use of noninvasive methods for monitoring ventilation and from an improved model. • Use activity-weighted size-distribution data that have become available on residences. • Use the recent data on growth of particles from various sources typically found in homes. • Use biologic dosimetry to reduce the uncertainties associated with the exposure-dose relationship and apply the reduced uncertainties in various aspects of lung dosimetry to risk calculations. |
Studies of Miners |
• Critique the recently completed analyses of pooled data on 11 cohorts and whatever new risk models emerge from them and use the database to suggest additional analyses. • Model the pooled data with emphasis on biologically-driven modeling. • Formally evaluate sources of exposure error in the miner cohorts and the consequences of those errors for risk estimation and risk assessment. |
Studies of Lung Cancer in the General Population |
• Evaluate and interpret the results of completed case-control and ecologic studies, including evaluation of their limitations and uncertainties. • Determine the appropriate role of case-control studies in developing a risk model of residential exposure to radon. • Make general recommendations regarding the potential role of case-control studies that are not yet complete. • Estimate the statistical power of the completed studies for various end points as a group and identify the expected upper and lower confidence limits according to the assumptions of the recommended model and alternative models. • Estimate the potential of the completed studies for providing information on the modifying effects of smoking and other factors, taking into account uncertainties in exposure estimates. • Make recommendations on the desirability of initiating new case-control or ecologic studies of residential radon exposure. |
Modifications to the Phase 1 Recommendations |
• Assess the validity and scientific reliability of analytic approaches used by various investigators. • Examine in detail the interaction between radon exposure and cigarette-smoking on the basis of data on US and other miners. • Reexamine the effect of the rate of exposure to radon on the incidence of lung-cancer. • Critically examine exposure estimates for miner cohorts and reassess the consequences of the exposure rates. • Propose, in light of the phase 1 findings, a mathematical model of risk based on more complete and up-to-date U.S. and international miner data. |
• Assess the role of arsenic, silica, and other contaminants in mines on the consequences of exposure. • Examine the uncertainties associated with the miner studies. |
Analysis of Data from Studies of Residential Exposure |
• Critically review studies and comment on their strengths and weaknesses and their current and future roles in risk assessment. • If EPA and BRER agree that is feasible, update the assumptions and estimates in the Research Council report that compared miner and home dosimetry, focusing on recent data on such physical and biologic factors as aerosol size distribution, ultrafine fraction, equilibrium fraction, and hygroscopicity and deposition of radon daughters in the respiratory tract. • Test and possibly revise models in light of available residential data. • Consider the contribution of radon-220 to risk in mines and homes. • Examine the effects of age, sex, and smoking on radon-associated risk. • Incorporate concepts from cellular and molecular biology into models for risk assessment. |
Most of those projected deaths are attributable to the lower range of exposures, well below the exposures and exposure rates sustained by the miners, so there is substantial uncertainty as to the total burden of lung-cancer resulting from indoor radon. The agency's action guideline of 148 Bqm-3 (4 pCiL-1) is also in this range, as are the action levels set by other countries. The BEIR VI committee therefore characterized the certainty that could be attached to risk estimates made with its model, moving from a high level of confidence in estimates at exposures comparable with those received by the underground miners to a substantially lower degree of confidence in estimates related to typical indoor exposures.
CRITICAL ISSUES
The BEIR VI committee identified 6 issues deemed critical in characterizing the risks associated with indoor radon. Each deals with a point of uncertainty in formulating a risk model and estimating the risk posed to a population by indoor radon. In the review of the evidence, those critical issues served as points of synthesis for identifying the limits of current evidence for risk assessment. The issues are briefly described here.
Extrapolation from Higher to Lower Exposures
Early in its work, the committee recognized that the evidence on quantitative risks from studies of underground miners would remain the principal basis for estimating the risk associated with indoor radon. Most of the evidence from the
miners is based on cumulative exposures at least 10 times the estimates of typical lifetime residential exposure, that is, about 0.049 Jhm-3 (14 WLM) (NCRP 1984). Consequently, extension of risk models based on data from miners to the general population rests on extrapolation below the exposure range of most of the miner data. A linear-nonthreshold model has been assumed for this purpose by other groups developing radon risk models, including the BEIR IV committee (NRC 1988). Risk projections are sensitive to the extrapolation model chosen. For example, a model that incorporated a threshold at lower levels of exposure, such as, below the mean of about 0.0035 Jhm-3 (1 WLM), would project far fewer radon-caused lung-cancers than a nonthreshold model, because of the distribution of population exposures (Figure 1-4).
Extrapolation from Higher to Lower Exposure Rates
The underground miners included in the epidemiologic studies typically received their occupational exposures to radon over several years (Lubin and others 1994). However, exposure to indoor radon takes place across the full lifetime, so exposure rates are generally far lower for the radon exposures received by the general population than for the exposures received by the miners in the epidemiologic studies. The analysis of pooled data on 11 cohorts of underground miners indicated an inverse exposure-rate effect; that is, the effect of exposure increased as the rate at which the exposure was received diminished (Lubin and others 1994). Laboratory systems have shown a parallel inverse dose-rate effect for some end points (NRC 1994). The BEIR VI phase 1 committee recognized that the full BEIR VI committee would need to address the extrapolation of exposure-rate effects for exposures received by the miners to those typically received by the general population.
Interactions of Radon Progeny with Other Agents
Lung-cancer can be caused by a number of inhaled environmental agents, including tobacco smoke (Table 1-1) (Lubin and others 1995). Agents in the mining environment other than radon progeny have been associated with lung-cancer, including diesel exhaust, arsenic, and silica. Those agents might potentially confound the relationship between radon progeny and lung-cancer or modify the risk associated with radon progeny. The majority of the miners included in the epidemiologic studies also were probably cigarette smokers, although the information on smoking in the various cohorts is incomplete (Lubin and others 1994). Exposure to radon progeny is an established cause of lung-cancer in those who have never smoked (NRC 1988), but the risks to ever-smokers and never-smokers have not been separately characterized with great precision. Lack of information on the combined effect of smoking and radon introduces uncertainty in extending a risk model based on data from miners to the general population.
Interpretation of risk projections for the general population is further clouded by synergism between smoking and radon progeny: some lung-cancer cases reflect the joint effect of the two agents and are in principle preventable by removing either agent.
Susceptibility
The development of lung-cancer is considered to be a multistep process involving a sequence of genetic changes that ultimately results in malignant transformation. A rapidly growing body of literature describes some of these changes, for example, in tumor-suppressor genes and oncogenes (Economou and others 1994). There are many points in this sequence, from carcinogen metabolism to DNA repair, at which genetic susceptibility might influence lung-cancer risk. In fact, there is descriptive evidence from epidemiologic studies that a family history of lung-cancer is associated with increased risk (Economou and others 1994). The evidence has not addressed radon progeny specifically, but it was deemed sufficiently compelling by the BEIR VI committee to warrant its being considered as one of the critical issues. In particular, the committee considered the implications of identifying population groups at risk for lung-cancer generally or for lung-cancer caused by radon progeny specifically.
Links Between Biologic Evidence and Risk Models
The BEIR VI phase 1 committee recognized that key sources of uncertainty listed above as the first 4 critical issues would be best addressed by risk models that directly reflect current biologic understanding directly and are not simply empiric and based on analysis of observational data. The assumptions to be made with regard to the relationship between exposure and risk and with regard to the inverse exposure-rate effect were judged to be particularly critical. Consequently, the phase 2 committee was constituted to include the full range of scientific disciplines concerned with radon carcinogenesis, and a subcommittee, the Molecular and Cellular Working Group, synthesized the evidence with a goal of providing guidance to a second subcommittee, the Risk Modeling Working Group.
Signatures of Radon Effects
Lung-cancer constitutes a clinically and histologically heterogeneous group of malignancies. There are 4 principal histologic types: squamous cell carcinoma, adenocarcinoma, small cell carcinoma, and large cell carcinoma. Each has somewhat different clinical characteristics. In addition, substantial heterogeneity at the molecular level has been demonstrated with markers of genetic change. This heterogeneity, initially at the light-microscopy level and now at the genetic
level, has motivated investigations to determine whether specific lung-cancers can be linked to radon; that is, is there a ''signature" that identifies a particular cancer as caused by radon? Demonstration of a signature would add specificity to risk assessments and even permit the designation of lung-cancers in individuals as caused by radon or some other factor. The committee reviewed the literature for evidence of signatures of radon-related lung-cancer
OVERVIEW OF COMMITTEE RISK ASSESSMENT
The committee's approach to assessing the risks associated with indoor radon had multiple components. Analysis of the miner data supplied a model describing the relationship between cumulative exposure to radon and lung-cancer risk; that model development is described in chapter 3 and appendix A. The greatest proportion of the population's exposure to radon is at the lower end of the distribution of residential concentrations (Figure 1-4), a distribution which results in exposures across the lifespan substantially lower than the cumulative exposures received by most of the miners in the epidemiologic studies.
As a basis for selecting the preferred exposure-response model, the committee reviewed relevant biologic information on mechanisms of DNA damage by alpha particles and the dosimetry of alpha particles at exposures relevant to environmental exposures. This review, described in chapter 2, led to the conclusion that, at typical indoor exposure levels and over a typical lifetime, target-cell locations in the respiratory tract would be traversed by no more than a single alpha particle. Even allowing for DNA repair and cellular lethality, such a single alpha-particle traversal is known to produce, with significant probability, large-scale damage to the DNA in surviving traversed cells. Thus, at low exposure levels, a further decrease in the exposure would result in a proportionate decrease in the number of target cells traversed by single alpha particles, and thus suffering large scale DNA damage. Each of these heavily damaged traversed cells would still be expected to show the same probability of initiating the sequence of events that ultimately lead to carcinogenesis. Taken together, these observations provide a rationale for the committee's assumption that the dose-response relationship for radon-induced lung-cancer is likely to be best described by a linear model with no threshold in dose.
The committee noted, as discussed elsewhere in this report, that statistical considerations preclude a direct investigation of low-dose thresholds for radon-induced carcinogenesis, at least in human populations. However, the view of the committee was that the mechanistic and experimental considerations were sufficiently compelling to support assumption of a linear/nonthreshold dose-response relationship at low radon exposures. It is also important to note that the linear/nonthreshold approach which the committee has adopted is based on mechanistic considerations relating to the nature of alpha-particle induced energy deposition
and damage; whether these considerations also hold for other carcinogens, such as x rays, was not an issue that was addressed by the committee.
In developing its risk model, the committee conducted analyses of the full miner data set and additional analyses limited to the exposure range of < 0.35 Jhm-3 (less than 100 WLM) and focused on < 0.175 Jhm-3 (less than 50 WLM) which is an exposure range involving less than one alpha-particle traversal per cell nucleus location depending on the assumed target cell. An exposure of 0.35 Jhm-3 (100 WLM) results in about 0.84 traversals for basal cell nuclei, about 1.88 traversals for bronchiolar secretory-cell nuclei, and about 3.25 traversals per bronchial secretory-cell nuclei. Additional details regarding alpha-particle traversals of basal- and secretory-cell nuclei can be found in Harley and others (1996) who used a large data base of bronchial-cell nuclei morphometry. The committee's preferred risk model describes lung-cancer risk with a linear nonthreshold relationship between exposure and risk. The model describes risk as time-dependent and age-dependent.
When the risk model is applied to the general population, possible differences between miners and the general population need consideration; that is, similar exposures to radon progeny in homes and miners may not result in the same doses of alpha energy for target cells. Following the approach of the BEIR IV committee, the BEIR VI committee used a mathematical model of the lung to calculate doses to target cells received by miners and by the general population for a given exposure. Those doses were used to determine whether an adjustment was needed in extending risks from miners to the general population.
The committee's model describes the increase in the relative risk of lung-cancer associated with exposure to radon progeny. To project the lung-cancer risks associated with exposure to indoor radon, the committee needed to assume a background rate of lung-cancer mortality. Assumptions will also be needed to extend the model to the full lifespan, to women, and to ever-smokers and never-smokers. The bases for these assumptions and associated uncertainties are described in the report.