10
Teaching to Promote the Development of Scientific Knowledge and Reasoning About Light at the Elementary School Level
Shirley J. Magnusson and Annemarie Sullivan Palincsar
Children at play outside or with unfamiliar materials look as though they might be answering such questions as: What does this do? How does this work? What does this feel like? What can I do with it? Why did that happen? This natural curiosity and exploration of the world around them have led some people to refer to children as “natural” scientists. Certainly these are the very types of questions that scientists pursue. Yet children are not scientists. Curiosity about how the world works makes engaging children in science relatively easy, and their proclivity to observe and reason (see Chapter 1, Box 1-1) is a powerful tool that children bring to the science classroom. But there is a great deal of difference between the casual observation and reasoning children engage in and the more disciplined efforts of scientists.
How do we help students develop scientific ideas and ways of knowing?1 Introducing children to the culture of science—its types of reasoning, tools of observation and measurement, and standards of evidence, as well as the values and beliefs underlying the production of scientific knowledge—is a major instructional challenge. Yet our work and that of others suggest that children are able to take on these learning challenges successfully even in the earliest elementary grades.2
THE STUDY OF LIGHT
Unlike mathematics, in which topics such as whole-number arithmetic are foundational for the study of rational number, and both are foundational for the study of functions, there is currently little agreement on the selection and sequencing of specific topics in science, particularly at the elementary level.3 What clearly is foundational for later science study, however, is learning what it means to engage in scientific inquiry—learning the difference between casual and scientific investigations. That learning can be accomplished in the context of many different specific topics.
In this chapter, we choose light as our topic of focus because it affords several benefits. The first is practical: the topic involves relatively simple concepts that children can understand from investigating with relatively simple materials. For example, our bodies and the sun make shadows that can be studied, and similar studies can occur with common flashlights and classroom materials. Pencil and paper, and perhaps some means of measuring distance, are all that is needed for data collection. Children can also study light using simple light boxes (Elementary Science Study’s Optics unit4) in which light bulbs are placed in cardboard boxes containing openings covered with construction paper masks that control the amount of light emanating from the box. Thin slits in the masks make the thin beams of light necessary for studies of reflection and refraction. Multiple wider openings covered with different colored cellophane filters enable investigations mixing colors of light. And again, pencil and paper are all that are needed for data collection showing the paths of light.
In addition, developing scientific knowledge of light challenges us to conceptualize aspects of the world that we do not directly experience—a critical element of much scientific study. For example, light travels, yet we do not see it do so; we infer its travel when we turn on a flashlight in the dark and see a lighted spot across the room.
Developing scientific knowledge often requires conceptual change5 in which we come to view the physical world in new ways.6 Students must learn that things are not always what they seem—itself a major conceptual leap. The study of light gives children an accessible opportunity to see the world differently and to challenge their existing conceptions. We see the world around us because light reflects from objects to our eyes, and yet we do not sense that what we see is the result of reflected light.
Some children, moreover, view shadows as objects instead of understanding that shadows are created when light is blocked. Conceptual development is required if they are to understand the relationship among a light source, an object, and the shadow cast by that object. Working with flashlights can provide children an opportunity to challenge directly everyday conceptions about shadows, providing them with a powerful early experi-
ence of scientific ways of knowing. Because casual observation of the behavior of light can be misleading, but a relatively accessible investigation of light can be illuminating, the study of light demonstrates the contrast between casual observation and experimentation. For all these reasons, then, the study of light supports children’s understanding that relationships in the physical world are not self-evident and that constructing scientific knowledge from observation of the world is different from their everyday reasoning.
Three major instructional challenges parallel the principles of How People Learn as they apply to the study of light: (1) providing students with opportunities to develop deep conceptual understanding of targeted aspects of light, and of standards and norms in science for investigating and drawing conclusions (both about light and more generally); (2) supporting students in building or bridging from prior knowledge and experience to scientific concepts; and (3) encouraging children to engage in the kind of metacognitive questioning of their own thinking that is requisite to scientific practice.
Conceptual Understanding
How People Learn suggests that learning for understanding requires the organization of knowledge around core concepts. Thus while light can be studied with tools that are easy to use and opportunities to observe the behavior of light abound, if the classroom activity described in this chapter were simply a set of experiences and observations, it would leave students with little deep knowledge. Experiencing many individual activities (e.g., seeing that light reflects from wood as well as mirrors) does not ensure that students understand the overarching concepts about light outlined below that allow them to predict how light will behave in a wide variety of circumstances. As a result, a major focus in this chapter is on the role of the teacher in guiding students’ observations, reasoning, and understanding so that core concepts are grasped.
What conceptual understandings do we consider to be core? As suggested above, grasping the differences between everyday observations and reasoning and those of science is not only core in our approach to teaching about light, but also paramount in providing a foundation for further science study. Salient concepts include the following:
-
Standards of the scientific community for understanding and communicating ideas and explanations about how the world works are different from everyday standards. Science requires careful observations that are recorded accurately and precisely, and organized so that patterns can be observed in the data.
-
Patterns in observations are stated as knowledge claims.
-
Claims are judged on the quality of the evidence supporting or disconfirming them.
-
Hypotheses take on the status of claims only after they have been tested.
-
Claims are subject to challenge and not considered new scientific knowledge until the scientific community accepts them.
These understandings are foundational for all future study of science.
There are also core concepts regarding the topic of light that we want students to master. These will vary somewhat, however, according to the grade level and the amount of time that will be devoted to the topic. These concepts include the following:
-
All objects (experienced in our everyday lives) reflect and absorb light, and some objects also transmit light.
-
Dark or black objects mainly absorb light; light or white objects mainly reflect light.
-
There is an inverse relationship between light reflected from and absorbed by an object: more reflected light means less absorbed light.
-
-
Light reflects from objects in a particular way: the angle of incoming light equals the angle of reflected light.
-
What we see is light reflected from objects.
-
There must be a source of light for us to see an object.
-
Sources of illumination can produce light (e.g., the sun) or reflect light (e.g., the moon).
-
-
When an object blocks a source of light, a shadow is formed. Shadows are dark because there is no light reaching them to be reflected to our eyes. The distance of an object from a source of light it blocks determines the size of the object’s shadow. The shape of an object’s shadow depends on the angle of the object to the light, so the shadow of an object may have more than one shape.
-
The color of an object is the color of light reflected from the object.
-
The colors of light come from white light, which can be separated into many colors.
-
The color of an object depends on the extent to which particular colors of light in white light are reflected and absorbed.
-
Other concepts—such as the nature of light as both a wave and a particle—are beyond what elementary students need to understand. But teachers need to know these core concepts to deal effectively with questions that may arise, as we discuss later in this chapter.
Prior Knowledge
Students bring many prior conceptions about light to the classroom. Some of these are influenced relatively easily. For example, some students believe a shadow is an object, but this conception is not deeply held, and simple experiments with light can provide convincing evidence to the contrary. Other scientifically inaccurate conceptions are not so easily changed by simple experiments.
A very common belief is that light reflects only from shiny objects, such as a mirror or shiny metals. This is hardly surprising; reflections from shiny objects are strikingly obvious, while observing reflection from objects with no apparent shine requires a tool (e.g., a simple device such as a piece of paper strategically placed to show reflected light, or a more sophisticated device such as an electronic meter that measures light energy). In fact, the nature of light has puzzled scientists for centuries.7 Part of the challenge to our understanding is that the behaviors and effects of light are not easily determined by our senses. Light travels too fast for us to see it traveling, and our observation of light that has traveled great distances, such as light from the sun and other stars, provides no direct evidence of the time it has taken to reach us. Scientists have determined that light exerts pressure, but this is not something we can feel. We see because light is reflected to our eyes, but we have no way of experiencing that directly. We commonly think of color as an intrinsic characteristic of an object because we do not experience what actually occurs: that the color we see is the color of light reflected from the object. Furthermore, grasping this notion requires understanding that white light is made up of colors of light that are differentially absorbed and reflected by objects. If none are reflected, we see black, and if all are reflected, we see white, and this is counter to our experience with colored pigments that make a dark color when mixed together. Finally, perhaps the strongest testimony to the complex nature of light is the fact that scientists use two very different models to characterize light: a particle and a wave.
Because daily experience reinforces ideas that may be quite different from scientific understanding, fostering conceptual change requires supporting students in paying close attention to how they reason from what they observe. For this reason, the approach to teaching we suggest in this chapter provides students with a great many opportunities to make and test knowledge claims, and to examine the adequacy of their own and others’ reasoning in doing so. Once again, however, the role of the teacher is critical. As we will see, the prior conceptions with which students work may lead them to simply not notice, quickly dismiss, or not believe what they do not expect to see.
Metacognition
Young children, and indeed many adults, assume that things are as they appear, and no further questioning is required. That light reflects off objects only if they are shiny may appear to be true and in no need of further questioning. Science, however, is about questioning—even when something seems obvious—because explanation is at the heart of scientific activity. Thus the search for an explanation for why shiny objects reflect light must include an answer to the question of why nonshiny objects do not. Such a search, of course, would lead to evidence refuting the notion that only shiny objects reflect light. Engaging children in science, then, means engaging them in a whole new approach to questioning. Indeed, it means asking them to question in ways most of us do not in daily life. It means questioning the typical assurance we feel from evidence that confirms our prior beliefs, and asking in what ways the evidence is incomplete and may be countered by additional evidence. To develop thinking in this way is a major instructional challenge for science teaching.
THE STUDY OF LIGHT THROUGH INQUIRY
With the above principles in mind, we turn now to the learning of science through investigative activity in the classroom, or inquiry-based instruction.8 Investigations in which students directly observe phenomena, we believe, serve several critical functions. First, when students experiment with light and observe phenomena they do not expect, these discrepant experiences can directly challenge their inaccurate or partially developed conceptions. Students will need many opportunities to observe and discuss the behavior of light that behaves in unexpected ways if they are to develop scientific conceptions of light. Inquiry that is designed to occur over weeks and allows students to work with many different materials can provide that experience. The opportunity for repeated cycles of investigation allows students to ask the same questions in new contexts and new questions in increasingly understood contexts as they work to bring their understanding of the world in line with what scientists think. Equally important, participation in well-designed guided-inquiry instruction provides students with a first-hand experience of the norms of conducting scientific investigation.
But inquiry is a time- and resource-intensive activity, and student investigations do not always lead to observations and experiences that support the targeted knowledge. Therefore, we combine first-hand investigations with second-hand investigations in which students work with the notebook of a fictitious scientist to see where her inquiry, supported by more sophisticated tools, led. This second-hand inquiry provides a common investigative experience that allows the teacher to direct attention to steps in the
reasoning process pursued by the scientist that led to the development of core concepts. Moreover, it allows students to see that while scientists engage in a similar type of inquiry, more sophisticated tools, more control over conditions, and larger sample sizes are critical to drawing conclusions that can be generalized with some confidence.
A Heuristic for Teaching and Learning Science Through Guided Inquiry
To aid our discussion of the unfolding of instruction, we present a heuristic—a thinking tool—to support planning, enacting, and evaluating guided-inquiry instruction with elementary school teachers.9 This heuristic (see Figure 10-110), which shares many features with other researched-based approaches to teaching elementary science through investigation,11 represents instruction in terms of cycles with phases. The words in all capital letters in Figure 10-1 indicate the phases, and the lines with arrows show the progression from one phase to the next. Reporting is a key phase in this conception of instruction; it is the occasion when groups of students report the results of their investigations to their classmates. Students are expected to report on knowledge claims they feel confident in making and providing evidence for those claims from the data they collected during investigation. This expectation lends accountability to students’ investigative activity that is often absent when they are simply expected to observe phenomena. To make a claim, students will need precise and accurate data, and to have a
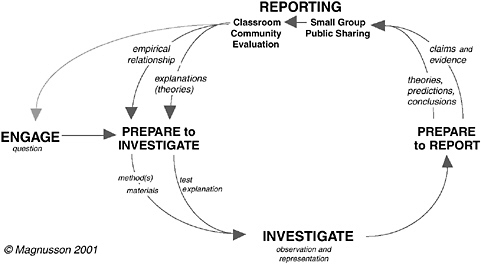
FIGURE 10-1 A cycle of investigation in guided-inquiry science.
claim that is meaningful to the class, they will need to understand the relationship between the question that prompted investigation and the way in which their investigation has enabled them to come up with an answer.
Multiple lines leading from one phase to another indicate the two basic emphases of investigative activity in science: generating knowledge that describes how the world works (outer loop), and generating and testing theories to explain those relationships (inner loop). The reporting phase always marks the end of a cycle of inquiry, at which point a decision is made about whether to engage in another cycle with the same question and investigative context, or to re-engage with a novel investigative context or a new question. Cycles focused on developing knowledge claims about empirical relationships generally precede cycles in the same topic area focused on developing explanations for those relationships. Thinking and discussing explanations may occur in other cycles, but the focus of the cycle represented by the inner loop is on testing explanations.
Each phase in the heuristic presents different learning opportunities and teaching challenges. Each also provides opportunities to focus on ideas describing the physical world (concepts and theories or content) as well as the means by which we systematically explore the nature of the physical world (methods and reasoning or process).
Each phase requires different types of thinking and activity on the part of the students and the teacher; hence, each has a unique role to play in supporting the development of scientific knowledge and ways of knowing. The following illustrations of teacher and student activity in each phase of instruction are drawn from our work in elementary school classrooms.12
The Engage Phase
Description. Each unit of study begins with an engagement phase, which orients thinking and learning in a particular direction. In the elementary classroom, a version of the classic KWL (i.e., what do I Know, what do I Want to learn, what have I Learned) can be a fine way to initiate engagement. In contrast to the typical use of KWL in the language arts, however, to maximize the value of having students identify what they know, teachers should invite students to identify how they have come to know the topic area. Doing so can develop students’ awareness that “knowing” can mean different things. Does their knowledge arise from something they actually observed? If so, where and when did that occur, and under what circumstances? Or did others observe it and report it to them? If so, how confident were they in what was reported and why? If a student reports knowledge from something written in a book, what other information was provided? Were any data provided to substantiate the claim? How extensive was the information provided regarding what the student reports knowing? This dis-
cussion can provide the grist for later comparisons of ways of knowing in everyday life versus in science, history, or the language arts. It also affords teachers an opportunity to draw out and learn about students’ prior knowledge, metacognitive awareness, and reasoning abilities. For example, in a class beginning to investigate how light interacts with matter, one student stated that he already knew the answer because he knew that objects were opaque, transparent, or translucent. This statement indicated to the teacher that the student might assume light interacts with an object in only one way, which could limit what he observed. Knowing of this possibility, the teacher would want to monitor for it, and possibly raise questions about the thoroughness of students’ observations.
The scientific community defines for itself what knowing in particular ways means. For example, in each discipline (e.g., physics, chemistry, biology), the community defines what are acceptable methods for data collection and what constitutes precise and accurate observation. The community also dictates what constitutes a valuable contribution to the knowledge base. The relative value of a contribution is a function of the extent to which it extends, refines, or challenges particular theories of how the world works. In our everyday world, we do not have a community determining the validity of our thinking or experiences. Thus, the initial conversation when beginning a new area of study provides an important opportunity for the teacher to ascertain children’s awareness of the roots of their knowledge, as well as the expectations of the scientific community. For example, when students describe knowing something about the physical world but indicate that their knowledge did not arise from observation or direct experience, the teacher might ask them to think about what they have observed that might be the kind of evidence scientists would expect to have. When students do provide evidence, the teacher might ask them questions about that evidence such as those above, reflecting the norm that systematic study under controlled conditions is a hallmark of the practice of science, and that evidence not obtained under those conditions would lead scientific thinkers to be skeptical about the knowledge claim.
The next step in engagement is to begin to focus the conversation about the topic of study in ways that are likely to support the learning goals. For example, showing students the kinds of materials and equipment available for investigating can lead to a productive conversation about phenomena they can explore. Focusing on ideas that were generated during the KWL activity, the children can be encouraged to suggest ways they might investigate to determine whether those ideas are scientifically accurate (meaning that the claims can be backed by evidence from investigation). Students can also be encouraged to identify what cannot easily be studied within the classroom (because of the nature of the phenomenon or a lack of resources or time) and might be better studied in a second-hand way (i.e., through
reading or hearing about what others have studied and concluded from first-hand investigation). For example, we observed a group of third graders studying light who had numerous questions about black holes, the speed of light, and light sources on different planets, all of which they decided were best pursued through second-hand investigation.
At the end of engagement, the students should have a sense of a general question they are trying to answer (e.g., How does light interact with matter?), and should have identified a particular question or questions to be the focus of the first cycle of investigation. To this end, a teacher might (1) focus the class on a particular phenomenon to study and have them suggest specific questions, (2) draw upon conflicting ideas that were identified in the KWL activity and have the class frame a question for study that can inform the conflict, or (3) draw on a question that was identified during the discussion that is a profitable beginning for investigation.
Illustration. What does this kind of beginning look like in a classroom? In a kindergarten classroom,13 after a brief opportunity for the children to state what they thought they knew about light and how it behaved, the teacher, Ms. Kingsley, arranged for pairs of students to take turns using flashlights in an area of the classroom that had been darkened. This activity provided children an opportunity to become familiar with investigative materials and phenomena that Ms. Kingsley knew would be the focus of later investigation. The children responded to this activity in a variety of ways, from initially becoming focused on finding spiders to dwelling later on the effects they could create with flashlights. For example, one student commented on the colors she saw as she shone the flashlight on the wall in the darkened area: “There’s color. When it shines on a color, then it’s the color, green, or white, or red, or black. And then you put the light on the ceiling, it’s gone.” In the following interaction, the children “discover” reflection:
|
[Anisha walks forward under the loft, holding the flashlight with her left hand at an angle to the mirror that she holds flat in front of her.] |
Anisha |
Oh Deanna, look, I can bounce the light. [Deanna holds the mirror so light is bouncing directly behind her.] Deanna [excitedly] If you look back, maybe you can see the light. |
A third student focused on what he saw while holding objects in the beam of light. The following interchange occurred when the students explored with large cardboard cutouts of letters of the alphabet.
Jeremy |
[working with a letter] Ooo, this makes a shadow. A different shadow [than the one he just saw]. [He picks up the letter G and hands it to his partner.] See if the G makes a shadow. |
Hazel |
It does make a shadow. See, look at this. |
When the children described their observations to the class, Ms. Kingsley was able to use those observations to elicit the children’s current ideas about light and shadows and how they might investigate those ideas.
In a fourth-grade classroom,14 the teacher, Ms. Lacey, introduced her students to the study of light by asking them what they wondered about light. The children identified over 100 “wonderings,” including questions about how we see, why we see rainbows of color from some glass objects or jewelry, what makes light from the plastic sticks you bend to make them “glow” in the dark, what are black holes, and how fast is the speed of light. The next day, students were given a written assessment about light, presented as an opportunity for them to identify their current thinking about the nature and behavior of light. After reviewing students’ responses, Ms. Lacey wrote statements on the board (see Table 10-1) indicating the variety of ideas the class held about light. The variation in views of light exhibited by the students provided a reason to investigate to determine the accuracy of the ideas and the relationships among them.
TABLE 10-1 Fourth Graders’ Initial Ideas About Light
Light travels. |
Light can be blocked by materials. |
Light travels in a curved path. |
Light can shine through materials. |
Light travels in a straight line. |
Light can go into materials. |
Light travels in all directions. |
Light can bounce off of materials. |
Later in the unit on light, Ms. Lacey turned to other wonderings the students had about color and light. In the following excerpt, she ascertains whether students’ questions came from what they had been told, read, or observed, and she prompted one student to hypothesize about color from what had previously been learned about the behavior of light.
Ms. Lacey |
I know you guys had a few questions about color, so I’m wondering what you know or would like to know about color? What is it you think you want to learn? Levon? |
Levon |
When I said that my shirt’s a light blue, you said how do we know it? And you said we might be able to tell. |
Ms. Lacey |
Mm-hmm. You want to know how you know it’s blue? |
Levon |
And you said we might be able to tell how. |
Ms. Lacey |
Well, I think you want to know why when you see a blue shirt, you—it’s blue. Okay. We might be able to figure that out. Tom? What is it you want to know? |
Tommy |
How you change color with light. I know it’s real, cause I seen it. |
Ms. Lacey |
What did you see? |
Tommy |
Light makes your shirt be a different color. I want to know how to do that. |
Ms. Lacey |
Hmm. Jared? |
Jared |
I’m wondering how light can make color. |
Ms. Lacey |
How light can make color? You think it does? |
Jared |
Yeah. |
Ms. Lacey |
Oh. Marcus? |
Marcus |
I think light is color. |
Ms. Lacey |
You think light is color. Hmm. So, is that a hypothesis or is that something you really think? |
Marcus |
Hypothesis. It’s something I heard. |
Ms. Lacey |
Okay. So we’ll see if that’s right or not. |
Marcus |
How does light blend, blend. |
Ms. Lacey |
How does it … |
Marcus |
Different colors of light blend. Like, in the first-hand, the white light blends with … |
Ms. Lacey |
Do you mean bend? Okay. |
Michael |
I don’t really have a question about color, but I have a question about light. Why do they call light, light? |
Ms. Lacey |
Ah! Good question. |
Marcus |
Cause it’s, cause it’s light, like a light color. You can’t even see it. |
Michael |
And why did they call it that? Why did they call it? |
Ms. Lacey |
What do you think they should call it? |
Michael |
Something ‘cause it’s so light, you can’t see it. |
Chris |
How does color make white? |
Ms. Lacey |
How does color make white? It does? |
Chris |
Mm-hmm. |
Ms. Lacey |
You think so? |
Chris |
Yeah. I saw it in a book. |
Ms. Lacey |
So, that is your hypothesis. |
Ronny |
How does color interact with light? |
Jared |
How does light, how does light form color? |
Ms. Lacey |
How does light make color? You think it does? |
Jared |
How does light form color and make color? |
Ms. Lacey |
Do you think there’s a difference between the word form and make? Or do you think it’s the same thing? |
Jared |
It’s kinda the same. Forms like light, or something. |
Ms. Lacey |
Do you think light forms color? |
Jason |
Yeah. |
Ms. Lacey |
What makes you think that it might do that? |
Jason |
Cause light does. |
Ms. Lacey |
You just think that? That’s a hypothesis you’re thinking. Okay. |
Andrew |
It’s not a, I don’t have a question, but it’s sort of a thought. I read in this book that when colored light reflects off, like, the same color, that it’ll reflect off that. |
Ms. Lacey |
I don’t understand what you mean. |
Andrew |
Okay. If, if there’s red light and it reflected off somebody’s red shirt … |
Ms. Lacey |
Reflected like off like Jared’s shirt? |
Andrew |
Red, yeah, red shirt. |
Ms. Lacey |
Okay. |
Andrew |
And then, to another red shirt and off. |
Ms. Lacey |
So you think this red light can bounce only if it’s on red stuff? Is that what you’re thinking? |
Andrew |
Yeah. Or if it reflects on like green, red light can’t reflect on a green object. |
Ms. Lacey |
Red light can’t reflect on a green object? What would happen to it if wouldn’t reflect? |
Andrew |
It’d stay in. It’ll absorb. |
Ms. Lacey |
You think it might absorb? Could it do anything else? |
Andrew |
[pause] Transmit? |
Ms. Lacey |
You think it might transmit? Oh. Jamal? We’ve got some good ideas here…. |
A common strategy for engagement not illustrated here is the use of a discrepant event—a phenomenon whose behavior or result is unexpected. For example, if one shines a bright, thin beam of light at an angle into a rectangular block of clear, colorless glass with a frosted surface, one can see that the light interacts with the block in multiple ways. Because the object is transparent, students are not surprised to see light through it, but they may be surprised that the light goes through at an angle (refraction), and they are surprised that light also reflects off the block where it enters and where the refracted light exits the block. We can then ask the question: If light behaves in all these ways with this material, does it do the same with other materials?
While it may be easy to engage children with unfamiliar phenomena or new aspects of familiar phenomena, it is more challenging to support them in developing scientific understanding of the world because scientists often “see” the world differently from what our senses tell us. So using the engagement phase to gain knowledge about the conceptual resources students bring to instruction is just the first step. As the knowledge-building process unfolds in subsequent phases, paying attention to how students use those ideas, promoting the use of particular ideas over others, and introducing new ideas are key. In the next phase, the primary focus shifts from eliciting students’ thinking about what the physical world is like to preparing them to investigate it in scientific ways.
The Prepare-to-Investigate Phase
Description. Preparing to investigate is an opportunity for teachers to support children in learning how scientific knowledge is produced. While inquiry often begins with a general question, investigation must be guided by very specific questions. Thus, an important goal of this phase of instruction is to establish the specific question that will be the subject of the subsequent investigation.15 The question must be specific enough to guide investigation, amenable to investigation by children, and central to the unit of study so that students can construct the desired knowledge of scientific concepts, procedures, and ways of knowing. If the teacher presents a question, it is important that this be done in a way that involves the children in discussion about why the question is important and relevant to understanding the broader topic of inquiry. This discussion provides an opportunity to signal the role of questions in scientific investigation and prompts the metacognitive activity that is the hallmark of any good reasoning. If students suggest a question, or the teacher and students together generate the question, it is still important to check the students’ understanding about how the question is relevant to the topic of study.16
Once a question has been specified, attention can turn to determining how the question will be investigated. This is a critical issue for scientists,
and is no less important for children’s developing understanding. The teacher may provide information about procedures to use, students may invent or design procedures, or the teacher and students may work together to determine how investigation should be carried out. Increasingly, there is evidence that children can think meaningfully about issues of methodology in investigation.17 Nevertheless, it is always important for the teacher to check students’ understandings about why particular approaches and procedures are useful to answering the question. To this end, the teacher might ask students to describe the advantage of using particular materials or tools over others, or to tell why particular steps or tools are necessary. Then, during the actual investigation, the teacher should periodically reassess students’ understanding of what they are doing to ascertain whether accurate understanding was sustained in the face of their actual encounter with phenomena. In addition, the teacher can ask students to evaluate the effectiveness and accuracy of their tools or procedures. These actions support students’ metacognitive awareness regarding the question–investigation relationship.
We think of investigation in classrooms as addressing how students should interact with materials, as well as with one another (when investigation is carried out by groups of students). A critical aspect of preparing to investigate is determining with students what they will document and how during their investigation. This may take the form of discussing the extent to which procedures need to be documented (only to a small degree when students are all investigating in the same way, but in detail when groups of students investigate differently), and promoting and illustrating the use of drawings to show investigative setups.
If the amount of data collection has been left undefined, the students will need to consider how they will know when they have collected enough data. The fact that students will have to make and report claims and evidence to their classmates lends greater significance to this issue. Students may find they need to collect more data to have sufficient amounts to convince their classmates of their claim in comparison with what they might have found convincing. Finally, it will be important to have students discuss how to document observations so they are accurate, precise, and informative.
When students are working in groups, assigning them roles can be helpful in supporting them in working together effectively. There are various types of roles that students can adopt during investigation. Possible roles to support effective management of the students’ activity are Equipment Manager, Timekeeper,18 and Recorder. These roles are not unique to scientific inquiry, but other roles are. For example, having the required materials does not mean that students will use them effectively; it is necessary to monitor that the correct procedures are being carried out and with care.
In addition, a number of responsibilities attend data collection, such as
ensuring that enough data will be collected to fulfill the norms of scientific investigation, determining the level of precision with which observations are to be made (e.g., whether length should be recorded to the centimeter, tenth of a centimeter, or hundredth of a centimeter).19 These sorts of issues form the basis for intellectual roles that students can adopt, in contrast to the management roles discussed above.20 These roles, rather than being named for a task, are named for the conceptual focus maintained during investigation. For example, one student in a group can assume primary responsibility for pressing the group to evaluate how well procedures are working and being carried out in order to answer the question. Another student can be given primary responsibility for evaluating the extent to which the data being collected are relevant to the question. Finally, another student can be given primary responsibility for checking whether the group has enough data to make a claim in answer to the question.
If the practice of adopting roles is utilized, the prepare-to-investigate phase is used to set this up. Modeling and role-playing are helpful to support students in adopting roles that are new to them. In addition, the formal assignment of roles may change over time because while management roles may always be needed, intellectual roles represent ways of thinking that we want all students to adopt. Thus, the need to formalize such roles should decrease over time as students appropriate them as a matter of course when engaging in scientific investigation.
Finally, it is useful to give some attention to the issue of how data will be recorded. At times it may be best to provide a table and simply have students discuss how they will use it and why it is a useful way to organize their data. At other times it may be best to have the class generate a list of possible means for recording data. Sometimes it may be sufficient to indicate that students should be sure to record their observations in their notebooks, and have the students in their groups decide what approach is best for recording their observations.
Illustration. In the unit on light and shadows, Ms. Kingsley posed to her kindergarteners the question of whether an object’s shadow can be more than one shape, following the opportunity they had to explore with flashlights prior to beginning any formal investigation. She knew that not all the children had made shadows during their exploration, so she used part of the discussion in this phase to ascertain students’ understanding of how to put objects in the light to make shadows. She showed the class how the materials would be set up, with a light source placed a couple of feet from a wall and a piece of poster paper taped on the wall to allow them to draw the shadows they observed.
During her fourth graders’ investigation of the interaction of light and matter, Ms. Lacey bridged from the children’s wonderings to a question she introduced: How does light interact with solid objects? She began the pre-
paring-to-investigate phase by ascertaining students’ understanding of the question. One boy asked what “interacts” means. She responded that she was interacting with the students, and then asked them to interpret the question without using the word “interact.” The students responded with such questions as: “What would it do”? “How does it act”? “How does it behave”? “How do they act together (but not like in a movie)”? Ms. Lacey then solicited questions about other words in the investigation question, and a boy asked, “What is a solid?” Students responded with statements such as: “A solid is not like water.”“ It’s filled in.” “It’s hard, maybe.” “It doesn’t bend.” At this point, Ms. Lacey picked up a bendable solid, bent it, and asked the students whether it was a solid. Students were divided on whether it was. Ms. Lacey proceeded to review states of matter with the students, discussing properties and examples. She then returned to the preparation for investigating light.
The materials on which the students would shine a flashlight were simple, but there were many of them (more than 20 items), and describing each in order to identify it would have been cumbersome (e.g., blue plastic sheet, colorless plastic sheet, plastic sheet with gold coating on one side). So Ms. Lacey prepared a poster with each type of material mounted on it and numbered. She used the poster to show children the materials with which they would be working, and they discussed the use of the numbers to facilitate documenting their observations.
Ms. Lacey also introduced a new tool to the students: a small rectangular piece of white construction paper, which she called a “light catcher.” This tool functioned as a screen to look for reflected or transmitted light. Figure 10-2 shows the setup Ms. Lacey showed the students, with the letters A and B indicating the places where the students expected they might see light.

FIGURE 10-2 Investigative setup for studying how light interacts with solid objects.
In addition, the class talked about categorizing objects in terms of how light behaved. Ms. Lacey asked the students what they thought the light might do, and they discussed categorizing the objects based on whether light bounced off, went through, became trapped, or did something else (the students were not sure what this might be, but they wanted to have a category for other possibilities).21 In the course of that conversation, Ms. Lacey introduced the terms “reflected,” “transmitted,” and “absorbed,” which she stated were terms used by scientists to name the behaviors they had described. There was some discussion about what it meant when an object blocked light: Did that mean light had been absorbed, or was it simply stopped by the material? Ms. Lacey suggested that the class leave that question open, to be discussed again after they had investigated and had the opportunity to observe the light.
Ms. Lacey chose to focus students’ recording of their observations by preparing a simple table for them to complete: a column for the number/ name of the material and a column each for indicating whether light reflected, transmitted (went through), or was absorbed (trapped by) the material. The use of the table seemed straightforward, so there was little discussion. Ms. Lacey later noticed that most students used the table as though their task was to determine which single column to check for each object. She realized that the students needed guidance to check for each object whether light was reflected, transmitted, or absorbed. The next time Ms. Lacey taught this topic, she made two changes in this phase. First, she was careful to raise the question of whether light could behave in more than one way with a material. Students were divided on whether they thought this was possible, which gave them a reason to investigate and supported them in realizing the need to be thorough in observing light with each object. Second, she asked students how they might provide evidence that light did not interact in particular ways with an object. This discussion led students to realize that they would have information to record in each column of the table, and that what does not happen can be as informative as what does happen.
The Investigate Phase
Description. In this phase, students interact with the physical world, document their observations, and think about what these observations mean about the physical world. The teacher’s role is to monitor students’ use of materials and interactions with others (e.g., in small groups), as well as attend to the conceptual ideas with which students are working and the ways in which their thinking is similar and different from that of their classmates.
Investigating involves the interaction of content and process. It may appear to students to be more about process because what we observe is a function of when, how, and with what tools we choose to observe. At the same time, what we observe is also a function of what we expect to observe, and how we interpret our observations is clearly influenced by what we already know and believe about the physical world. For example, we have experienced children describing only one type of interaction when shining light on objects because they expected that light could interact in only one way. Thus they described light as only “going through” a piece of clear, colorless plastic wrap even though we could see bright spots of light on the front of the wrap indicating reflected light. Furthermore, students described light as only “being blocked” from a piece of cardboard even though a disc of light the size of the flashlight beam could be seen on the back of the piece of cardboard, indicating that light was going through it.22
The teacher determines whether and when to prompt students’ awareness of the ways in which their prior knowledge may be influencing their observations. With respect to students’ interactions with materials, it is important to monitor whether students are using them appropriately. Students invariably use materials in unexpected ways; hence, the teacher needs to observe student activity closely. When students use materials incorrectly, the teacher needs to determine whether to provide corrective feedback. Since it is important for the development of metacognition that students be in the “driver’s seat” and not simply follow the teacher’s directions, determining whether, when, and how to provide feedback is critical. If the teacher judges that the students’ activity is so off the mark that the targeted learning goals will be sacrificed, it is critical to provide prompt corrective feedback. An example in the study of light would be if students measuring angles of the path of light coming into and reflecting off of a mirror were using the protractor incorrectly.
Other cases, however, provide opportunities for students to become aware of gaps in their thinking. An example of this occurred when the teacher in the kindergarten class studying light and shadows noticed that some students were tracing the object directly on their recording paper rather than tracing the object’s shadow. When the teacher saw this happening, she joined the group as they were working and began to ask them about their data. In the course of the conversation, she asked them to show her how they had made the shadows, which led them to indicate that some were tracings of the objects themselves, not the shadows. She then asked them, “If our question is about shadows, which drawings show shadows?” The students were able to point to their drawings that were shadows. She then asked, “How could you mark your drawings so that you can tell which ones are shadows, so that when we look for patterns, you’ll know which drawings to look at?” They devised a scheme—to draw dots around the
drawings that were shadows—and the teacher moved on to another group. Later, when the former group reported, the rest of the class learned about their strategy and how they had dealt with the “mixed” nature of their observations.
Another important category of feedback is when the teacher brings out the norms and conventions of scientific investigation (e.g., holding conditions the same when trials are conducted, measuring from the same reference point, and changing only one variable at a time). Attention to such issues can be prompted by asking students about the decisions they are making about how to investigate. For example, in the fourth-grade investigation of the interaction of light and matter, one group’s response to Ms. Lacey’s question about what they had found out revealed a lack of attention to the transmission of light. Ms. Lacey handled this in the following way:
Ms. Lacey |
When we were preparing to investigate, we said that light might also be transmitted, but I didn’t hear you say anything about that. Did you check for that? |
Student |
No, but we already know light doesn’t go through these materials; they block it. |
Ms. Lacey |
But remember that scientists believe it is important to test out such ideas, and as scientific thinkers, your classmates will be encouraged to look for such evidence. How will you convince them that these materials don’t transmit light? |
Here Ms. Lacey gave students an important message about the need to rule out possibilities instead of relying on assumptions.
With small-group investigation, in addition to general monitoring to support student collaboration, the teacher needs to be attentive to whether differences in students’ ideas create difficulties. In the excerpt below, two kindergarten children in Ms. Kingsley’s class are investigating reflection from a mirror. Their initial conflict is due to Brian’s interest in placing the mirror so that its back faces the light source. Amanda objects because her exploration during the engage phase revealed that reflection is best from the front of the mirror. She is very interested in seeing the reflection because the class is examining a claim she made from her exploration activity, which was that you can use a mirror to make light “go wherever you want it to.”
Amanda |
[tracing line to mirror] This goes to here. The light has to hit the mirror. Then … |
Brian |
I want it to go that way. [referring to placement of the mirror with its back to the light source] |
Amanda |
No, the mirror has to face the light source. |
Amanda |
[turns mirror to face the light source] Lookey! Light, see. |
Brian |
[turns mirror back around] Lookey, no light, see. |
Amanda |
But that’s because it’s not facing that way. [turns mirror to face the light] |
Brian |
You said you could move it wherever you wanted it to go. So your plan has failed…. |
Amanda |
The light has to do the—okay. This is the light source, right? [points to source] This light has to hit the mirror … And then, look, look, see … Now you think my plan works, see? Watch, see … |
Brian |
[takes hold of mirror] I can’t make it go this way! [referring to making the light go behind the mirror] If I take this off [removes mirror from where it rests on their drawing paper], it’s going my way. But [puts mirror back onto paper], it’s not going my way. |
Amanda |
The mirror has to face … The light has to hit the mirror. [taps mirror with hand] And look: light, light, light. [points to reflected beams of light] |
Brian |
But you said it could go anywhere. You said it could go anywhere you wanted it to go and I wanted it to go backwards, like this. [referring to making the light go behind the mirror] |
Amanda |
But the mirror [forcefully places the mirror on their drawing paper] has to face the light source [forceful gesture toward light source], face the light source, and THEN you can move it. [referring to the reflected beam of light] |
The interaction of content and process that occurs during investigation means that teachers must be mindful of children’s cognitive activity as they undergo and interpret their experiences with the physical world. Teachers should ask students what they are observing and what they think their observations mean about the question under investigation. Sometimes it is useful to ask students why they think what they are doing will help them answer the question. In addition, the teacher needs to observe what stu-
dents observe so students can be prompted to notice important phenomena they might otherwise ignore or be encouraged to pursue observations the teacher believes useful to the knowledge-building process.
When investigative procedures are simple, students are able to focus more of their attention on what the data are and what these data suggest about the question being investigated. When procedures are more complex, students need more time to focus on the meaning of the data apart from the actual investigation. When students design the investigation themselves, they may need to give more attention during the investigation to evaluating how well their plans are working so they can make adjustments. Thus, teachers need to monitor how well children are handling the complexity of the investigation so that sufficient time is allocated to support the knowledge-building process.23
Once the data have been collected, students need to analyze them. Identifying patterns is a deductive analytic process in which students work from specific datasets to identify general relationships. From this step, students make knowledge claims, just as scientists would. That is, they make claims about the physical world, using the patterns they identified to generate those claims. We consider this aspect of investigation to be a different instructional phase because the nature of the cognitive activity for the teacher and students has changed. This aspect is discussed as part of the preparing-to-report phase.
Illustration
To illustrate the investigation phase, we draw upon an event that occurred in Ms. Kingsley’s kindergarten class during their investigation of light and shadows. Amanda and Rochelle were working together, with Amanda basically directing Rochelle. When Ms. Kingsley checked on them and asked questions to determine their thinking about what they were finding out, it became clear to her that Amanda was quite certain that the shadow from an object could be only one shape, and Rochelle appeared to go along with whatever Amanda thought. While Amanda’s thinking was incorrect, Ms. Kingsley chose not to intervene, recognizing that during reporting, the children would have the opportunity to see a wider range of data and possibly reconsider their thinking (see the illustration of the reporting phase).
The following excerpt is from Ms. Lacey’s fourth-grade class. This interchange occurred early in the investigation, and Ms. Lacey was checking on a group of three girls that she knew from previous experience had found investigative activity challenging. She began by asking which materials the students had used in their investigation and what they had found out. She learned that one student in the group had been working independently instead of with the other two, and they had not been discussing their results.
Ms. Lacey encouraged them to work together, especially since it would be helpful to have one person holding the flashlight and material, and another person using the light catcher.
Ms. Lacey |
[picks up a blue styrofoam object and shines the flashlight on it] What’s it doing? |
Mandy |
Some goes through. |
Ms. Lacey |
How do you know? |
Mandy |
Some blue light is on the wall. |
Ms. Lacey |
Does it do anything else? [Ms. Lacey directs the student to use the light catcher to check other possibilities.] |
Mandy |
Some is reflected. |
Ms. Lacey |
Write that down. |
Perhaps the most important question asked by the teacher in this excerpt is “How do you know?” This question is at the core of distinguishing systematic research from our everyday sense making. It also sent the message that the students were accountable for their observations, and allowed Ms. Lacey to indicate the need to check for multiple ways in which light might behave with the object.
The Prepare-to-Report Phase
Description
As the activity shifts to a focus on the public sharing of one’s findings from investigation (reporting phase), the role of the class as a community of scientific thinkers takes on new meaning. In scientific practice, this phase marks a shift in emphasis from divergent to convergent thinking, and from operating with the values, beliefs, norms, and conventions of the scientific community in the background to operating with them in the foreground.24 Now it matters a great deal what fellow classmates will think and not just what the investigating group thinks.
In this phase, just as scientists use their laboratory documents to prepare papers for public presentation to the larger scientific community, students use the information and observations in their notebooks to prepare materials for public presentation to their classmates. The public nature of sharing one’s claims and evidence means that students need to determine the claim(s) for which there is enough evidence to warrant public scrutiny, and what data they should feature as the compelling evidence backing their own claim(s) and supporting or refuting the claims of others.
Students can use poster-size paper as the medium for reporting, thus allowing the information to be large enough for everyone in the class to see. Posters are expected to include a statement of the group’s knowledge claim(s), as well as data backing the claim(s); if groups investigated different questions, the poster should include the question as well. Data may be presented in written, tabular, or graphical form (including figures or graphs), and students may decide to include a diagram of the investigative setup to provide a context for the data. (This is to be expected when students investigated in different ways.) As each group prepares its poster, students should be thinking about how to present their findings to best enable others to understand them, and be convinced of the group’s claim. Decisions about how to state a claim and what data to include in presenting one’s claim provide important learning opportunities.
A major aspect of the teacher’s role in this phase is to reflect the norms of the scientific community regarding the development and evaluation of knowledge claims. In the scientific community, for example, there is an expectation that relationships will be stated precisely and backed by unambiguous and reliable data. It should also be recognized that claims can be stated in the negative, thus indicating a relationship that is claimed to be inaccurate—for example, the brightness of the light source does not affect whether light reflects from an object. Such claims help the community narrow its consideration of possible relationships.
Another role of the teacher is to help students attend to issues that may affect the quality of their public presentation. For example, teachers can encourage students to draw as well as write out their ideas to communicate them more effectively. Furthermore, teachers can prompt students to evaluate their poster for its effectiveness in communicating findings. For example: Is it readable? Are things clearly stated? Is there enough information for others to evaluate the claim or be convinced of its validity?
Finally, a key role for the teacher is to monitor the types of claims students are generating and the nature of the evidence they are selecting. The teacher determines whether and to what extent to prompt students’ awareness of the role played by process in determining what they observed (e.g., ascertaining students’ awareness of imprecise or inaccurate data). With respect to content, the teacher determines whether and when to focus students on particular strategies for interpreting or analyzing their data or to provide additional information to support students in writing claims. It may be necessary for the teacher to help groups reorganize their data to find patterns. For example, Table 10-2 shows two tables. The top table shows the data as they were originally recorded. The order of the columns matches the order of places that students looked to check for light from the flashlight. The order of objects in the first column is simply the order students selected to observe them. The bottom table shows the same data in a similar form,
TABLE 10-2 Data Tables from Initial Recording and with Revisions for Analysis Purposes
Original Data Table and Observations: |
|||
Object |
On Light Catcher in Front of Object (reflected) |
On Back of Object (transmitted) |
On Light Catcher Behind Object (absorbed) |
Clear glass |
dim light |
bright light |
light shadow |
Purple glass |
dim purple light |
bright purple light |
dark purple shadow |
Silver wrap |
bright light |
no light |
dark shadow |
White plastic sheet |
dim light |
medium light |
medium shadow |
White typing paper |
bright light |
dim light |
medium shadow |
Black felt |
no light |
no light |
very dark shadow |
Orange cardboard |
dim orange light |
dim reddish light |
dark shadow |
Reorganized Data Table and Simplified Observations: |
|||
Object |
On Light Catcher in Front of Object (reflected) |
On Back of Object (transmitted) |
On Light Catcher Behind Object (absorbed) |
Black felt |
no light |
very dark shadow |
no light |
Orange cardboard |
dim light |
dark shadow |
dim light |
Purple glass |
dim light |
dark shadow |
bright light |
White plastic sheet |
dim light |
medium shadow |
medium light |
Clear glass |
dim light |
light shadow |
bright light |
Silver wrap |
bright light |
dark shadow |
no light |
White typing paper |
bright light |
medium shadow |
dim light |
but to facilitate looking for patterns, the columns and rows have been reordered, and the data have been simplified (information about color has been removed). This type of reorganization and simplification of data is common for scientists, and may be necessary for students to find patterns from which to make a claim.
Often, the teacher’s support is at the level of helping groups figure out
how best to state the claim(s) they want to make from their data. It does not include evaluating whether their data support the claim; that is part of the reporting phase and should be shared by the class.
On the other hand, the teacher may choose to support students in making additional claims based on the data they have, particularly in instances where the group has unique data to make a claim that the teacher believes would promote desired knowledge-building for the class. In Ms. Lacey’s fourth-grade class, for example, despite students’ assumptions that light would behave in only one way with an object, a group had evidence that light behaved in more than one way. Given that this was the only group in the class making such a claim from that body of evidence, Ms. Lacey supported the group to ensure that they would include the claim in their poster so it would be introduced to the whole class.
An alternative approach involves the teacher’s questioning students during the prepare-to-investigate phase to lead them to consider the possibility that light may behave in more than one way. The emphasis in this case may be on ruling out the possibility of disconfirming evidence. With this approach, the teacher monitors during the investigation phase whether students are checking for multiple possibilities, and will know whether the students observe light interacting with objects in more than one way.
Illustration. The following excerpt from an investigation of light by third graders shows a typical teacher–student interaction as students attempted to generate knowledge claims.25 The students were working with light boxes producing narrow beams of light and had been given latitude regarding which questions—identified during the engagement phase—they would like to study. As a result, different groups of students investigated with different types of materials. In the transcript, note that the students did most of the talking. The teacher primarily asked questions to determine the nature of the students’ thinking. Note also that the teacher reflected an important norm of scientific activity by asking the students how they planned to represent the observations supporting their claim.
Ms. Sutton |
What claim are you working on right now? |
Don |
We had to change it because we thought that the speed of light would be a [second-hand investigation]. |
Ms. Sutton |
Mm hmm. |
Kevin |
So, light can reflect off a mirror. Any other object that’s not a mirror, like a piece of paper. Let me demonstrate. [Ms. Sutton: Okay.] This is a piece of paper. You see, when the light hits the paper, it disappears. But before it disap- |
|
pears, it hits the paper, it goes through the paper. It disappears. |
Ms. Sutton |
Hmm. Does all the light disappear through the paper? |
Kevin |
No. Okay, you see all the light that’s coming through, from this hole? |
Ms. Sutton |
Yeah. |
Kevin |
It goes to the piece of paper. It disappears when it hits that piece—that object. |
Ms. Sutton |
Where do you think it goes? |
Don |
Through the paper. There’s a little light over here. And it stops here because it doesn’t have enough power to go anymore. |
Ms. Sutton |
Okay. Hang on a second. So, you’re saying a little bit of light goes through the paper. And you think the rest of the light just disappears? |
Kevin |
No. The rest of the light that hits the paper disappears from the light—from the object, cause it’s not a mirror. But if it hits the mirror it can reflect off of it. |
Ms. Sutton |
So if it’s a mirror, the light goes in another direction, or reflects off. If it’s something besides a mirror … |
Kevin |
It doesn’t get reflected. |
Ms. Sutton |
It just disappears, it doesn’t reflect? |
Kevin |
Yep. |
Ms. Sutton |
Okay. Are you going to try to prove that some way to the group? You have to show some data. |
Don |
Well, it’s not exactly data. We sort of … |
Kevin |
I drew a picture out here. |
Ms. Sutton |
How could you show that? We could get another piece of paper. Save what you’ve got so far. How could you show on another piece of paper how the light is different with different—with the mirror and with the paper? How could you show it? What you just said—so you could show it to the rest of the group? |
Kevin |
We can draw the top and just say that the light is coming through—put light right here. And then the light through—going out of the box. And then we can put, make like a little part of it |
|
like this, like the target. And put the paper right here. |
Ms. Sutton |
So, Kevin is saying, when the light hits the mirror, it looks one way. When the light hits a piece of paper, it looks another way. How could you show how it looks those two ways on a piece of paper? |
Don |
And, another thing is, I sort of drew this thing. That’s the light that’s over here that goes there. And then when it hits these, it stays there and it doesn’t come back. |
Ms. Sutton |
That’s interesting, too. But you guys need to stick to one claim and deal with that. When you think you have evidence for that, if you want to explore something else and have some time, you could do that. |
The Report Phase
Description. A critical feature of inquiry-based instruction is the point at which students’ findings are publicly shared and discussed. This phase has two parts (see Figure 10-1). First, groups of students who have been investigating together present their claims and evidence, which are discussed by the class in terms of their own merits and in light of the findings presented by previous groups. Second, the class discusses the commonalities and differences among the claims and evidence presented, noting claims that can be rejected, developing a class list of community-accepted claims, and determining claims or questions that need further investigation. In addition to providing occasions for discussing important issues related to the investigative process (e.g., possible errors, missed observations), public reports require students to make and defend statements about their understandings, and provide occasions for examining their own thinking and sense making as well as that of others.26 In addition, when students publicly share their results, the need for vocabulary and a common language to communicate ideas becomes salient. Thus, there is an important opportunity for the teacher to support and guide students in the use of scientific terms to facilitate their communication.
When students first experience this activity, the teacher plays a pivotal role in communicating and modeling expectations for audience members. This includes establishing and maintaining conversational norms. Despite the fact that children may need to challenge the ideas or work of their classmates, the teacher is key in setting the tone so that this is done with the
understanding that the students are all thinking together so they can collectively determine how to understand the aspect of the physical world under investigation. The primary expectations for audience members are to determine whether there is a clearly stated claim that is related to the question under investigation, whether there is evidence backing that claim, and whether the evidence is unambiguous in supporting the claim. The issue of unambiguous support concerns whether there is any evidence—either from other groups or from within the presenting group’s data—that would counter the claim. With teacher modeling and practice with the teacher’s feedback, students become able to sustain substantive conversations regarding the knowledge they are developing about the physical world.
The reporting phase is particularly complex and rich with opportunities for the teacher to engage in supporting children’s thinking and actions. As each group shares its claim(s) and describes the relationship between these claims and their data, the teacher assumes multiple roles: monitoring for understanding, working with the students to clarify ambiguous or incomplete ideas, seeding the conversation with potentially helpful language or ideas, and serving as the collective memory of prior conversations (both in the whole-class context and in the small-group investigation contexts). The challenge in this phase of instruction is to promote the group’s advancement toward deeper understanding of the phenomenon under investigation, as well as the nature of scientific ways of knowing, using the fruits of the investigation activity and the collective thinking of the classroom community.
The reporting phase culminates with the whole class discussing the claims that have been shared to determine which if any have sufficiently convincing evidence (and a lack of contradictory evidence) to elevate them to the status of “class claim”—indicating that there is class consensus about the validity of the claim. This discussion of claims typically results in identifying where there is disagreement among claims or contradictory evidence related to particular claims (e.g., when the data presented by one group can also be used to contradict the claim of another), which provides the motivation for the next cycle of investigation.
Illustration. Excerpts from classroom instruction illustrate various aspects of teacher and student activity during this phase. The following transcript is from the beginning of the reporting phase in Ms. Lacey’s fourth-grade class. Ms. Lacey introduces students to the class claim chart, on which the class will track the claims that have been introduced and the classroom community’s reaction to them. She also forewarns students that they have conflicting views, anticipating the need to prepare the students to hear things from their classmates with which they will not agree.
Ms. Lacey |
And we’re going to start making a list of claims. Or we might have a list of—we don’t know whether we believe that or not…. Some of our claims may end up being “think abouts.” We need to think about them some more…. You know what? You guys don’t all agree. I’ve been to every group … so you better pay attention. They may not convince you, but you might think to yourself, “aha! I’m gonna try that.” Or, “I might need to check that out.” |
Ms. Lacey’s introduction of the class claim chart sends an important message about the dynamic nature of the inquiry process: reporting is not a culminating activity; it is part of an ongoing activity, the next phase of which will be shaped by what has just transpired. Her decision to alert students to the presence of conflicting ideas provides an authentic purpose for paying attention to one another during the reporting phase and stimulated metacognition.
In the next excerpt, a student questions one of the claims made by the reporting group. The group made a claim that “light can’t be trapped” and cited as evidence that “you can’t roll it up and throw it.” The students’ interaction presents the teacher with many issues to which she could react to support the students’ development of scientific knowledge and ways of knowing.
Bobby |
When you said that you believe that light can’t be trapped because it’s a gas, you can’t roll it up and throw it. What do you mean? |
Megan |
We mean we can’t grab light and throw it at someone. |
Heather |
It’s not solid. |
Megan |
It’s not a liquid, either. |
Bobby |
So you’re saying that light is a gas? How do you know light is a gas? |
Heather |
Air is a gas, and you can’t feel it. Well, you can feel it only when it’s blowing. But you can’t feel light because it’s not blowing. |
Bobby |
So you guys are saying that you think light is a gas because light is like air? |
Ms. Lacey could have pointed out that a claim about light being a gas is unrelated to the focus of this particular investigation; she could have trun-
cated the interaction by providing the information that light is a form of energy, not matter; she could have identified this claim as one that requires further exploration, perhaps in a second-hand way. But Ms. Lacey chose not to interject at all. While this decision has limitations with respect to developing scientific knowledge about light, it has the advantage of giving the students opportunity and responsibility to examine one another’s thinking with respect to the norms and conventions of scientific practice, as illustrated by Bobby’s pressing the girls to address how they know light is a gas. Such questions can provide opportunities for students particularly interested in a question to pursue it outside of class, or resources might be brought into the class (books or descriptions downloaded from the Internet) that provide information pertinent to the question.
In the next two excerpts, Ms. Lacey responds in two different ways to students’ questioning of the reporting group based on her judgment of the reasons for those questions. In the first excerpt, she responds to confusion that she suspects arises from the way students are interpreting language in the phrasing of claims. The excerpt illustrates the language demands involved in both representing one’s thinking in a claim and interpreting the claims of others.
Barbie |
I’m confused—“we believe light does go in a path.” Well, how do you know it goes in a path? It could go different ways. [“A path” appears to be interpreted as “one path.”] |
Megan |
We tried it on the flashlight. It’s just straight. [“A path” appears to have meant “a straight path.”] |
Barbie |
Cause there’s a whole bunch of light. Light can go [other ways] [shows with hand]. [“A path” appears to be interpreted as “one particular path” instead of many possible paths.] |
Megan |
We don’t believe that. |
Ms. Lacey |
Can you draw a diagram on the board? [Change from an oral to a written medium may resolve issues due to language demands.] |
The girls used a context from their preinstruction assessment—a tree, a person, and the sun—to show two different possibilities regarding the path of light: wavy and straight lines. They drew multiple paths from the sun and pointed to the straight lines as the representations that matched their claim. Ms. Lacey then worked with the class to modify the students’ claim about the path of light so that it was consistent with the illustration:
Ms. Lacey |
[to class] Can you think of some way they could switch that claim to make more sense to us? She’s telling us one thing, and they didn’t put that one word in. [to Megan and Heather] Cause you don’t think it goes wavy, you think it goes … |
Megan |
Straight. |
Ms. Lacey |
How could you change your claim to say that? |
Heather |
We believe light goes only in a straight path. |
Ms. Lacey |
[to class] Will that make better sense to us? |
Class |
Yeah. |
In the second excerpt, a student struggles to make sense of the claim that light reflects and goes through. Ms. Lacey suspects, because of the student’s language, he has difficulty conceptualizing that light can behave in multiple ways simultaneously. As a result, she intervenes, asking a question to help achieve greater clarity regarding the student’s confusion:
Megan |
Yeah. Stefan? |
Stefan |
Reflect and go through—on the plastic tray. When you put it on reflect, it reflected off the plastic tray. And when you put it on go through, it went through the plastic tray. But I don’t get it. If it reflected off, then how did it go through? |
Megan |
Well, we put it on an angle and shined it and it went on our screen. And when we put it straight, it went through. |
Ms. Lacey |
Stefan, are you having a hard time thinking that light can do two things at once? If it reflects off, why did it also go through? Did they explain? |
In both of the above examples, as well as in the excerpt at the beginning of this chapter in which a second-grade student objected to a claim about light reflecting from wood, students are revealing that they lack a conception of light that allows it to behave in the ways indicated by other students. Brad does not have a way to think about light that would account for its ability to reflect from wood. Stefan does not have a way to think about light that would account for its ability to simultaneously reflect and pass through an object. How does some of the light “know” to reflect, while other light gets transmitted through the material? These are reasonable issues, and we should not be surprised that the students do not readily accept claims
that speak to a reality they do not believe. It is part of the scientific culture to be skeptical about claims that do not fit existing scientific theories, as these claims clearly did not fit the students’ preexisting ideas. Indeed, there are numerous examples of scientific papers that presented novel scientific claims and were rejected by top scientific journals because of their inconsistency with prevailing knowledge and beliefs, but later became highly regarded and even prize-winning.27 Thus when such events occur, it is important for the teacher to recognize that the issue is the fit between the idea presented and the students’ conceptual framework. As How People Learn suggests, it is precisely in these situations that students’ thinking must be fully engaged if they are to develop desired scientific understanding.
There are several ways to proceed in such circumstances. Some research has demonstrated that having students observe relationships can lead them to change their initial thinking about those relationships,28 or at least come up with alternative ideas.29 In the case of the second grader who was skeptical about the reflection of light, this would mean setting up the materials so he could observe the reflection from wood that his classmates saw and providing opportunities to examine the reflection from other solids. Other researchers have proposed engaging students in reasoning through a series of phenomena that are closely related,30 helping students bridge analogous circumstances. In the case of disbelief about light reflecting from wood or other nonshiny solids, this might mean starting with observing instances of reflection that students readily accept (e.g., reflection from a mirror); linking to observations of a very thick mirror, whereby the light beam can be seen traveling through to the silvered back surface of the mirror and reflecting from there; linking to reflection from a less reflective surface, such as lead (a metal, but not shiny); then linking to a similarly less reflective surface but of a different type, such as gray construction paper; and so on. The bridging could go as far as examining reflection from black felt, a material students are initially quite sure does not reflect light, but can be observed to do so if the room is dark enough.31
Another approach to addressing the nonacceptance of claims that contradict everyday experience is to tell students that part of learning science means developing new conceptions of reality.32 This does not necessarily mean discarding existing ideas.33 However, it does mean that students need to recognize that in a science context, the cultural beliefs and practices that guide knowledge production in the scientific community dictate what knowledge is valued and accepted and hence is considered scientific knowledge,34 and that they need to operate accordingly in their knowledge-building activity during science instruction.
Despite the challenge of accepting claims that are initially counter to everyday thinking, we have regularly observed students, even very young children, developing new ideas that are counter to their initial thinking. The
following example comes from Ms. Kingsley’s kindergarten class during their study of light and shadows. The class was discussing two claims that arose from the day’s investigation and were posted on the board: (1) an object can make more than one shadow shape, and (2) an object can make only one shadow shape. When Ms. Kingsley asked the class to evaluate the claims in light of the data from students’ investigations, which were also posted, Amanda, who had repeatedly stated her view that an object’s shadow can be only one shape, gave the following response:
Ms. Kingsley |
Okay, look at the evidence we’ve got here. Does it support the claim that objects make more than one shadow? |
Amanda |
Both. |
Ms. Kingsley |
You think it says both Amanda, tell me why. |
Amanda |
Because um [touching each of the posters with multiple shapes of shadows], all shadow, all shadow, all shadow, all shadow. [touching each of the drawings containing only one shape of shadow] One shadow, one shadow. |
Here, Amanda correctly pointed out that the data did not conclusively support one claim over the other, drawing attention to the ambiguity of the results. This provided a reason to investigate further, so the teacher suggested that the class do so the next day. The next excerpt is an exchange that occurred following the next day’s investigation. Again, all the groups’ data were posted at the front. After examining the data from the second day, all of which showed more than one shadow, Amanda provided a different evaluation of the evidence:
Ms. Kingsley |
We need to find out if the documentation supports that a shape can make one shadow or more than one shadow. Does this evidence support the claim … [points to the two posted claims] |
JT |
More! |
Derek |
One! |
Amanda |
The first one [an object can make more than one shadow] is true. |
Ms. Kingsley |
Why? |
Amanda |
Because one object can make more shadows, see? Because look at all these shadows on the papers. [runs hand along all the posters because they all show multiple shapes of shadows for an object] |
Of note is that Ms. Kingsley and the other teachers featured in this section allowed the children to work with the ideas they had, but pressed them to continually reexamine those ideas in light of the results of their own and others’ investigations. Amanda needed the time of several cycles of investigation to become convinced of a different idea from the one she initially held. Thus, the cycling process of investigation within the same context is an important aspect of promoting desired development of scientific knowledge and ways of knowing.
Second-Hand Investigation
Our focus thus far has been on the development of understanding through first-hand investigation. Such experiences give students repeated opportunities to articulate and test their reasoning and ideas against one another’s first-hand observations, and steep them in the differences between a scientific approach to knowledge building from experience and a more casual everyday approach. However, inquiry-based science instruction can also profitably include learning from text-based resources (as suggested by the National Science Education Standards).35 The study of accumulated knowledge is authentic to scientific practice36 and involves cognitive activities that have many similarities with first-hand inquiry about the physical world.37 Second-hand sources can also reliably focus student attention on the core concepts of interest. The question is how to engage students in such activity in a way that keeps them actively engaged intellectually relative to scientific ways of knowing and permits a skeptical stance that is common to a scientific mindset.
To achieve this goal, we developed a novel type of text for inquiry-based instruction, whose use is called a second-hand investigation. These texts are modeled after the notebook of a scientist and so are referred to as notebook texts. They consist of excerpts from the notebook of a fictitious scientist, Lesley Park, who uses her notebook to “think aloud” regarding the inquiry in which she is engaged, sharing with the reader her observations of the phenomenon she is studying, the way in which she has modeled that phenomenon, the nature of her investigation, the data collected in the course of her investigation, and the knowledge claims suggested by the data.38
We share excerpts from this instruction to illustrate how text can be approached in an inquiry-based fashion to support students’ engagement in scientific reasoning and what role the teacher plays in such activity. The specific notebook text with which the children were working reports on an investigation with materials very similar to those used by the students in studying the interaction of light with matter, although there were several differences in Lesley’s investigation, including her use of a light meter to measure the light she observed.
Of note are the various ways that the teacher, Ms. Sutton, supported the students’ learning from the text. For example, she led the students in a quick overview of the text during which the students identified the features that signaled this was a scientist’s notebook: a header with the scientist’s name and date of activity, drawings showing investigative setups, and tables of data. During the reading of the text, a significant amount of time was devoted to examining the relationship between the information in the notebook and the students’ own experiences. Ms. Sutton accomplished this by revisiting the claims list arising from the students’ own first-hand investigations. The students identified those claims on which there was consensus and those that were still under consideration, but for which there was insufficient evidence. In addition, there were numerous instances in which Ms. Sutton called the students’ attention to vocabulary that was introduced in the notebook text and how it compared with terms the students had been using in their own writing and discussion (e.g., Lesley’s use of “absorbed” to describe the behavior students referred to as the “blocking” of light).
The following three excerpts illustrate how the text, in combination with the teacher’s facilitation, supported the students’ engagement in scientific reasoning. In the first excerpt, the students have encountered a table in which Lesley presents data in units she calls “candles.”
Ms. Sutton |
Okay, it’s the readout of how many candles. And right now it’s showing the flashlight all by itself has …? |
Leo |
Ten candles. |
Ms. Sutton |
Ten candles. |
Jihad |
Could it be like 10.5 or something or 10.3? |
Ms. Sutton |
I would imagine. Don’t you think it could go up or down depending on how bright the light is? |
Jihad |
So, if she puts zero candles, so that means it doesn’t transmit at all? |
Ms. Sutton |
Yes. Good observation. |
Tatsuro |
Are there such thing as like, um, a millicandle? |
Ms. Sutton mediated the students’ sense making with the table. To understand any of the other findings in this table, it was important for the students to recognize that the amount of light from the light source (the flashlight) was “ten candles.” This discussion, however, led several students to wonder about this unit of measure. Transferring their knowledge about other units of measure, they inquired about the system from which this unit
is derived and how that system “works” (i.e., whether it works like the metric system).
In the next excerpt, the students have encountered Lesley’s claim that “all objects reflect and absorb light.”
Ms. Sutton |
What evidence did you see that would support that [all objects reflect and absorb] even though that wasn’t your claim? |
Ian |
That almost all the objects did and maybe if we used a light meter, we might have found out that every single object did a little. |
Ms. Sutton |
How about you, Megan? |
Megan |
Some objects did both things—two different things, but not … we didn’t, like, kind of find out that for all objects … |
Ms. Sutton |
If you had done more, do you think we might have? |
Megan |
Maybe. |
Ms. Sutton |
If you had tested more? |
Megan |
We didn’t do all the objects, yet. |
In this exchange, we see how Ms. Sutton related the second-hand investigation to the students’ first-hand investigation by calling their attention to the differences between their claims and Lesley’s claim. This led to a discussion of two issues: the role of measurement and the sample size. Lesley used a light meter to collect her data, while the children had no means of measurement; they simply described their visual observations as precisely as possible. Ian suggested that with a measuring device, the class’s findings might have been consistent with Lesley’s. Ms. Sutton introduced the possibility that additional investigation might have yielded a different finding, to which Megan responded that the class had not investigated with all the materials yet. Determining how much evidence is enough to make a broad claim confidently, such as “all objects reflect and absorb light,” is fundamental to scientific problem solving.
In the following excerpt, the students entertain other possible explanations for the differences between their findings and Lesley’s. In this instance, Lesley is reporting the data for what happens when a flashlight shines on a piece of black felt. She reports that no transmitted light was recorded by her light meter. The majority of students, however, reported having seen transmitted light. Here the class considers why there might be these different findings:
Catherine |
When we stuck the lamp like, not like directly next to the black but a little bit up close to the black, it came out a maroon color on the other side. |
Ms. Sutton |
So we were getting some transmitted. We thought we had some transmitted light, too. She’s not getting—detecting that, is she, with her light meter? |
Jihad |
But she would be more sure because she has a light meter and we don’t. |
Ms. Sutton |
What might cause a difference in results from what you did and from what she did? |
Student |
She may have had her flashlight back farther and we had ours up very close. |
Ms. Sutton |
Anything else might have made a difference? Ian? |
Ian |
She might have either had a weaker flashlight or a thicker piece of felt or something. |
Ms. Sutton |
Okay, so two things there. |
Student |
Yeah, or maybe it was because of the light meter. |
Ms. Sutton |
What about the light meter? How would the light meter make it harder to detect transmitted light? |
Tatsuro |
Because it’s in, measuring in the tens. What if it was like 0.09? |
Ms. Sutton |
So maybe it’s not measuring to the tenth or the millicandle? |
Student |
Or maybe she’s just rounding off. |
Ms. Sutton |
Maybe she’s rounding it off. Maybe the little machine rounds off. Good. |
Louise |
Or maybe it’s because like, in the diagram, it shows it had the sensor pretty far back. Maybe the transmitted light didn’t go that far. |
In this excerpt, the students began to identify the range of variables that might explain the differences between their outcomes and Lesley’s, including differences in the setup, the materials, the strength of the light source, the device used to record the data, and the scientist’s decisions regarding the reporting of the data. This exchange is significant to the extent that the students demonstrate an appreciation for the role variables play in the design of an investigation. With this understanding, they are now situated to
consider the control of variables that is necessary so that only a single contrast is featured in an experiment.39
One final observation about the successful use of text in inquiry-based instruction is the importance of students assuming a skeptical stance rather than simply deferring to the text. The following three excerpts are illustrative. The first two are examples of instances in which students questioned the generality of Lesley’s claim that “all objects reflect and absorb light.” In the first instance, Kit interjects, “I think that she says ‘all’ too much. Like she could just say ‘most’ or she could test more objects because ‘all’ is kind of a lot because she only tested like, seven.” Ms. Sutton responds, “Okay, so you’re saying you don’t know if she’s tested enough to say ‘all,’ to make that kind of statement.”
The second excerpt begins when one student, Katherine, expresses concern that Lesley has not provided sufficient information about the kinds of materials with which she investigated. This leads a second student, Megan, to observe that the objects with which Lesley investigated are quite similar (i.e., they are all “flat”) and that Lesley should have selected objects with different characteristics if she wished to make the claim that “all objects absorb and reflect light.” Ms. Sutton prompts for more specificity, to which Megan responds, “None of them are kind of like a ball or something that’s 3-D. They’re all, like, flat … because something that’s 3-D … it gets thicker because if you had a green ball and you shine light through, it would be … probably be a darker color because there’s two sides to a ball and not just one.”
In a related criticism, Kit observes that Lesley needed to consider not only the color of the object she was investigating, but also the material of which it was made:
Kit |
I don’t think the claim would be as true if the white [objects] were different materials. |
Ms. Sutton |
Okay, so you would get a—if you had a light meter to measure like she did and you were measuring all the black objects on this list, do you think you still would get different readings? They’d absorb differently? They wouldn’t all absorb the same amount? |
Students |
Yeah … yeah…. |
Ms. Sutton |
How many people agree with that, that all the black objects probably wouldn’t absorb the same amount of light? Okay, so they’re agreeing with you. |
SUPPORTING LEARNING THROUGH CYCLES OF INVESTIGATION
Whether students’ experiences with investigation are first- or second-hand, the outcome of any single cycle of investigation will not result in development of all the targeted knowledge and reasoning goals for a particular topic of study. Thus, inquiry in any topic area requires multiple cycles of investigation. Discussion of how to design curriculum units with cycles of investigation and the interplay between first- and second-hand experiences is beyond the scope of this chapter. The important point is that students need to have multiple opportunities to learn concepts (i.e., multiple cycles of investigation that provide occasions for dealing with the same concepts) and encounter those concepts in multiple contexts (e.g., reflection is studied in contexts with mirrors, as well as in contexts with other opaque objects). The purpose of this section is to discuss how teachers might think about the development of knowledge across cycles of investigation.
The classroom community determines the fate of any knowledge claim generated by a group. Within and across each cycle, knowledge claims are generated, tested, refuted, tweaked, embraced, discarded, and ignored. (Note that the teacher’s guidance is critical to ensure that false claims are not embraced without further exploration and that core claims are understood.) Figure 10-3 illustrates this process. In this case, the class worked with five
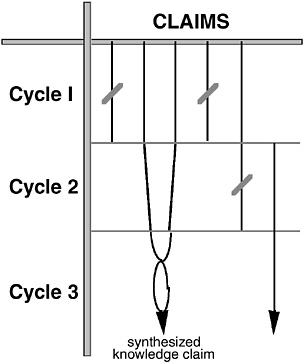
FIGURE 10-3 The development of community knowledge across cycles of investigation.
knowledge claims during Cycle 1 of its investigation. Following the reporting phase, two of these claims were abandoned: one because the child who had initially championed it no longer did so, and the other because there was significant evidence countering it. Three claims survived this first cycle of inquiry: one because there was clear and consistent data supporting it, and the other two because the data were insufficient to make a definitive judgment.
The reporting phase of Cycle 2 of the investigation led to the emergence of a new claim and the abandonment of one of the initial claims because only one of nine groups presented evidence in support of that claim, and the class expressed reservations regarding that group’s data collection procedures. The two remaining claims survived, but were revised in ways that suggested they might be related.
Cycle 3 began with the class considering three extant claims. During the reporting phase, the two claims that appeared to be related became combined and synthesized into one claim. This is a significant development from a scientific perspective given the value placed on simplicity and parsimony of claims about the physical world. The final claim, while still in the running, was not accepted by the class, but neither was it rejected.
This progression of events with the community knowledge claims resulting from each cycle is like threads that when woven together create the fabric of scientific knowledge and reasoning on the topic of study. Some threads will dangle, never fully attended to; some will be abandoned; while others will be central to understanding the topic of study and may need to be blended together to create a strong weave. The fate of each thread is determined by classroom community judgments about which claims have the most evidence, account for the greatest range of data, and are simple and concise; that is, the standards for acceptance are values adhered to by scientists in the production of scientific knowledge. Although it can be difficult for teachers to stand by while students initially make scientifically inaccurate claims, the teacher’s imposition of the constraints of the scientific community’s cultural norms—norms that the students themselves eventually enforce—results in the final set of community claims being scientifically accurate or having indeterminate status with respect to science. Furthermore, whereas dangling threads in a fabric are problematic, they are important to the process of learning science because the reasons for rejecting or abandoning claims form part of the understanding of scientific ways of knowing.
The Development of Conceptual Frameworks
Imagine now that the students have been through several cycles of investigation. What is to prevent these cycles from being experienced as a set
of disconnected experiences, resulting in isolated knowledge? How are the students to develop, elaborate, and refine conceptual frameworks from repeated inquiry experiences? We have argued40 that the “threads that bind” take the form of explicit attention to the relationships among knowledge claims. Conclusions from How People Learn tell us that the formulation of a conceptual framework is a hallmark of developing deep understanding, and that a focus on the development of deep understanding is one of the principles distinguishing school reform efforts that result in increases in student achievement from those that do not.41
The development of organized knowledge is key to the formulation of conceptual frameworks. Developing organized knowledge is enabled by well-designed curriculum materials, but requires specific guidance by teachers as well. Some of that guidance needs to involve pressing students to work from the perspective of the norms for knowledge building in the scientific community. For example, scientists assume that there are regularities in how the world works. If the sky appears gray with no evidence of clouds or the sun, a scientist, who has seen the sun in the sky every other day, will assume that it is still there and infer that something must be blocking it. This perspective dictates different questions than one that does not assume such regularity.
Another area of guidance comes from pressing students to focus on the relationships among the claims they are making. Sorting out these relationships may result in multiple claims being revised into a single claim, as shown in Figure 10-3. Alternatively, revisions may need to be more extensive to fit the expectation of scientists that relationships within a topic area fit together; that is, they are coherent with one another.42 If we claim that light reflects off the front of a mirror but does not appear to reflect off the back, or if we claim that light can go through glass but does not go through a glass mirror, what is the relationship between those ideas? It is not coherent to claim that light does and does not reflect from a mirror. Similarly, it is not coherent to say that light transmits through glass but not through a glass object (i.e., a mirror). Of course, the coherent view is that light is transmitted through glass, but in the case of a mirror, it is transmitted through the glass part but reflects from the backing that is placed on the glass to make it a mirror. To develop these kinds of perspectives, students must learn concepts in combination, with attention to the relationships among them.
Illustration: The Development of Conceptual Frameworks for Light
In this section we trace the development of student understanding about light over four cycles of investigation in Ms. Lacey’s class, guided by the question of how light interacts with matter. This instruction took place over
4 weeks, with each cycle taking about a week of daily instruction. We present concept maps constructed from classroom discourse during the instruction.43 That is, the maps represent the collective knowledge building that would be evident to the teacher and the class. Transcript excerpts accompany the maps to illustrate the nature of the conversation among the students and teacher.
During Cycle 1, students focused on the differences among objects, assuming that light interacted with each object in only one way. During reporting, they made statements such as: “Light can go through glass if it’s clear enough,” “Light reflects off mirrors and shiny materials, too,” and “We had a solid thing here. It just stopped at the object. It didn’t reflect.” Students wrestled with whether claims indicating that light could “be blocked” and “stay in” meant the same thing or something different. Figure 10-4 suggests that students thought light could interact with matter in one of three ways.
The question marks in the figure indicate that some individuals or groups asserted the relationships shown, but not all the students accepted these relationships, including one group that provided evidence that light can interact with an object in two ways—a finding that could have dramatically changed the structure of the class’s knowledge from what is shown in the figure. This particular group did not recognize the significance of its findings, focusing instead on the one way it should categorize objects from which it had observed multiple interactions. In the following excerpt, the teacher encourages the group to think of its results as a new claim.
Kevin |
We saw sort of a little reflection, but we, it had mostly just see-through. |
Ms. Lacey |
So you’re saying that some materials could be in two different categories. |

FIGURE 10-4 Community knowledge from the first cycle of investigation (first-hand).
Derek |
Yes, because some were really see-through and reflection together, but we had to decide which one to put it in. |
Ms. Lacey |
Do you think you might have another claim here? |
Kevin |
Light can do two things with one object. |
With the introduction of the idea that light can interact with matter in more than one way, the students embarked upon a second cycle of investigation with the same materials, with the intent of determining which if any objects exhibited the behavior claimed by Kevin and Derek. From this second round of investigation, all groups determined that multiple behaviors can occur with some objects, but there was uncertainty about whether these interactions occur with some types of materials and not others (see Figure 10-5). Nevertheless, the significance of this day’s findings is that they represent a different conceptual organization from that of the first cycle (see Figure 10-4) to the extent that light is not confined to behaving in only one way. At the same time, the possibilities for the behavior of light have increased significantly, and only the case of four types of interaction has been ruled out in discussion by the community (following interaction comparing what different groups meant by “blocked” versus “absorbed”).
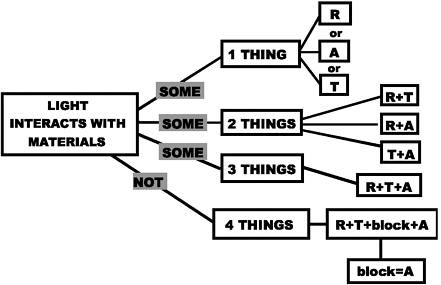
FIGURE 10-5 Community knowledge from the second cycle of investigation (first-hand). R = reflect; T = transmit; A = absorb.
In addition, some students expressed puzzlement about how light could interact with a material in more than one way. In response to this question, one group introduced the idea that there was a quantitative relationship among the multiple behaviors observed when light interacted with an object:
Miles |
If you said that light can reflect, transmit, and absorb, absorb means to block. How can it be blocked … and still go through? |
Corey |
If just a little bit came through, then most of it was blocked. |
Ms. Lacey |
Would you draw him a picture, please? [Corey and Andy draw setup.] |
Corey |
Here’s the light, a little being blocked inside, and a little of it comes out … |
Andy |
Some of it’s reflecting. |
During the third cycle of investigation, in which the students and the teacher interactively read a Lesley Park notebook text about light using reciprocal teaching strategies,44 the students encountered more evidence that light can interact with matter in multiple ways (see Figure 10-6). This led to conversation concerning how general a claim might be made about the behavior of light:
Andy |
Can all objects reflect, absorb, and transmit? Tommy? |
Tommy |
Most of them. |
Andy |
Corey? |
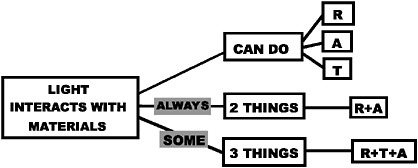
FIGURE 10-6 Community knowledge from the third cycle of investigation (second-hand).
Corey |
Yes, because it says right in here, “Light can be reflected, absorbed and transmitted by the same object.” |
Ms. Lacey |
I think we need to clarify something, because you said one thing, Corey, and Miles said something else. Andy’s question was “Can all objects reflect, absorb, and transmit light?” |
Alan |
No. It just says light can be reflected, absorbed, and transmitted by the same object. It doesn’t say anything about every object. |
Ms. Lacey |
So you say not all can. Do we have any data in our reading that tells us that not all things absorb, reflect, and transmit? |
Tommy |
We have evidence that all objects reflect and absorb [referring to a table in the notebook text]. |
The concept map representing the community’s understanding about light up to this point shows greater specification of the prevalence of relationships (“always” versus “sometimes”) and a narrowing of the possible relationships that can occur when light interacts with matter: light always reflects and is absorbed.
Lesley’s quantitative data about the amount of reflection and transmission of light from an object as measured by a light meter supported additional conversation about the issue of quantitative relationships raised by one group in the previous cycle. However, students did not yet add those ideas to their class claims chart.
In the fourth cycle of investigation, students returned to a first-hand investigation and were now quite comfortable with the idea that light can simultaneously interact with matter in multiple ways. In addition, despite not having tools to compare the brightness of the light, they qualitatively compared the amount of light behaving in particular ways. This is represented in the map in Figure 10-7.
Do all students have the understanding represented in Figure 10-7? The excerpt below suggests that this is unlikely. In this excerpt, a student reveals that he and his partner did not think light would reflect from an object even after the class had established in the previous cycle that light always reflects:
Ms. Lacey |
When you saw the blue felt, is that the claim you first thought? |
Kenny |
Yeah, we learned that this blue felt can do three—reflect, transmit, and absorb—at one, at this one object. And it did. It reflected a little, and transmitted some and it absorbed some. |
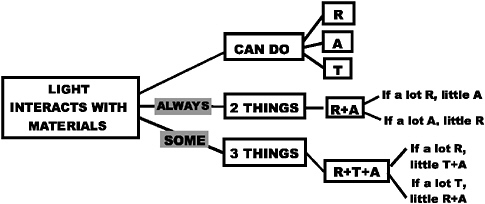
FIGURE 10-7 Community knowledge from the fourth cycle of investigation (first-hand).
Ms. Lacey |
And when you started out, what did you think was going to happen? |
Kenny |
That it was only going to transmit and absorb. We didn’t think it would reflect. |
Ms. Lacey |
What do we know about materials and reflecting? |
Class |
They always reflect and absorb. |
We see the teacher checking on the student’s understanding, which is scientifically accurate. But we know that for such a claim—that light reflects off all materials—many experiences may be needed for that knowledge to be robust. Relationships such as this for which we have no direct experience or that are counterintuitive (we see reflected light from objects, not the objects themselves) take time and attention, as well as recursive tacking to knowledge-building processes and the conceptual framework that is emerging from those processes. Conceptual frameworks that represent the physical world in ways we have not experienced (e.g., the electromagnetic spectrum) or are counterintuitive (light is a particle and a wave) pose even greater challenges to the development of scientific knowledge.
THE ROLE OF SUBJECT-SPECIFIC KNOWLEDGE IN EFFECTIVE SCIENCE INSTRUCTION
At the core of teacher decision making featured in this chapter is the need to mediate the learning of individual students. To do this in a way that leads to targeted scientific knowledge and ways of knowing, teachers must be confident about their knowledge of the learning goals. That is, teachers
must have sufficient subject matter knowledge, including aspects of the culture of science that guide knowledge production, to fully understand the nature of the learning goals. When students say that light “disappears” into paper but reflects off of mirrors, a teacher’s uncertainty about whether that claim is accurate will hamper effective decision making. When students claim an object is opaque and the question at hand is how light interacts with matter, the teacher needs to recognize that the word “opaque” describes the object and not light, and that an opaque object can reflect and absorb light and even transmit some light in certain cases (e.g., a piece of cardboard).
At the same time, having accurate subject matter knowledge is not sufficient for effective teaching. When students claim that light is a gas, it is not sufficient for the teacher to know that light is energy, not a state of matter. The teacher also needs to know what observations of light might convince students that it is not a gas, which in turn is informed by knowing how students think of gases, what their experiences of gas and light have likely been, and what it is possible to observe within a classroom context. This knowledge is part of specialized knowledge for teaching called pedagogical content knowledge because it is derived from content knowledge that is specifically employed to facilitate learning. It is the knowledge that teachers have about how to make particular subject matter comprehensible to particular students.45
Pedagogical content knowledge includes knowledge of the concepts that students find most difficult, as well as ways to support their understanding of those concepts. For example, it is difficult for students to understand that the color of objects is the color of light reflected from them because we are not aware of the reflection. Having students use a white screen to examine the color of light reflected from colored objects can reveal this phenomenon in a way that is convincing to them. Pedagogical content knowledge also includes knowledge of curriculum materials that are particularly effective for teaching particular topics. A still valuable resource for the study of light in the elementary grades is the Optics kit mentioned earlier that is part of Elementary Science Study curriculum materials developed in the 1960s. A teacher’s knowledge of these materials and how they can be used to support knowledge building is key to employing them effectively in mediating student learning.
Finally, pedagogical content knowledge includes ways to assess student knowledge. A classic item to determine students’ understanding of how we see is a diagram with the sun, a tree, and a person looking at the tree.46 Students are asked to draw lines with arrows in the diagram to show how the person sees the tree. Arrows should be drawn from the sun to the tree to the person, but it is not uncommon for students to draw arrows from the sun to the person and the person to the tree. Use of this item at the beginning of a unit of study can provide a teacher with a wealth of information on current
student thinking about how we see, as well as stimulate students to wonder about such questions.
The more teachers know and understand about how their students think about particular concepts or topics of study, how that thinking might develop and unfold during systematic study of the topic, and how they might ascertain what students’ understanding of the topic is at any point in time, the better they are able to optimize knowledge building from students’ varied experiences and support students in developing desired scientific knowledge and ways of knowing. When and how to employ particular strategies in the service of supporting such knowledge building is a different issue, but the topic-specific knowledge for teaching that is identified as pedagogical content knowledge is a necessary element if students are to achieve the standards we have set.
CONCLUSION
Science instruction provides a rich context for applying what we know about how people learn. A successful teacher in this context is aware that he or she is supporting students in activating prior knowledge and in building upon and continuing to organize this knowledge so it can be used flexibly to make sense of and appreciate the world around them. To do this well, the teacher must be knowledgeable about the nature of science, including both the products—the powerful ideas of science—and the values, beliefs, and practices of the scientific community that guide the generation and evaluation of these powerful ideas. Furthermore, teachers must be knowledgeable about children and the processes of engaging them in knowledge building, reflecting upon their thinking and learning new ways of thinking.
We have proposed and illustrated a heuristic for conceptualizing instruction relative to the opportunities and challenges of different aspects of inquiry-based instruction, which we have found useful in supporting teachers in effective decision making and evaluation of instruction. We have argued that the development of scientific knowledge and reasoning can be supported through both first- and second-hand investigations. Furthermore, we have proposed that the teacher draws upon a broad repertoire of practices for the purposes of establishing and maintaining the classroom as a learning community, and assessing, supporting, and extending the knowledge building of each member of that community. All of these elements are necessary for effective teaching in the twenty-first century, when our standards for learning are not just about the application of scientific knowledge, but also its evaluation and generation.
NOTES
1. |
Schwab, 1964. |
2. |
Hapgood et al., in press; Lehrer et al., 2001; Magnusson et al., 1997; Metz, 2004. |
3. |
National Research Council, 2003. |
4. |
These materials, originally developed in the 1960s, can be purchased from Delta Education: http://www.delta-education.com/. |
5. |
Whereas some view conceptual change as referring to a change from existing ideas to new ones, we suggest that new ideas are often developed in parallel with existing ones. The new ideas are rooted in different values and beliefs—those of the scientific community rather than those guiding our daily lives. |
6. |
Chi, 1992. |
7. |
Galili and Hazan, 2000. |
8. |
Our decision to focus on instruction in which investigation is central reflects the national standard that calls for science instruction to be inquiry based. |
9. |
We use the term “guided” inquiry to signal that the teacher plays a prominent role in shaping the inquiry experience, guiding student thinking and activity to enable desired student learning from investigation. |
10. |
Magnusson and Palincsar, 1995. |
11. |
Barnes, 1976; Bybee et al., 1989; Karplus, 1964; Osborne and Freyberg, 1985; Lehrer and Schauble, 2000. |
12. |
All of the instruction featured in this chapter was conducted by teachers who were a part of GIsML Community of Practice, a multiyear professional development effort aimed at identifying effective practice for inquiry-based science teaching. |
13. |
This discussion draws on a study focused on children’s self-regulation during science instruction, which took place in a school in a relatively small district (about 4,600 students) that includes a state university. Approximately 45 percent of the students in this district pass the state standardized tests, and 52 percent are economically disadvantaged. |
14. |
This class is in a school in a relatively small district (about 3,000 students) near a major industrial plant in a town with a state university. Approximately 38 percent of the students in this district pass the state standardized tests, and 63 percent are economically disadvantaged. |
15. |
While we are featuring contexts in which there is a single question, teachers could choose to have a context in which children are investigating different questions related to the same phenomenon. However, it is important to recognize the substantially greater cognitive and procedural demands this approach places on the teacher, so it is not something we recommend if a teacher is inexperienced in conducting inquiry-based instruction. |
16. |
Although it can be motivating and conceptually beneficial for students to be placed in the role of generating questions for investigation, the teacher needs to be mindful of the consequences of taking time to investigate questions that may be trivial or peripheral to the unity of study. The teacher may judge the time to be useful as students can still learn a great deal about investigation, but |
REFERENCES
Barnes, D. (1976). From communication to curriculum. Hammondsworth, UK: Penguin Books.
Blumenfeld, P.C., and Meece, J.L. (1988). Task factors, teacher behavior, and students’ involvement and use of learning strategies in science. Elementary School Journal, 88(3), 235-250.
Brown, A.L., and Campione, J.C. (1994). Guided discovery in a community of learners. In K. McGilly (Ed.), Classrooms lessons: Integrating cognitive theory and classroom practice (pp. 229-272). Cambridge, MA: MIT Press.
Bybee, R.W., Buchwald, C.E., Crissman, S., Heil, D.R., Kuerbis, P.J., Matsumoto, C., and McInerney, J.D. (1989). Science and technology education for the elementary years: Frameworks for curriculum and instruction. Washington, DC: National Center for Improving Science Education.
Campanario, J.M. (2002). The parallelism between scientists’ and students’ resistance to new scientific ideas. International Journal of Science Education, 24(10), 1095-1110.
Chi, M.T.H. (1992). Conceptual change within and across ontological categories: Examples from learning and discovery in science. In R. Giere (Ed.), Cognitive models of science: Minnesota studies in the philosophy of science (pp. 129-186). Minneapolis, MN: University of Minnesota Press.
Clement, J. (1993). Using bridging analogies and anchoring intuitions to deal with students’ preconceptions in physics. Journal of Research in Science Teaching, 30, 1241-1257.
Crawford, S.Y., Hurd, J.M., and Weller, A.C. (1996). From print to electronic: The transformation of scientific communication. Medford, NJ: Information Today.
Driver, R., Asoko, H., Leach, J., Mortimer, E., and Scott, P. (1994). Constructing scientific knowledge in the classroom. Educational Researcher, 23(7), 5-12.
Eaton, J.F., Anderson, C.W., and Smith, E.L. (1984). Students’ misconceptions interfere with science learning: Case studies of fifth-grade students. Elementary School Journal, 84, 365-379.
Einstein, A. (1950). Out of my later years. New York: Philosophical Library.
Ford, D. (1999). The role of text in supporting and extending first-hand investigations in guided inquiry science. Dissertation Abstracts International, 60(7), 2434.
Galili, I., and Hazan, A. (2000). Learners’ knowledge in optics: Interpretation, structure and analysis. International Journal of Science Education, 22(1), 57-88.
Hapgood, S., Magnusson, S.J., and Palincsar, A.S. (in press). Teacher, text, and experience: A case of young children’s scientific inquiry. Journal of the Learning Sciences.
Herrenkohl, L., Palincsar, A.S., DeWater L.S., and Kawasaki, K. (1999). Developing scientific communities in classrooms: A sociocognitive approach. Journal of the Learning Sciences, 8(3-4), 451-494.
Karplus, R. (1964). Theoretical background of the science curriculum improvement study. Berkeley, CA: Science Curriculum Improvement Study, University of California.
Klahr, D., Chen, Z., and Toth, E. (2001). Cognitive development and science education: Ships that pass in the night or beacons of mutual illumination? In S. Carver and D. Klahr (Eds.), Cognition and instruction: Twenty-five years of progress (pp. 75-120). Mahwah, NJ: Lawrence Erlbaum Associates.
Lehrer, R., and Schauble, L. (2000). Modeling in mathematics and science. In R. Glaser (Ed.), Advances in instructional psychology, vol. 5: Educational design and cognitive science (pp. 101-159). Mahwah, NJ: Lawrence Erlbaum Associates.
Lehrer, R., Schauble, L., and Petrosino, A.J. (2001). Reconsidering the role of experiment in science education. In K. Crowley, C.D. Schunn, and T. Okada (Eds.), Designing for science: Implications from everyday, classroom, and professional settings. Mahwah, NJ: Lawrence Erlbaum Associates.
Magnusson, S.J., and Palincsar, A.S. (1995). The learning environment as a site of science education reform. Theory into Practice, 34(1), 43-50.
Magnusson, S.J., and Palincsar, A.S. (in press-a). The application of theory to the design of innovative texts supporting science instruction. In M. Constas and R. Sternberg (Eds.), Translating educational theory and research into practice. Mahwah, NJ: Lawrence Erlbaum Associates.
Magnusson, S.J., and Palincsar, A.S. (in press-b). Learning from text designed to model scientific thinking. In W. Saul (Ed.), Crossing borders in literacy and science instruction: Perspectives on theory and practice. Newark, DE: International Reading Association.
Magnusson, S.J., Templin, M., and Boyle, R.A. (1997). Dynamic science assessment: A new approach for investigating conceptual change. Journal of the Learning Sciences, 6(1), 91-142.
Magnusson, S.J., Borko, H., and Krajcik, J.S. (1999). Nature, sources, and development of pedagogical content knowledge for science teaching. In J. Gess-Newsome and N. Lederman (Eds.), Science teacher knowledge. Dordrecht, The Netherlands: Kluwer Academic.
Magnusson, S.J., Palincsar, A.S., and Templin, M. (in press). Community, culture, and conversation in inquiry-based science instruction. In L. Flick and N. Lederman (Eds.), Scientific inquiry and the nature of science: Implications for teaching, learning, and teacher education. Dordrecht, The Netherlands: Kluwer Academic.
Metz, K.E. (2004). Children’s understanding of scientific inquiry: Their conceptualization of uncertainty in investigations of their own design. Cognition and Instruction, 22(2), 219-290.
Mortimer, E.F. (1995). Conceptual change or conceptual profile change? Science and Education, 4, 267-285.
National Research Council. (1996). National science education standards. National Committee on Science Education Standards and Assessment, Center for Science, Mathematics, and Engineering Education. Washington, DC: National Academy Press.
National Research Council. (2003). Learning and instruction: A SERP research agenda. M.S. Donovan and J.W. Pellegrino (Eds.), Panel on Learning and Instruction, Strategic Education Research Partnership. Washington, DC: The National Academies Press.
Newmann, F.M., Marks, H.M., and Gamoran, A. (1995). Authentic pedagogy: Standards that boost student performance. (Issue Report #8, Center on Organization and Restructuring of Schools.) Madison, WI: Wisconsin Center for Educational Research, University of Wisconsin.
Osborne, R. (1983). Towards modifying children’s ideas about electric current. Research in Science & Technological Education, 1(1), 73-82.
Osborne, R., and Freyberg, P. (1985). Learning in science: The implications of children’s science. Portsmouth, NH: Heinemann.
Palincsar, A.S., and Brown, A.L. (1984). Reciprocal teaching of comprehension-fostering and monitoring activities. Cognition and Instruction, 1, 117-175.
Palincsar, A.S., and Magnusson, S.J. (2001). The interplay of first-hand and text-based investigations to model and support the development of scientific knowledge and reasoning. In S. Carver and D. Klahr (Eds.), Cognition and instruction: Twenty-five years of progress (pp. 151-194). Mahwah, NJ: Lawrence Erlbaum Associates.
Palincsar, A.S., Anderson, C.A., and David, Y.M. (1993). Pursuing scientific literacy in the middle grades through collaborative problem solving. Elementary School Journal, 93(5), 643-658.
Palincsar, A S., Magnusson, S.J., and Hapgood, S. (2001). Trafficking ideas through the rotaries of science instruction: Teachers’ discourse moves and their relationships to children’s learning. Paper presented at the annual meeting of the American Educational Research Association, Seattle, WA.
Schwab, J.J. (1964). Structure of the disciplines: Meanings and significances. In G.W. Ford and L. Pugno (Eds.), The structure of knowledge and the curriculum (pp. 6-30). Chicago, IL: Rand McNally.
Wilson, S.M., Shulman, L.S., and Richert, E.R. (1988). ‘150 different ways’ of knowing: Representations of knowledge in teaching. In J. Calderhead (Ed.), Exploring teachers’ thinking. New York: Taylor and Francis.