5
Human Health Risk Assessment in the Coeur d’Alene River Basin
INTRODUCTION
The objective of this chapter is to present an overview of the manner in which a human health risk assessment (HHRA) is conducted and then to describe in stepwise fashion the procedures that the U.S. Environmental Protection Agency (EPA) and its partners followed in conducting the Coeur d’Alene River basin HHRA (TerraGraphics et al. 2001). The Coeur d’Alene River basin HHRA for the area extending from Harrison to Mullan, Idaho, was jointly prepared by the Idaho Department of Health and Welfare (IDHW), the Idaho Department of Environmental Quality, and EPA Region 10. Oversight and guidance were provided by the Governor’s Advisory Council on Human Health Risk Assessment, which included the Lieutenant Governor of Idaho. The five-member EPA Technical Review Workgroup for lead ultimately conducted an independent review of the document. Finally, numerous citizens, tribal representatives and community organizations provided or facilitated reviews and comments of a public draft of the document. Below, we summarize and critique the outcome of that effort. It should be noted that issues that the committee considered as the most important are emphasized in the review. A comprehensive and exhaustive review of all assumptions used in EPA’s assessments and their underlying scientific basis was beyond the scope of what the committee could be expected to accomplish.
General Objectives of an HHRA
The objectives of an HHRA are two-fold: first, to estimate the level of risk to human health associated with concentrations of environmental contaminants; and second, if that risk is found to be unacceptable, to calculate media-specific cleanup levels that will protect human health.
Risks are estimated for current uses of a site as well as foreseeable future uses. All contaminated media are considered (for example, soil, water) if individuals are likely to be exposed to the media. All relevant routes of exposure are also considered, including direct contact, such as inhalation, ingestion, and dermal exposure, and indirect contact, such as exposure to vegetables that have taken up contaminants through the soil or water.
Cleanup levels are calculated based on the relationship between contaminants and risk as defined in the risk assessment and a policy decision (risk management) about the level of risk that is considered acceptable. As a result, cleanup levels for a single contaminant can vary from one site to another either because the relationship between environmental levels and risk differs or because different policy decisions have been made concerning the level of acceptable risk.
Overview of the Superfund HHRA Process
HHRA typically is described as including four steps: hazard identification, exposure assessment, toxicity assessment, and risk characterization. Early in the development of the field of risk assessment, hazard identification referred to determining which chemicals or compounds at a site could lead to risk. Today, the list of chemicals and compounds with associated human health risks are well known, and the first step has changed to data collection and analysis, including collecting data on the characteristics of the site and the chemicals or compounds of concern.
The second step in HHRA involves exposure assessment, including identifying the populations of individuals exposed to hazards at the specific site and how those exposures may occur. For example, the Coeur d’Alene River basin HHRA identifies children as the primary population of concern for lead exposure and identifies the presence of local American Indian populations. Potential pathways of exposure are defined, such as children ingesting soil and house dust contaminated with lead, and American Indian ingestion of locally grown foods contaminated with lead. At other sites, exposures could include scenarios such as inhalation and dermal exposure to volatile chemicals in groundwater while showering. In addition to identifying the potential pathways of exposure, this step may involve defining several parameters (for which there are insufficient measured data) that will
govern the estimated risk from each exposure pathway. These are often referred to as assumptions, or default values, and they are assumed to be representative of a population, although they often include a conservative safety factor. These parameters include things such as time spent indoors and outdoors, which can differ as a function of climate.
The third step is toxicity assessment, or identifying and quantifying a chemical’s or compound’s intrinsic toxic properties. Again, at this point in the development of risk assessment, based on numerous controlled animal and/or human experiments and on epidemiological studies, toxicity parameters have been established by EPA and other agencies for many of the major chemicals and compounds. At times, when a great deal of information is known about a compound’s toxicity, this step involves examining an EPA database for the chemical-specific cancer slope factor (SF) or reference dose. But for many compounds found at Superfund sites, much less is known, and there are myriad assumptions made that often prove very controversial.
The fourth step, risk characterization, combines the results of the first three steps into an estimate of risk. The estimated risk is then compared with a level of risk deemed “acceptable” according to risk management decisions (see below), and the site is thereby identified as either having acceptable risk levels or in need of remedial measures.
All the risk assessment steps described above inherently incorporate uncertainty. Each of the steps generally involves extrapolation from observations in one set of circumstances (for example, the effect of known, high doses of a chemical given to laboratory animals over a short period) to the circumstances of interest (for example, the potential effects of unknown, small doses of a mixture including the tested chemical on humans over a lifetime). Each such extrapolation introduces qualitative and quantitative uncertainties; and an adequate HHRA should describe qualitatively—and, if possible, quantitatively the sizes and types of such uncertainties.
One additional tenet of the Superfund HHRA process bears discussion, and that is EPA’s preferred focus on the individual with reasonable maximum exposure (RME). A risk assessment generally includes a calculated estimate of the likely risks for an average individual—the central tendency (CT)—and for an individual experiencing RME conditions. EPA defines RME as the highest exposure that is reasonably expected to occur at a site. Generally, the RME risk is compared with the acceptable level of risk when determining whether remedial measures are needed.
If risks are found to be unacceptable, thus requiring remediation, then the models used in the risk assessment can also be used to determine acceptable concentrations of contaminants, equated to “cleanup levels.” It is important to note that a cleanup level calculated in this way is applicable over the same geographic area that was assessed in the risk calculation and
represents the same mathematical formulation used for the concentration term in the risk assessment. For example, if the chronic risk to a child exposed over several years to the average contaminant concentration in his/ her yard is found to be unacceptable, then a cleanup level derived from the corresponding risk equation will represent the acceptable average concentration for soil in the yard. As a further example, if a risk calculation focused solely on a heavily used play area finds unacceptable risk, then the cleanup level calculated from that risk equation will represent the acceptable average concentration for the play area. However, the derivation of an actual cleanup level is typically controversial, partly due to the uncertainties associated with each piece of information that go into the mathematical derivation of the cleanup number.
Finally, a distinction needs to be drawn between risk assessment and risk management. Simply put, risk assessment is scientific and involves identifying pathways of exposure and some mathematical calculations; risk management involves policy and societal values. Cleanup levels are calculated on the basis of a policy decision about the level of acceptable risk as well as on the basis of the mathematical risk assessment. Further, the assessment of uncertainty in a risk assessment may lead to the development of more than one possible cleanup level or a range of cleanup levels. A risk manager will choose a cleanup level from the range after considering other site characteristics such as technical feasibility of the remediation, public desires, and so forth. As a result, a cleanup level may not be directly linked to an actual risk calculation, but it is generally expected that the cleanup level chosen during the risk management process will fall within a range developed in the course of the risk assessment.
Geographic Area Considered in the Coeur d’Alene River Basin HHRA
The Coeur d’Alene River basin HHRA considered an area that included the South Fork of the Coeur d’Alene River, its tributaries, and the main stem of the river west of its confluence with the North Fork. The region of interest spans roughly 53 miles from the Idaho-Montana border to Lake Coeur d’Alene and excluded the 21-square-mile Bunker Hill Superfund site. The towns of Mullan, Osburn, Wallace, and parts of Pinehurst, Idaho, are all included and all lie within Shoshone County.
Demographics of the Population
The demographic characteristics of the Coeur d’Alene River basin are primarily a function of its mining past and were strongly affected by the closure of the Bunker Hill smelter in 1981. Since the smelter ceased operations, the region has suffered chronically high unemployment, averaging
12.3% in the 1990s, about twice the state average. In 2001, the per capita income was just over $19,000, or 78% of the state value (Idaho Department of Commerce 2004). The lower wage base is accompanied by an increase in poverty; according to the 2000 U.S. census, 12.4% of the families and 16.4% of the individuals in rural Shoshone County lived below the poverty level during 1999. These values were higher than the statewide values of 8.3% and 11.8%, respectively. With the lack of a viable economic base, there has been a gradual out-migration of people from Shoshone County; due to limited turnover of the population, the county’s age and racial profiles do not generally reflect those of the state as a whole. For example, the median age for Idaho was 33 years in 2000, but in the mining communities of the river basin, it was over 40 years. Racially, the county’s population of 13,771 was predominantly white (96% white versus 93% for Idaho), with small American Indian (1.5%) and Hispanic populations (1.9%) versus 2.1% and 7.9%, respectively, statewide. The total population of the river basin areas addressed in the HHRA was 10,496 based on 1990 census data (TerraGraphics et al. 2001, Table 3-4). Children aged 0 to 4 years—a population cohort that is particularly susceptible to lead toxicity—made up 5.6% of the population (587 children).1
CHEMICALS OF CONCERN IN THE COEUR D’ALENE RIVER BASIN: HAZARD IDENTIFICATION
The database of environmental chemical analyses available for the HHRA process was extensive and included thousands of analyses of metals in soil, house dust, groundwater, homegrown vegetables, sediment, surface water, fish, and edible wild plants (water potatoes) in the river basin. Typically, for each sample, the precise geographic location and concentrations of up to 23 metals and other inorganic materials were ascertained. For example, 4,000 soil and sediment samples were collected within the study area and analyzed for 23 inorganic compounds. Yard soils from 1,020 homes throughout the river basin were analyzed for lead, corresponding to roughly one-quarter of the yards present in the river basin in the 1990 census. Soils from 191 residential yards were analyzed for 23 inorganic compounds. Before chemical analysis, all soil samples were sieved to obtain soil particles less than 175 micrometer (μm) in diameter. Pre-sieving is justified by the observation that fine particles preferentially adhere to hands (Duggan et al. 1985; Duggan and Inskip 1985; Sheppard and Evenden 1994; Kissel et al. 1996) and the assumption that they are therefore more likely to be ingested. Dust mats were placed and collected from 500 river basin homes, and vacuum cleaner bags
were collected from 320 of those homes. Measurements of these samples allowed for estimates of both lead concentration and dust loading rates. Tap water from 100 homes was analyzed for 23 inorganic compounds, and 425 homes had water lead analyzed. Eighty samples of water from 27 monitoring wells near Ninemile and Canyon Creeks were analyzed for 23 inorganic compounds. X-ray fluorescence measurements of lead concentrations on interior and exterior surfaces were performed in 415 homes. While this tabulation could go on, the point is that a substantial environmental database was available to the risk assessors as they sought to quantify chemicals of concern from a variety of media in the Coeur d’Alene River basin environment that might pose a risk to human health. Because of the large geographic area of the river basin, additional studies of specific areas will be required as remediation proceeds.
Not all substances present at various test sites pose a human health risk. For example, some of the numerous metals present in environmental samples from the river basin are essential nutrients, including zinc, calcium, iron, magnesium, potassium, and sodium. Yet even these, in excess, can pose health risks. Thus, EPA has developed guidelines for selecting a group of chemicals of potential concern (COPCs) based on their toxicity, concentration, and other factors (EPA 1989). Typically, applicable or relevant and appropriate requirements (ARARs) are used to compare the observed concentration of a substance in an environmental sample with some screening value, threshold, or legally defined concentration in that environmental medium. For example, the ARARs for drinking water at this site are actually the EPA maximum contaminant levels (MCLs)—concentrations of substances in drinking water above which unacceptable health risks to the public may occur. The ARARs for surface water are the MCLs as well as the ambient water-quality criteria (AWQC). The latter, used for controlling releases or discharges of pollutants, are protective of those who drink surface water, those who eat fish caught in surface water, and aquatic organisms. The only ARAR for substances in air that is relevant at this site is that for lead—the National Ambient Air Quality Criterion for lead. There are no ARARs at this site for substances in soil or sediments.
The river basin HHRA considered which COPCs might pose a human health risk for each medium of possible exposure: soil/sediment, tap water, surface water, groundwater, house dust, air, fish consumption, and homegrown vegetables. The process used was very typical of any HHRA at sites where chemical exposures might occur. In addition, it considered possible risks due to the ingestion of water potatoes, a culturally important food source for the Coeur d’Alene tribe. Because a “screening value” for substances in water potatoes is not known, cadmium and lead were evaluated as substances with possible risk, a decision consistent with the evaluation of other food substances.
As a result of these hazard-identification activities, selected metals were chosen for further evaluation of human exposure, and a list of possible sources of exposure was created for each (Table 5-1). The metals were antimony, arsenic, cadmium, iron, lead, manganese, mercury, and zinc.
In summary, the HHRA appropriately identified COPCs for each possible source of exposure. However, no effort was made to identify the particular chemical species of lead or arsenic (or other metal) in any of these sources. The absence of chemical speciation is less than ideal because the bioavailability and toxicity of particular chemical species of the same metal can vary substantially.
APPROACH USED TO ASSESS HUMAN HAZARDS: EXPOSURE ASSESSMENT
After identifying which chemicals might pose hazards to human health, the HHRA set out to characterize human exposure. Because the concentrations of metals in various media and exposure profiles in the river basin are not uniform, EPA considered it necessary to divide the region of interest into nine distinct geographical areas: lower basin, Kingston, side gulches, Osburn, Silverton, Wallace, Ninemile, Mullan, and Blackwell Island (TerraGraphics et al. 2001, Fig. 3-1a). For each of these regions, diagrams were created to conceptualize possible pathways of exposures to metals that might occur under several scenarios—for example, during residence in the home, neighborhood recreation, public recreation, occupation, and subsistence living. An example of this approach, for Silverton, Idaho, taken directly from the HHRA, is provided as Figure 5-1 (TerraGraphics et al. 2001). This portion of the HHRA was basically a paper exercise, but one that is based on a rather extensive literature that has documented that such pathways of exposure have resulted in significant chemical exposures in
TABLE 5-1 Possible Exposure Sources of Chemicals of Potential Concern
Possible Exposure Source |
Chemicals of Potential Concern |
Soil/sediment |
Antimony, arsenic, cadmium, iron, lead, manganese, and zinc |
Tap water |
Arsenic and lead |
Surface water |
Arsenic, cadmium, lead, manganese, and mercury |
Groundwater |
Antimony, arsenic, cadmium, lead, and zinc |
House dust |
Antimony, arsenic, cadmium, iron, lead, manganese, and zinc |
Fish |
Cadmium, lead, and mercury |
Homegrown vegetables |
Arsenic, cadmium, and lead |
SOURCE: TerraGraphics et al. 2001, Table 2-12. |
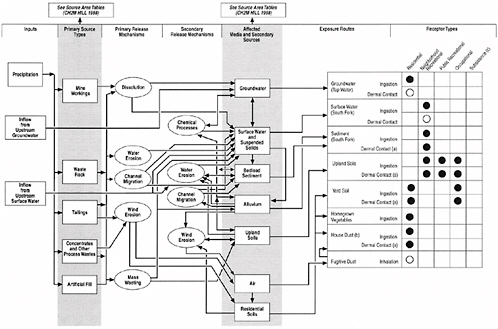
FIGURE 5-1 Example of conceptual site model diagram from HHRA. ●, Complete pathway; evaluated quantitatively in the HHRA. ◯, Pathhway potentially complete, but of minor concern; not qualified in the HHRA. A blank cell indicates that the pathway is not complete or the receptor type does not exist in the this area. (a) Quantified for arsenic and cadmium only. See text for discussion. (b) This pathway evaluated quantitatively for nonlead COPCs and quantitatively for lead. (c) Both traditional and modern subsistence tribal member exposure scenarios will be evaluated at a later time.
other circumstances. Thus, this approach represents an acceptable technique for eventually estimating potential current and future exposures.
Ultimately, to estimate possible risks of adverse health outcomes, it is necessary to estimate the metal concentration in each environmental medium to which an individual may be exposed. EPA guidelines (EPA 1991a, 1992a) state that this concentration term (exposure point concentration [EPC]) should represent the average concentration to which one is exposed for the relevant portion of one’s lifetime. Because of the obvious uncertainty in estimating the true average concentration from measurements of samples, EPA recommends using the 95% upper confidence limit (UCL95) of the mean as a conservative estimate of the EPC, because this is associated with only a 5% probability of underestimating the true average (EPA 1991a, 1992b, 1993a). In addition to the concentrations in each environmental medium, it is necessary to estimate the pathway-specific intakes from that medium to ultimately estimate exposures. In the Coeur d’Alene River basin HHRA, intakes were estimated in two ways, consistent with EPA guidelines for risk characterization (EPA 1995). A CT exposure estimate is considered to be representative of average human exposures, whereas a higher value, the RME, illustrates a high-exposure scenario that is nevertheless likely to occur.
For each of the nine geographic regions, the Coeur d’Alene River basin HHRA used this approach to estimate point concentrations and intakes of surface soil, vacuum bag dust, floor mat dust, tap water, groundwater, subsurface soil, waste piles, and sediments. A total of 49 data sets were analyzed rather than 72 (nine regions × eight sources) because not every region had potential exposure from each of these sources. In 38 of 49 cases, at least 10 measured values were available to make this estimate, and in many cases, hundreds of measurements were used, thus providing stable estimates of the true average concentration. In the remaining 11 cases, fewer than 10 measurements were available; in these cases, the maximum value was used in place of the UCL95. Because the formula used to appropriately calculate UCL95s depends on the distribution of the data, the HHRA first examined the shape of the distributions before carrying out these calculations.
Regional estimates of chemical intakes were subsequently made for soil, sediment, drinking water, surface water, homegrown vegetables, and fish. The exposure models utilized were straightforward and took into account a variety of behavioral and physiological factors, including exposure frequency and duration, contact rate, EPC, body weight, and averaging time. An example of one of these models, derived from the HHRA, which estimated exposure via the consumption of groundwater as a drinking source, is shown below:

(1)
and

(2)
where
Cw = chemical concentration in groundwater/tap water (μg/L);
SIFw = summary intake factor for ingestion of tap water (L/kg/day);
IRw = ingestion rate for tap water (L/day);
EF = exposure frequency (days/year);
ED = exposure duration (years);
CF = conversion factor (mg/μg);
BW = body weight (kg); and
AT = averaging time (days).
The intake parameters used to solve such equations (in this case, IRw, EF, ED, BW, and AT) for children and adults were obtained from previous EPA guidance for such calculations (EPA 1989, 1991a, 1993a). In the example presented, the intake parameters are known with a relatively high degree of certainty (for example, ingestion rate for tap water). In other equations, such as those related to exposure from homegrown vegetables or dermal exposure to surface water, intake parameters are less certain (for example, vegetable ingestion rates, and gastrointestinal and dermal absorption factors) but represent conservative estimates of the weight of current scientific evidence.
HUMAN HEALTH: TOXICITY ASSESSMENT
After identifying the chemical hazards and estimating the human exposures to each, the next step in an HHRA involves evaluating the scientific evidence from animal and human epidemiologic studies that have examined dose-response relationships for cancer and noncancer health outcomes. The fundamental tenet of toxicology is that the dose determines the effect.
For Carcinogens (Arsenic)
For cancer outcomes, the dose-response information is condensed into an SF, in units of (mg/kg-day)−1, which expresses excess cancer risk as a function of (lifetime average) daily dose. EPA maintains an online database, the Integrated Risk Information System (IRIS) (EPA 2004a), which contains SFs that are based on the current weight of toxicologic evidence. Of the metals identified as potential hazards in the river basin, only arsenic was
evaluated for carcinogenic risk.2,3 Arsenic’s SF—unchanged since the early 1990s—is based largely on data from international epidemiologic studies that have been reviewed in previous National Research Council (NRC) reports (NRC 1999, 2001). Several U.S.-based studies have failed to find an association between arsenic in drinking water and cancer risk in non-smokers (Bates et al. 1995; Lewis et al. 1999; Karagas et al. 2001; Steinmaus et al. 2003), possibly suggesting that the SF may overstate the risks at low doses. In this regard, however, a recent study of arsenic and bladder cancer in New Hampshire that examined individual arsenic exposures using toenail arsenic as a biomarker of exposure found that low-level arsenic exposure was associated with a doubling of the risk for bladder cancer (Karagas et al. 2004). At the present time, a great deal of research concerning arsenic and cancer is ongoing, much of it supported by the Superfund Basic Research Program, and it seems possible that the SF may need to be reexamined in the future as a result of past and ongoing work.
For Noncarcinogens Other Than Lead
For noncancer outcomes, a chronic reference dose (RfD) is derived from the no-observed-adverse-effect level (NOAEL) or lowest-observed-adverse-effect level (LOAEL) in animals or humans.4 RfDs are derived by dividing the NOAEL or LOAEL by an uncertainty factor that represents a combination of various sources of uncertainty associated with the database for that particular chemical. Once again, EPA’s IRIS database served as a source of RfDs for the chemicals of concern in the basin, except for lead
(discussed below) and iron, for which there is no IRIS RfD and for which other sources of toxicity data were used. Note that arsenic also has non-cancer effects and its own IRIS RfD.
For Lead
Of all the metals of potential concern, the adverse health effects of lead are best characterized in human populations. Risk assessments for lead therefore differ from those for other noncarcinogens in that they rely on observed or predicted blood lead levels (BLLs) because blood lead concentrations have been directly related to adverse outcomes in adults and children. In studies conducted around the world, population average blood lead concentrations have been found to be associated with adverse effects on average measures of cognitive and behavioral development in young children. In short, dose-response relationships between blood lead and adverse health outcomes in children are sufficiently well described that community BLLs can be used to estimate risk. Community BLLs can be determined precisely through appropriately designed surveys, or they can be estimated from environmental data through modeling techniques. The estimation of BLLs through modeling, which involves environmental rather than biological measurements, is considered in Chapter 6.
RISK CHARACTERIZATION
Risk characterization, the last step in an HHRA, strives to combine the estimates of chemical exposure with the estimates of potential human hazard (based on known dose-response relationships) to estimate the actual or potential risks to human health at the site. At the Coeur d’Alene River basin site, EPA estimated cancer and noncancer health risks for both CT and RME conditions. As mentioned above, the CT estimate represents an average level of chemical exposure, while the RME is a more conservative estimate intended to be the highest exposure that can reasonably be expected to occur. Risks were estimated separately for different segments of the population, such as children, adults, and those with occupational exposure.
For Carcinogens
The probability of developing cancer due to arsenic exposure, the only carcinogen assessed, was estimated by a standard approach that involved multiplying the arsenic SF by the estimated arsenic daily intake.
Cancer risk = chemical intake (mg/kg-day) × SF (mg/kg-day)–1.
EPA’s target “acceptable” excess cancer risk is between 10−6 and 10−4 in a lifetime (EPA 1991b). In the HHRA, the method for estimating cancer risk due to estimated arsenic exposure involved multiplying estimated arsenic intakes (in different age groups within different geographic regions) by the arsenic SF. Under RME conditions, cancer risks exceeded 10−6 for each scenario in each of the nine geographic regions. Under RME conditions, residents of the side gulches had cancer risk estimates exceeding 10−4. Under CT conditions, several of the regions also had cancer risk estimates greater than 10−6. Collectively, these findings indicate that arsenic in the side gulches must be dealt with by risk managers. The analysis in the HHRA indicated that exposure to yard soils was the primary driver of arsenic cancer risk in residential scenarios, and that, in the side gulches, tap water also contributed significantly to cancer risk. It should also be noted that cancer risk for the 90th percentile background soil level of 22 mg/kg arsenic in the upper basin is associated with an estimated cancer risk greater than 10−6 using the risk assessment methodology employed in the basin.5
Modern tribal subsistence scenarios yielded cancer risk estimates similar to those for the highest nontribal residential exposures, but traditional subsistence scenarios had risks roughly 10 times higher. During visits to the river basin, the committee learned from tribal leaders that tribal members no longer practice subsistence living in the basin (CDA Resolution 42 [2001]). Nevertheless, risk managers need to address the tribe’s concerns should their members engage in subsistence activities.
For Noncarcinogens Other Than Lead
Methods used for characterizing risks differ for carcinogens and non-carcinogens. For noncarcinogens other than lead, a hazard quotient (HQ) is derived by dividing the estimated total daily exposure to a chemical by the RfD. If the average daily intake exceeds the RfD (if the HQ is greater than 1), there is a potential for risk for an adverse noncancer health outcome:

The river basin HHRA estimated HQs separately for children and adults; in general, children were found to have higher HQs because they are likely to ingest more soil/dust relative to their body weight. For CT exposures to nontribal residents, the only potentially unacceptable hazards would
occur if future residents of the Burke/Nine Mile area were to use groundwater as a source for drinking water. In general, however, soil rather than drinking water contributed most to the HQs. Several other estimated HQs exceeded 1 and indicated possible hazards from the following sources: cadmium from homegrown vegetables and/or water potatoes, iron from soil/sediment ingestion in the lower basin, hypothetical exposure to cadmium and zinc from consumption of groundwater in the Burke/Nine Mile area, and mercury exposure from fish for the traditional subsistence scenario. Although the possible health risks associated with these scenarios should not be ignored, the committee believes that the primary area of focus for risk managers does not lie with these metals. Clearly, other than lead, arsenic is the chemical of potential concern that was consistently a risk driver for all non-lead risk assessment scenarios, with the major source being soil.
Risk assessment of non-lead COPCs appeared generally to follow EPA guidelines. Residential soil EPCs in the basin sub-areas were computed by lumping data from multiple residences—rather than on a residence-specific basis, which is probably more common. The fraction of ingested soil that a child typically obtains from areas other than his or her own yard is essentially unknown. The consequences of using area-wide rather than residence-specific EPC values will depend upon within-residence and across-residence variance in soil concentration. The committee did not have residence-specific soil arsenic data (the soil contaminant of greatest concern in this context) and did not investigate this question.
For Lead
As mentioned above, risk assessments for lead rely on observed or predicted BLLs in a community, as blood lead concentrations have been directly related to adverse outcomes in adults and children. In 1991, the U.S. Centers for Disease Control and Prevention (CDC) promulgated specific guidelines aimed at reducing BLLs in individual children (CDC 1991). These are summarized in Table 5-2.
Because vast quantities of lead have been distributed throughout the river basin due to historical mining-related activities, the HHRA devoted substantial effort to characterizing the risks of lead toxicity to the basin communities, and to children in particular. At sites like this one, EPA policies seek to protect the health of the most vulnerable populations, namely children and women of childbearing age. EPA policy (EPA 1994) strives to reduce soil lead levels so that no child would have more than a 5% chance of exceeding a BLL of 10 micrograms per deciliter (μg/dL). EPA has promoted use of the integrated exposure uptake biokinetic (IEUBK) model for estimating risks to children from lead exposure from soil and
TABLE 5-2 CDC Guidelines for Reducing Blood Lead in Children
Blood lead (μg/dL) |
Action |
<10 |
Reassess or rescreen in 1 year |
10-14 |
Family education; follow-up testing; social services if warranted |
15-19 |
Family education; follow-up testing; social services if warranted; if blood lead persists or rises within 3 months, proceed as below for blood lead concentrations of 20-44 μg/dL |
20-44 |
Provide clinical management, environmental investigation, and lead hazard control |
45-69 |
Immediately begin coordination of care, clinical management, environmental investigation, and lead hazard control |
≥70 |
Hospitalize and treat immediately with chelating agents; environmental investigation and lead hazard control immediately |
SOURCE: CDC 1991. |
other media. The charge to this committee included several questions specifically directed at the IEUBK model. Thus, Chapter 6 is devoted to use of the IEUBK model to understand lead exposure and uptake. The use of the model in this HHRA has projected significant risks of lead toxicity throughout the Coeur d’Alene River basin.
PLAUSIBLE HEALTH RISKS FROM LIVING IN THE COEUR D’ALENE RIVER BASIN
If we assume that the Coeur d’Alene River basin HHRA is correct and that without significant remedial actions, the populations of the basin are at risk from arsenic and lead exposures, what human health effects might be expected? What are the consequences of arsenic and lead exposure, and how strong is the evidence of toxicity? In addition to the actual risks due to exposure to chemicals, what are the psychosocial consequences of living in proximity to or in the midst of large amounts of potentially toxic materials? Moreover, how might the conclusions of the basin HHRA have been strengthened? In this section, we briefly explore these issues.
Risks from Arsenic
Ingestion of inorganic arsenic is an established cause of skin, bladder, and lung cancer (NRC 1999). Many noncancer health outcomes are also associated with arsenic exposure, including effects on the skin, cardiovascular, nervous, endocrine, hematologic, and renal systems. The primary toxicity from arsenic is oxidative toxicity to cells. A shortcoming of the HHRA is that no human exposure data were collected. Urine and/or hair arsenic
levels are commonly used to quantify chronic arsenic exposure and could have been collected. The risks from arsenic in the basin were mainly determined by modeling human exposures based on arsenic concentrations in environmental samples. Although risk determinations using such modeling are appropriate in the absence of human data, a coupling with actual biological measurements would have strengthened the HHRA. Like lead, there are concerns that some forms of arsenic may not be bioavailable (Caussy 2003; Rodriguez et al. 2003; Turpeinen et al. 2003). The relatively small population size of the basin would make epidemiologic investigation of cancer risk impossible; cancer end points such as skin and bladder cancer are too infrequent to determine increased prevalence in such a small sample.
Risks from Lead
Toxic exposures to lead during early childhood and even fetal life can lead to permanent neurologic deficits. Communities near lead industries frequently have increased exposure. A full review of the epidemiologic evidence for the developmental toxicity of lead is beyond the scope of this report, but the developmental toxicity of lead is clear. Numerous studies have reported inverse associations between infants’ scores on tests of neurobehavioral development and indices of fetal lead exposure such as umbilical cord blood lead concentration (Bellinger et al. 1987; Wasserman et al. 1994) or maternal blood lead during pregnancy (Dietrich et al. 1987). In some studies, associations between prenatal lead exposure and children’s neurobehavioral outcomes ultimately decrease with time, although associations tend to emerge between postnatal exposures and later childhood (Bellinger et al. 1992). Canfield et al. (2003) recently reported that the inverse association between BLL and IQ at age 7 is apparent among children whose BLLs never exceeded 10 μg/day. This finding is consistent with Schwartz’s (Schwartz 1994) nonparametric smoothing analyses of the 10-year follow-up data of the Boston study and with a report on cognitive effects associated with BLLs <10 μg/dL (Lanphear et al. 2000). Recent studies also suggest associations with important forms of psychosocial morbidity (Bellinger et al. 1994; Needleman et al. 1996; Wasserman et al. 1998), including juvenile delinquency (Needleman et al. 2002).
For decades, the impact of environmental lead exposure on children has been a central focus of the field of environmental health. However, there is a growing body of more recent evidence that environmental lead exposure is also associated with an important set of adverse health effects in adults. For example, bone lead levels that were related to lead in drinking water in Boston (Potula et al. 1999) were associated with the development of hypertension among participants in the Normative Aging Study (Cheng et al. 2001). In the same cohort, elevated blood and bone lead levels in-
versely predicted performance on the Mini-Mental Status Exam (Wright et al. 2003). Environmental lead exposure has also been linked to elevated blood pressure and proteinuria among pregnant women (Factor-Litvak 1992). Lead exposure in women of childbearing age is a hugely important issue because lead is known to freely pass the placenta to the unborn child (Graziano et al. 1990). Furthermore, there is evidence that calcium supplement, a simple and cost-effective intervention, will reduce the resorption of lead from bone to blood during pregnancy and limit fetal lead exposure (Janakiraman et al. 2003). Recent studies have also identified environmental lead exposure as a risk factor for essential tremor, one of the most common neurological diseases (Louis et al. 2003; Louis in press). Thus, while the focus of remedial activities has nearly always been due to potential risks to children, the adult population is also vulnerable to significant lead-related morbidity.
Risks from Psychosocial Stress
At the town hall meetings that occurred during the committee’s two visits to the region, some residents, but certainly not all, expressed fears and concerns about possible exposures to hazardous substances. Nothing in the Superfund law (CERCLA) requires EPA to consider community stress from designation of a region as a Superfund site. Nevertheless, there is substantial evidence concerning the psychosocial consequences of living in proximity to hazardous materials at Superfund and other sites, including Love Canal, New York, Three Mile Island, Pennsylvania, and the Exxon-Valdez disaster in Alaska. Furthermore, an Agency for Toxic Substance and Disease Registry (ATSDR) expert panel report (Tucker 2002) recommended both additional research on the effects of psychosocial stress in communities impacted by toxic waste and the development of public health intervention strategies to mitigate such stress. These goals clearly have not been achieved, as the literature on the health effects of stress in Superfund communities is sparse, and no such interventions have been developed.
Exposure to toxic chemicals generally is perceived to involve “invisible” contaminants not detectable by the senses. For this reason, the presence of a toxic waste site may induce chronic stress independent of actual chemical exposure. Living near a toxic waste site is associated with health effects that can be slow in onset and insidious in nature. Often, little technical information is available to families about the likelihood of exposure and effects, leaving them uncertain about their actual risk. Helplessness and fear of the unknown are also common complaints in such communities (Kroll-Smith and Couch 1990). People who believe they have been exposed to toxic chemicals tend to develop chronic stress (Fleming et al. 1982), with symptoms including depression, a feeling of lack of control of the environment, in-
creased family quarrels, increased health worries, and increased intrusive and avoidant thoughts (Stone and Levine 1985; Davidson et al. 1986; Gibbs 1986; Levine and Stone 1986; Edelstein 1988; Stefanko and Horowitz 1989). Trust in both government agencies and scientific experts erodes when communities perceive a failure to adequately respond to toxic contamination (Kroll-Smith and Couch 1990). Children of parents who report chronic stress from the uncertainty of toxic exposures also tend to report increased stress (Edelstein 1988). As a moderating factor, social support can help families cope with stressful events (Figley 1986; Unger et al. 1992). The existence of increased social supports predicted a reduction in symptomatology among subjects living proximal to Three Mile Island (Bromet and Dunn 1981). Unfortunately, social supports can also be eroded by residence near a toxic waste site. Members of a social network may blame the family for moving to the area. Residents may become stigmatized, even ridiculed, further isolating them and increasing their chronic stress (Edelstein 1988).
Such chronic stress from potentially hazardous sites can have multiple adverse health effects. Increased risks of heart disease, hypertension, infection, asthma, premature delivery, and diabetes have been associated with chronic elevated stress. A particular effect of stress that may be relevant to populations with elevated lead exposure is the role of chronic stress in neurodevelopment. Psychological stress results in activation of the hypothalamic-pituitary-adrenal axis. The traditional view is that the hypothalamus produces corticotropin-releasing hormone, which leads to downstream activation of the adrenal cortex to secrete corticosteroids (for example, cortisol) into the blood, which then enter the brain (Sapolsky 2000; McEwen 2001). The hippocampus is the brain region with the highest density of glucocorticoid receptors (Sousa and Almeida 2002). These receptors modulate neurologic development. The primary functional end point of chronic stress appears to be changes in the development and formation of memory. Whereas acute stress may enhance memory formation, chronic stress appears to inhibit it. Animal behavioral studies have confirmed the adverse independent effects of both prenatal and postnatal chronic stress on memory and learning (Zaharia et al. 1996; Vallee et al. 1999; Aleksandrov et al. 2001; Frisone et al. 2002). Research on children exposed to political or domestic violence suggests that a number of the domains of cognitive, social, and emotional function are adversely affected by exposure to such stressors (Golier and Yehuda 1998). With respect to “lower doses” of chronic stress, maternal anxiety both during pregnancy and postnatally, have been independently associated with a 1.5- to 2-fold increase in risk for behavioral/emotional problems in children at 4 years of age (O’Connor et al. 2002a,b).
The social stress associated with potentially hazardous sites may have adverse health effects independent of chemical exposure. As previously outlined, the development of the brain is likely affected by hormonal signals
which modify neuronal-genesis and synaptic formation and synaptic pruning (LeDoux 2002). Environmental factors can promote or disrupt this process depending on whether they are positive (social supports, good nutrition) or negative (toxicants, malnutrition, trauma) (Nelson and Carver 1998). Animal research suggests that the social environment will modify the toxicity of lead and the combined effects of lead and social isolation may augment toxicity (Schneider et al. 2001; Guilarte et al. 2003; Cory-Slechta et al. 2004). In humans, poverty, psychological stress, and lead exposure are likely correlated, but the nature of the relationship (independence [additive toxicity], covariance [confounding], or synergy [effect modification]) in predicting health outcomes has not been determined. Clearly, this is an area of great research need, especially at Superfund sites.
Risks Unique to the Coeur d’Alene Tribe
Most hazardous waste sites on American Indian lands have never been evaluated for their impact on the cultural resources and practices of the tribes who inhabit them (Osedowski 2001; Harper et al. 2002). Furthermore, many American Indian lands border contaminated lands not designated as Superfund sites. These sites represent potentially important sources of plants and wildlife used in traditional diets and may be contaminated with toxic materials. With information on the real risks of contamination in their traditional lifestyles, tribes will be empowered to make decisions based on this information and can educate tribal members about uses of exposed resources and continue their traditional lifestyle without compromising their cultural identity or health (Harris and Harper 1997).
American Indian tribal members may choose to follow traditional lifestyles despite knowing that there are risks posed by environmental contamination. Maintaining a homeland where present and future generations may live in a clean, functioning ecosystem is a goal that often has not been respected by agencies and researchers who study the impact of environmental contamination on native lands. There is also substantial evidence that traditional (noncontaminated) subsistence diets among American Indians are inherently healthier than Western diets and reduce the risk of diabetes and heart disease (McDermott 1998; Lev-Ran 2001). Switching from a traditional lifestyle to a suburban American lifestyle carries significant health risks, emphasizing the importance of providing a clean environment to support traditional lifestyles. American Indian reservations are intended to provide permanent homelands for their members. When these lands are contaminated with industrial waste, environmental justice mandates that exposure assessments appreciate the value of traditional lifestyles.
Exposure scenarios designed for American “suburban lifestyles” have been reported to be unsuitable for tribal communities (Harris and Harper
1997). Harris and Harper described an approach to determining exposure assessment in subjects with a subsistence diet that included qualitative interviews and expert elicitation to determine foods consumed and practices common among tribal members (Harris and Harper 1997, 2001; Harper et al. 2002). Subsistence in this context refers not only to diet but also to cultural and religious practices, which may include medicinal and ceremonial uses of natural resources. The goal is not to increase precision regarding a single pathway of exposure (such as diet) but to increase overall understanding and community awareness about multiple pathways of exposure and the role of culture-based behaviors. All these factors may predispose American Indians to exposure and may make them a vulnerable subpopulation within a Superfund site.
New methodologies are being developed to assess exposure in tribal lands. For example, through the assistance of the tribal governments, expert elicitation of local traditional lifestyle practitioners and tribal elders can assist with environmental sampling strategy. Expert elicitation is a technique used in decision analysis to derive numeric data through interviews with acknowledged experts (Meyer and Booker 1991; Hora 1992). This technique has been used successfully in other studies of American Indian exposure scenarios (Harris and Harper 1997, 2001; Harper et al. 2002). Tribal experts can compare survey results with their knowledge of hunting and gathering practices of their tribal members. Sample locations of plants and animals identified as culturally important could be based on this process.
The Coeur d’Alene River basin HHRA acknowledged that American Indians likely have higher risks than non-American Indians living in the basin. As presented in the HHRA, “it is clear that a subsistence-based lifestyle requires environmental lead levels orders of magnitude lower than those measured throughout the floodplain of the Coeur d’Alene River” (TerraGraphics et al. 2001, p. 6-2). Further, the HHRA concludes, “Estimated lead intake rates for these scenarios are too high to predict BLLs with confidence. Predictions for BLLs associated with subsistence activities … would significantly exceed all health criteria for children or adults” (TerraGraphics et al. 2001, p. 6-51). Given the magnitude and extent of contamination, it is difficult to envision how the tribes could reduce exposure risks to an acceptable level if a return to subsistence lifestyle were to occur.
BLOOD LEAD STUDIES IN THE COEUR D’ALENE RIVER BASIN
The Coeur d’Alene River basin HHRA included some survey data of blood lead concentrations in children, but these were sufficiently limited that the document essentially relies on the IEUBK model to predict risks from lead exposure. The limitations of the blood lead data have their
origins in an agreement between community leaders, the state of Idaho, and EPA, which affirmed that no studies would be conducted for “scientific research or academic” reasons (von Lindern 2004). Basically, blood lead screening programs do not work well when the community is not cooperative. How could the HHRA have been strengthened in this regard?
Ideal Blood Lead Screening Methodology
An ideal screening program would include all at-risk children in a highly lead-exposed geographic area. This program would not be limited to a single cross-sectional measurement but would include longitudinal measurements and an intervention program that is triggered at predetermined BLLs. Widespread participation would ensure not only that most children with high lead exposure are identified and treated but also would allow for epidemiologic assessment of exposure risks for specific sites within the geographic region. Ideal lead screening programs identify specific housing associated with lead exposure—information then used by the state or federal government to direct remediation efforts.
However, the American Academy of Pediatrics no long endorses universal screening for lead poisoning but instead recommends targeted screening in high-risk populations. Today, only 53% of pediatricians in the United States screen blood lead in all their patients before the age of 3 (AAP 1995), but this percentage is much higher in regions where lead hazards are thought to exist. The distinction between a high-risk population and a high-risk individual merits discussion. Questionnaires and risk factors for lead exposure have poor sensitivity and specificity in detecting individual children with elevated BLLs, in part because lead-exposure pathways include home dust, soil, water, and other more unique sources (for example, ceramic pottery). For that reason, the unit of measure for a lead screening program is a high-risk population and not a high-risk individual. The history of the Coeur d’Alene River basin certainly warrants evaluation of its residents as a high-risk population.
An ideal lead intervention program in the Coeur d’Alene River basin would include both primary and secondary prevention strategies for exposure reduction. Observational research has noted associations of lead poisoning with poor nutrition (iron and calcium intake in particular), elevated lead levels in home dust, and elevated lead levels in soil, making nutritional and environmental interventions logical starting points for tempering exposure to lead. As part of primary prevention, nutritional and behavioral risk reduction counseling would be offered to all families with children less than 5 years of age. Secondary prevention would consist of specific exposure-reduction interventions tailored to a specific child with elevated BLLs (>10 μg/dL). This may include home visits to develop and convey strategies
for exposure reduction specific for that child’s home environment. Home inspections for lead paint and soil lead assessments would seek to determine the source(s) of the lead exposure, assisting families in directing their exposure reduction efforts at the source for lead exposure and establishing that the exposure source is indeed the home and not a daycare center, relative’s home, or other site where the child spends a significant amount of time.
However, it should be noted that interventions short of actual remediation of lead sources have not been found to reduce the prevalence of childhood lead poisoning in previous studies. Therefore, these counseling efforts should be adjuncts to remediation efforts in which the lead hazard is removed from the child’s environment. Secondary prevention, which relies on identifying lead-poisoned children is important but should not be the primary focus of public health intervention. Given the lack of effective treatments for lead toxicity, primary prevention strategies are more likely to have a positive public health impact.
Screening Methods Used in the Coeur d’Alene River Basin
Participation is the key to any health screening program. On a national level, state health departments have used several strategies to maximize participation in childhood lead screening programs in the United States. Some states have instituted mandatory annual screening programs for children between the ages of 1 and 4 years. The Women Infants and Children supplemental nutritional program in many states requires that a hemoglobin and BLL be measured before families can participate. Before leaded gasoline was phased out, when high exposures to lead were more widespread, universal screening of all children aged 1-4 years was recommended. However, lead exposure in the general population has been greatly reduced, and more cost-efficient strategies are now appropriate.
Sampling the Coeur d’Alene River Basin Population for Lead Exposure
Data on the prevalence of elevated and mean blood lead concentrations in the Coeur d’Alene River basin between 1996 and 2004 consist primarily of screening conducted at a fixed site for a brief time in the summer months. Screening is not mandatory in Idaho, and there is no evidence that physicians widely screen children in the Coeur d’Alene River basin.6 Therefore, these are the only data available with which to assess the prevalence of lead poisoning and to test the assumptions of the IEUBK model (see Chapter 6). With respect to the validity of the annual blood lead screening data as an accurate characterization of the population distribution of blood lead, only the 1996 data are from an attempt at population-based sampling. The results
of this assessment have been criticized as biased because the overall participation rate was only 25%. Because this study was the only recent attempt at generating representative population-based blood lead screening data, we focus our discussion on the methods used in this study.
A Coeur d’Alene River basin Environmental Health Assessment was conducted before the HHRA by the IDHW with ATSDR funding (ATSDR 2000). State health statistics did not provide a precise count of children living in the Coeur d’Alene River basin; therefore, a comprehensive census was undertaken to determine the denominator for the lead exposure survey. Informational public meetings were held before the 1996 assessment to publicize the meetings, encourage participation, and distribute information on the study. The Idaho Panhandle Health District and TerraGraphics Environmental Engineering collaborated on the project. A census of the basin was conducted in July and August of 1996 to identify all households within 1.5 miles (2.4 km) of the 100-year floodplain of the South Fork and main stem of the Coeur d’Alene River stretching from the border with Montana to Lake Coeur d’Alene. There were 1,643 homes identified.7 Of these, 130 refused to participate in the census. Of the remaining 1,513 homes, 670 provided census data only. All homes were approached in a door-to-door survey. There were 3,651 persons identified as living in the study area. If a home was inaccessible or unoccupied during the visit, a callback form was left at the home. A minimum of three attempts were made to contact each household; 815 households provided soil samples, 222 provided well-water samples, 156 provided vacuum dust samples, 400 provided floor mat dust, 710 provided interior paint samples, and 749 provided exterior paint samples for lead analysis. Paint lead was assessed by a portable x-ray fluorescence machine. The environmental samples were appropriately sieved to collect small particle sizes representative of those that would be found on a young child’s hands after contact.
With respect to blood lead screening, 231 children aged 0-5 years8 and 170 children aged 6-9 years were identified by the census. Of these, 47
(20.3%) children 0-5 years of age and an additional 51 of 170 (30%) children between 6 and 9 years of age participated.
Limitations of the Sampling
In general, a 70% participation rate will provide assurance that significant selection bias did not influence the results. However, epidemiologic studies, or for that matter political polls with targeted sampling strata, can be successful without meeting the goal of 70% overall participation if the selection of participants is not biased. Lead exposure does not occur stochastically, and there are known risk factors for exposure. If selection bias did occur, one would expect differences in the prevalence of such risk factors between those families who participated in the blood lead screening and those who did not. The health assessment (ATSDR 2000) summarized community member characteristics, stratified by blood lead screening participation. Most characteristics were similar between groups. Nonparticipants were more likely to be renters (16.4% versus 9.8%) and were less likely to have attended a four-year college (13.7% versus 18.4%). Both factors likely would be associated with higher BLLs among nonparticipants.
In the years following 1996, blood lead results were from fixed-site annual screenings. Participating families had to bring children to a fixed site for the sole purpose of obtaining a blood lead measurement. Bias is much more likely to have occurred from this screening program. The direction of this bias is impossible to predict as no demographic data were collected with the screening. For these reasons, the 1996 data (which are the best available) and subsequent blood lead data have serious limitations for the purpose of making policy decisions.
Shifting the design from a fixed site to a more widespread screening program utilizing the local health care community likely would increase participation. This type of screening program would provide a population of participants less likely to be biased. Such a practice could be timed to coincide with other medically indicated health care screening tests conducted by primary care physicians. For example, screening for iron deficiency anemia is routinely conducted for children 1-5 years of age. Blood lead screening could be timed to coincide with this blood draw, thereby minimizing inconvenience to the family and child. Linking the screening program to pediatric well-child visits likely will increase participation, will provide built-in follow-up for children with elevated BLLs, and will be more convenient for families.
Blood Lead Studies from the River Basin
The committee found it unusual that this HHRA presented aggregate data on childhood lead screening data for children aged 0-9 years
(TerraGraphics et al. 2001). Children less than 1 year of age are at very low risk for lead poisoning because of their relative lack of mobility. Likewise hand-to-mouth activity falls dramatically at about age 4 years. Children 5-9 years of age are very unlikely to have elevated lead levels. Although the data were further stratified in many cases to 0-5 years and 6-9 years, there was an inexplicable tendency to lump these age groups together.
Figure 5-2 displays geometric mean blood lead measurements for children aged 1-5 years found in annual Coeur d’Alene River basin surveys, together with nationwide results from the National Health and Nutrition Examination Survey (NHANES). Error bars represent 95% confidence intervals on the sample geometric mean (which is taken to be as an estimator of the geometric mean of an underlying population represented by the sample). As noted above, Coeur d’Alene River basin measurements do not reflect random sampling strategies and may or may not be representative of the basin population. However, available sample geometric means are statistically elevated relative to the most closely corresponding NHANES results for all years through 2004.9 (The most recent available NHANES data were collected in 1999-2000. Results of more recent national sampling are expected to be available sometime in 2005 and, on the basis of historical trends, are likely to reflect still lower geometric mean values.) Figure 5-3 compares the same Coeur d’Alene River basin and NHANES blood lead data among 1- to 5-year-olds when expressed as percentages of the respective populations having levels ≥10 μg/dL. Slightly more than 2% of the national population displayed blood lead ≥ 10 μg/dL in 1999-2000. By this metric, the proportion of children in the Coeur d’Alene River basin with BLLs ≥ 10 μg/dL was elevated relative to national norms at least through 2001 (see Box 5-1). The available data indicate that the percentage of children sampled in the basin with BLLs ≥ 10 μg/dL has dropped over time and, in 2004, was approximately 2.8%.
In contrast to national data, the Coeur d’Alene River basin blood data show no discernible downward trend in the years 1996-2000. Between 2000 and 2001, an apparent sharp decline in geometric mean blood lead is observed. This apparent decline may be an artifact of nonrepresentative sampling. If it is real, it appears to be much more rapid than the background rate of decline occurring in the national population. One possibility is that the decline is real and attributable to remedial activities in the Coeur d’Alene River basin. Between 1997 (the inception of remedial activities) and 2000, sixty-six residences, six schools or daycare centers, and five common-use or recreational properties were remediated (TerraGraphics et al. 2001, Table 2.3-1). Remediation of that number of properties could have contributed substantially to

FIGURE 5-2 Geometric mean BLLs among 1- to 5-year-olds in the basin, with corresponding NHANES survey data. The estimation of basin geometric means includes the assumption that values less than the limit of detection equal half the limit of detection. Error bars represent 95% confidence intervals. Basin sample sizes in years 1996 through 2004 were 47, 12, 59, 139, 77, 98, 83, 61, and 71, respectively. It should be noted that the sampling in 1996 (ATSDR 2000) sampled individuals from a smaller area (and population) than the fixed-site sampling in subsequent years. SOURCE: Basin data, IDHW, unpublished materials 2004; NHANES data, CDC 2004.
declining blood lead, since cleanups were intended to first address sites posing the greatest apparent threats, and blood sampling was not random. In any case, this apparent improvement in the Coeur d’Alene River basin results was observed only after substantial remedial activity.
Other Information
Results of follow-up studies of 50 findings of a river basin child exhibiting a high BLL by the Panhandle Health District are reported in the HHRA
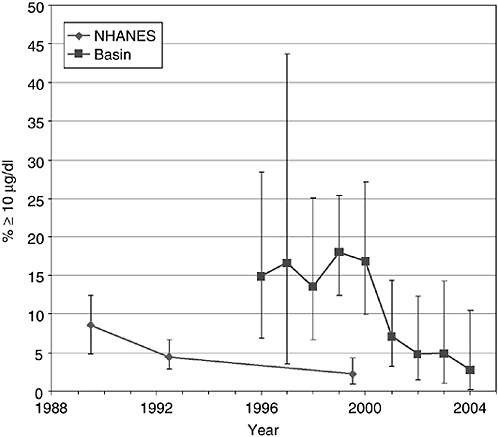
FIGURE 5-3 Comparison of fraction of blood samples among 1- to 5-year-olds from the basin with BLLs ≥ 10 μg/dL with corresponding NHANES survey data. Error bars represent 95% confidence intervals. Basin sample sizes in years 1996 through 2004 were 47, 12, 59, 139, 77, 98, 83, 61, and 71, respectively. It should be noted that the sampling in 1996 (ATSDR 2000) sampled individuals from a smaller area (and population) than the fixed-site sampling in subsequent years. SOURCE: Basin data, IDHW, unpublished materials 2004; NHANES data, CDC 2004.
BOX 5-1 BLLs in Surveys of Children in the Coeur d’Alene River Basin Ideally, to estimate the true prevalence of elevated blood lead in a relatively small at-risk population (like that in the Coeur d’Alene River basin), all children 1 to 4 years of age would be surveyed. To estimate the prevalence at the national level, NHANES has measured a representative sample of children across the country. Some blood lead data are available for children in the Coeur d’Alene River basin, but the extent to which these data are representative of the entire population is not known. Only in 1996 was a door-to-door survey attempted, and even then only 25% of the eligible children were actually tested. Although imperfect, the Coeur d’Alene River basin blood lead data support the hypothesis that Coeur d’Alene River basin BLLs are higher than contemporaneous national BLLs. |
(TerraGraphics et al. 2001). It should be noted that many potential sources of lead exposure to children are not always obvious and are difficult to detect without an extensive history of everything a child has come into contact with (for example, painted furniture, mini-blinds, keys, and key chains). However, elevated lead in residential paint was identified as a risk factor for 5 of 21 children with BLLs ≥ 15 μg/dL and for 3 of 25 children with BLLs of 10-14 μg/dL. (Some children were followed more than once.) In a much higher proportion of cases, high residential soil or dust lead or known access to other properties with high soil or dust lead or to flood-affected areas was evident. Potential risks of flooded properties were illustrated in the box by the Milo Creek flood of May 1997. In that case, a flood deposited sediments with high lead concentration, recontaminating a previously remediated area. A spike increase in elevated BLLs was observed in children in the affected zone (TerraGraphics 2000).
These observations are anecdotal and not convincing in and of themselves. However, in concert with children’s known tendency to ingest soil, the demonstrated (although variable) bioavailability of lead in soil in mammalian gastrointestinal tracts, and observed BLLs in children in the Coeur d’Alene River basin, they do lend support to arguments that Coeur d’Alene River basin soils represent a lead hazard to young children.
Apportioning Risks to Humans from Multiple Contaminant Exposures
The committee was asked to assess the scientific and technical aspects of efforts regarding the following:
Assessing and apportioning risks to humans from multiple contaminant exposures related to waste-site sources as well as other sources (for example, lead exposure via soil and house paint dust). What techniques should be used to identify contaminants of concern and estimate the human health risks attributable to waste-site sources? In this case, were risks attributable to sources other than mining and smelting activities adequately analyzed?
Two issues appear to be involved in this charge. One is whether EPA adequately identified all the exposure sources and assessed the combined risk from multiple exposures. The second is whether EPA adequately apportioned risk among the different exposures when there were multiple sources. Although the specifics of the charge relate to human health concerns, the questions presumably are also relevant with respect to environmental health concerns.
With respect to human health concerns, the agency did attempt to identify possible different sources of exposure. For lead exposures, the agency identified lead paint in older houses as a significant source of exposure, as
well as the lead in yard soils and recreational and other public use areas. Another possible source of lead exposure is air deposition of lead from the exhaust of vehicles using leaded gasoline (which has been phased out) and from the emissions discharged by the Bunker Hill smelter and other ore-processing facilities in the box (eliminated in 1981). It is possible that lead from these sources still exists in the Coeur d’Alene River basin system, although the amounts would be expected to be very small in the areas covered by operable unit 3 (OU-3). The agency did not distinguish these as separate sources.
Although the agency did not identify these as distinct sources of lead exposure, it did include any exposure that still may be associated with these sources in its risk assessment. The exposure from these sources would be found in the same places as exposure from the lead in mining wastes (for example, yard soils and house dust), and the risk assessments were based on actual measurements of the amount of lead found in these exposure sources. Therefore, lead that may still exist from these nondistinguished sources would have been included in the risk assessment.
The agency did not identify any other sources of arsenic exposure, and the committee has not identified any environmental sources of arsenic that EPA may have missed. Again, the risk assessments were based on actual measurements of environmental media and, therefore, would have included arsenic from any unidentified environmental sources.
However, the residents of the area undoubtedly are exposed to other carcinogenic substances. One of these is cadmium, which has been shown to be associated with cancer in metal refinery workers who inhale cadmium fumes, but for which carcinogenicity by the oral route is equivocal. Other sources of possible exposure to carcinogens, such as smoking, pesticides, and other chemicals, are unrelated to the mining wastes. These different exposures to carcinogens may create a carcinogenic risk that is greater than that resulting from exposure to any one source. However, the consensus procedure in current risk-assessment methodology for aggregating such carcinogenic risks from multiple sources is to ignore all sources other than the one(s) of interest, treating multiple sources as exactly additive. Thus, EPA’s failure to explicitly identify and assess these multiple risks reflects the current status of risk assessment procedures.
A similar line of reasoning applies to environmental exposures. Water-quality standards (for instance, for dissolved zinc) are generally established on the basis of how much of that substance alone creates unreasonable risks—although there may be modifying factors (for example, hardness of water). The fact that aquatic species are exposed simultaneously to multiple contaminants probably results in an aggregate risk greater than that posed by any of the single contaminants taken alone (although there are also examples where aggregate risks may be reduced). However, current environmental risk
assessment procedures provide no guidance for aggregating such multiple risks other than by simple addition.
Thus, the answer to the first question implied in the charge is that EPA did consider risks from multiple contaminants to the extent that current risk assessment procedures provide for a basis for making such analyses. Because there is human and environmental exposure to multiple contaminants creating similar risk factors, the aggregate risk may well be greater than that estimated by EPA, but current risk assessment procedures provide no mechanism for estimating such aggregate risks.
With respect to the second question the charge appears to raise, current risk assessment procedures do not include methods for apportioning aggregate risks among multiple sources of exposure. The committee is unaware of any legal requirements that this be done or any practical use of such apportionments (except perhaps to apportion responsibility among potentially responsible parties or to obtain funds to address that portion of the total risk that cannot be remedied under Superfund).
Undertaking such an apportionment would require making a number of significantly simplifying assumptions about factors such as the shape of the dose-response curve, the amount of exposure the “typical” person has to different sources, the biological availability of contaminant in the different sources, and so forth. Given the discussion above, the only contaminant in the Coeur d’Alene River basin for which such apportionment could reasonably be attempted is human exposure to lead.
EPA did undertake a series of statistical analyses attempting to determine the relative effect of lead in mining wastes and lead in paint on BLLs (TerraGraphics et al. 2001, pp. 6-22 to 6-39). Such analyses can be considered only rough indicators because of sample weaknesses and because of the need to use surrogate measures for exposure to leaded paint.10
Nevertheless, these analyses, though not definitive, do strongly suggest that lead in soils was a major contributor to high BLLs. They indicated that
“although lead paint is important [as a source of exposure] for some individuals” “70% (14/20) of the children with high BLLs were not associated with an interior lead paint hazard” (TerraGraphics et al. 2001, pp. 6-29 and 6-25).11 The analyses also include a regression model that generally supports the conclusion that lead in yard soils has a significant impact on BLLs.
Although not strictly an apportionment of risk among exposure sources, these analyses do provide support for the conclusion that lead in yard soils is a significant contributor to elevated BLLs and that reducing exposure to this source is likely to reduce the risk of elevated BLLs. The committee observes that these analyses undertaken for OU-3 go beyond normal attempts to attribute elevated BLLs to different sources of exposure and that no alternative approaches to apportioning risks would have been preferable given the information available.
STRATEGIES TO MANAGE THE RISKS TO HUMAN HEALTH
Control of Exposure by Individuals
In the face of health hazards from contaminated environmental media, a number of measures can and should be taken to reduce exposure. These protective measures include actions that can be taken at the individual level, as well as at the institutional (governmental) level. At the individual level, relatively simple interventions, such as frequent hand washing, removing of shoes before entering the home, and thoroughly washing vegetables can substantially reduce exposures to hazardous substances. Occupations associated with contact with contaminated environmental media should include practices that prevent transporting such materials into the home. The phenomenon known as “fouling one’s nest” is well-known in occupational medicine.
Public notifications, such as those posted by health departments warning residents or recreators not to eat certain fish, to wash their hands, or not to drink certain water can encourage individuals to reduce their exposures to harmful substances. During the committee’s visits to Coeur d’Alene River basin area, many such public warnings were found and thought to be appropriate. Yet the downside of such warnings, expressed by residents during public meetings, is that they appeared to increase psychosocial stress by making the presence of otherwise invisible hazards visible and constant. Public health departments should be aware of this and provide sufficient educational materials to residents to place the hazards in context.
Health Programs
The HHRA states, “The Selected Remedy will include a lead health intervention program [LHIP] similar to the Bunker Hill Box LHIP, which provides personal health and hygiene information and vacuum cleaner loans to help mitigate exposure to contaminants.” However, the selected remedy has few specifications of what it might involve. A comprehensive health program—one that includes health education and resources for exposure prevention—can provide more benefits to the community than just monitoring the remedy. Because soil removal (discussed below) addresses only one source of lead exposure, such a program can help address these other sources. This type of approach has been used effectively at other sites for reducing lead exposure (Kimbrough et al. 1994; Markowitz et al. 1996; Niemuth et al. 2001; Lorenzana et al. 2003). Other sites with such programs include Leadville, Colorado (EPA 1999), Butte, Montana (EPA 2005), East Helena, Montana (LCCCHD 2005), and others. Regular monitoring and intervention also help decrease the duration and magnitude of increases in blood lead. Based on current knowledge, lowering the magnitude and duration of elevated BLLs would be expected to minimize the impact.
Medical Interventions
During its visits to the Coeur d’Alene River basin, the committee heard infrequent pleas from community members who believed that medications should be administered to rid the body of potentially harmful metals. The administration of drugs to remove lead from the body, known as chelation therapy, is reserved for people with significantly elevated body burdens. The first drug ever developed for such use, calcium disodium ethylenedia-minetetraacetate (CaNa2EDTA), must be administered by intravenous infusion. CaNa2EDTA has been associated with improved survival in young children with lead-induced encephalopathy, a syndrome that can occur when blood lead concentrations exceed 70 μg/dL (CDC 1991). This is a level many times higher than now expected in the basin. Because use of the drug is associated with the depletion of essential minerals as well as other adverse effects, it is appropriately reserved for severe cases of lead intoxication.
The CDC currently recommends that chelation therapy be reserved for children whose blood lead concentrations are higher than 45 μg/dL (CDC 1991), who are at risk for further exposure that might lead to encephalopathy. Historically, the blood lead distribution of children in the Coeur d’Alene River basin included cases substantially higher than 45 μg/dL. However, because recent blood lead surveys no longer find children with blood lead in that range, chelation therapy does not appear to be warranted except in rare cases. Chelation therapy should never be used for prophylactic purposes,
because the risks of adverse drug effects far outweigh potential benefits. Chelation in the absence of exposure reduction may be more than ineffective; it may do harm.
An oral medication with a better safety profile, dimercaptosuccinic acid (Succimer), was approved by the U.S. Food and Drug Administration in 1991 (Nightingale 1991; Graziano et al. 1992). In controlled clinical trials, Succimer has proven more effective than CaNa2EDTA in reducing blood lead concentrations and can be used on an outpatient basis (Graziano et al. 1985, 1992). Consequently, the National Institute of Environmental Health Sciences undertook a multicenter randomized, double-blind, placebo-controlled clinical trial to determine whether Succimer might be capable of improving cognitive function in children with blood lead concentrations ranging from 20-44 μg/dL (Rogan et al. 2001). The answer was no, implying that cognitive deficits associated with these levels of lead in blood are not reversible. Though there are no data concerning the impact of chelation therapy on children with lower blood lead concentrations, there is no reason to believe that the use of such drugs, which can be associated with significant adverse effects, would be effective. Thus, medical interventions with drugs that remove lead from the body do not appear to be warranted in the Coeur d’Alene River basin.
Yard Remediations: What Is the Evidence That They Are Effective?
A primary component of EPA’s strategy to mitigate the effect of past lead pollution in residential areas consists of removing contaminated surface soil in residential yards and replacing it with clean soil above a geotextile membrane. The intent of the soil replacement is to reduce the amount of lead that young children take in as they ingest or inhale soil and dust. Children undoubtedly ingest some soil and dust, primarily through mouthing of objects and body parts (particularly fingers and hands), after contact of those objects or body parts with indoor dust or outdoor soil or dust. In addition, they undoubtedly inhale some dust that is raised indoors or outdoors by everyday activities.
The amount of soil and dust ingestion and inhalation in children (or in others) is not known with any great precision, although available measurements and simple calculations suggest that ingestion of dust is more significant than inhalation. Measured soil and dust ingestion clearly varies substantially among individuals and over time (van Wijnen et al. 1990; Stanek and Calabrese 1995), and its magnitude is potentially sufficient to explain elevated BLLs in the presence of lead-contaminated outdoor soil and indoor dust. Eliminating exposures to lead-contaminated dust and soil thus can be expected to result in decreases in blood lead concentrations in children.
However, it does not necessarily follow that remediation of outdoor soil will have a significant or substantial effect on children’s BLLs, and the effect may vary in different circumstances. The relative contribution of indoor dust and outdoor soil to children’s total soil and dust ingestion is currently a matter of conjecture rather than measurement, and their relative contributions to elevated concentrations of blood lead is also not clear. Cross-sectional epidemiological studies indicate that indoor dust is likely to be a more important contributor to elevated blood lead concentrations than outdoor soil (for example, Lanphear et al. 1998), although many such studies are of (or are heavily influenced by) residential soil contamination associated with the same residence (for example, due to lead-based paint) and not primarily due to a large external source that has contaminated or is contaminating whole neighborhoods. The relevance of such studies to a Superfund site such as the Coeur d’Alene River basin is not entirely clear, since the relationship (if any) between outdoor soil and indoor dust may be different and the dynamics of lead transport may also be different.
Typically, multiple sources of lead contribute to residential indoor dust in addition to soil just outside the residence. These include lead-based paint, wind-blown lead-contaminated dust from other locations or sources, tracked-in dust from other locations, and contaminated dust from reservoirs remaining in the household from earlier periods (for example, in attic spaces, crawl spaces, air ducts, under fitted carpets, between floorboards, and generally in nooks and crannies). Different dust sources will give rise to dusts with different characteristics (for example, particle size ranges, lead concentrations, and bioavailability of the lead when ingested or inhaled), so that equal quantities of dust from different sources, or even equal quantities of lead in dust from different sources, presumably are not equivalent in their propensity to elevate BLLs in children. Moreover, children may be exposed to lead by routes other than soil and dust and at locations other than their residence. If other exposures dominate those due to soil and dust in the residence, then reductions in residential soil concentrations may result in relatively small reductions in blood lead concentration.
In view of the uncertainties suggested here, evaluation of the likely overall effect on blood lead concentrations of various remedial actions at residences contaminated by various sources of lead currently can be adequately ascertained only by empirical studies. Realizing this, EPA and others have made efforts to perform and evaluate empirical studies of remedial actions and to evaluate observations made during remedial actions (even when the observations were not made as part of a formal study), although most such remedial actions have been directed at lead-based paint.
A 1995 EPA report (Battelle 1995) examined 16 reports evaluating the effect of remedial actions, with 12 of the reports examining children's blood
lead concentrations as one end point. In ten of the reports, the principal factor evaluated was removal of exposures to lead-based paint; in five, the principal factor was cleanup of interior dust or education to encourage avoidance of dust exposures; and in one, the Boston arm of the Urban Soil Lead Demonstration Project (EPA 1993b; Weitzman et al. 1993; Aschengrau et al. 1994, 1997), the principal factor evaluated was soil removal and replacement in an urban area with no identified principal external lead source.
A 1998 update (Battelle 1998) examined 18 other reports (and in addition included further interpretation of the Boston arm of the Urban Soil Lead Demonstration Project). Five of these additional reports were of soil replacement actions—the Baltimore and Cincinnati arms of the Urban Soil Lead Demonstration Project (EPA 1993c,d) in urban areas with no identified principal external sources, and three Canadian community-wide actions, one in the South Riverdale suburb of Toronto (Langlois et al. 1996) near an operating secondary lead smelter, one in St.-Jean-sur-Richelieu in Quebec (Goulet et al. 1996) near a recently closed battery reclamation plant, and one in the Notre Dame district of Rouyn-Noranda, Quebec (Gagne 1994), around an operating copper smelter.
In a review article focused on remedial actions associated with lead contamination at locations characterized as “hazardous waste sites,” Lorenzana et al. (2003)12 examined the outcomes of eight reports, four on actions that included soil replacement—the three Canadian actions just mentioned and the activities around Port Pirie, Australia (Calder et al. 1994), near a primary lead smelter.
During a presentation to the committee (Southerland 2004), EPA cited four additional locations, and provided some additional supporting information (EPA 2004b). At these locations (Midvale, Jasper County, Bartlesville, and Tar Creek) EPA claimed that available pre- and postremediation measurements of BLLs were supportive of EPA actions at the Coeur d’Alene River basin Superfund site. The results of cross-sectional surveys of children at the Midvale, Utah, site (the former site of a lead, zinc, and copper smelter) have been reported in the peer-reviewed literature (Lanphear et al. 2003). The Jasper County, Missouri, site is near the Eagle-Picher smelter in northwest Joplin, Missouri. An extensive report detailing the surveys of children postremediation is available (MDHSS/ATSDR 2004) and incorporates limited comparisons with an earlier survey preremediation.13 Information available to the committee on the Bartlesville, Oklahoma, site associated with the National Zinc Company smelter is very limited. Results of surveys conducted before remediation are summarized in an ATSDR Public Health
Assessment (ATSDR 1995), whereas only the number of children tested and the number of those with blood lead exceeding 10 μg/dL in each year from 1995 to 2001 are documented in an EPA 5-year-review (EMC2 and Phelps Dodge Corporation 2001). In view of this very limited information, the site is not further considered here. For the Tar Creek, Oklahoma, site, ATSDR (2004a) recently provided a Report to Congress that summarized the available studies.
ATSDR (2004b) has also recently documented the experience at Galena, Cherokee County, Kansas, where remediation included residential soil replacement, and before and after studies on BLLs are available. Louekari et al. (2004) examined BLLs around a former smelter where some soil removal actions were taken; however, the authors did not attempt to evaluate the relative contributions of multiple actions designed to reduce exposures (including closure of the smelter), so this report is not further considered. A further report on Port Pirie has been published (Maynard et al. 2003), providing updated information on BLLs and activities intended to reduce them and including further references (Heyworth et al. 1993) to published material on Port Pirie. A report (Morrison 2003) describing activities around a smelter in the Lake Macquarie area of New South Wales, Australia, was brought to the committee’s attention. However, the activities described did not include soil replacement (although removal of slag was documented as was installation of landscaping covers like bark, chips, and grass), so this report is not considered further here.14
The EPA experience in the Bunker Hill box at the Coeur d’Alene River basin site has also been reported (Sheldrake and Stifelman 2003; von Lindern et al. 2003), where residential areas were contaminated by smelter emissions (the smelter closed in 1981) and mining waste. These studies report on 12 years of blood lead surveys that were conducted between 1988 and 2000. Participation rates over the period 1990 to 1998 averaged 50% for children aged 9 months to 9 years, and more than 4,000 blood samples were collected.
During this time frame, the site had a variety of interventions including community education programs; soil removal and replacement in yards (soil lead concentration >1,000 mg/kg), public areas, and rights-of-way; and stabilization of barren areas contributing to fugitive dusts. Actions focused on the former smelter complex included demolition of the industrial complex and removal of contaminated soils and mining wastes associated with the industrial areas.
On a site-wide basis, the geometric mean yard soil exposure metric decreased from 2,292 mg/kg in 1988 to 182 mg/kg in 1998. The geometric community soil concentration decreased from 1,528 mg/kg in 1988 to 297 mg/kg
in 1998. The geometric mean neighborhood (200 feet) soil concentration decreased from 2,119 mg/kg in 1998 to 325 mg/kg in 1998. During this period, geometric mean BLL decreased from 8.5 μg/dL in 1988 to 4.0 μg/dL in 1998 (and continued to decrease to 2.7 μg/dL in 2001).
The study concludes the following:
Repeat measures analysis assessing year to year changes found that the remediation effort (without intervention15) had approximately a 7.5 μg/dL effect in reducing a 2-year-old child’s mean blood lead level over the course of the last ten years. Those receiving intervention had an additional 2-15 μg/dL decrease. Structural equations models indicate that from 40 to 50% of the blood lead absorbed from soils and dusts is through house dust with approximately 30% directly from community wide soils and 30% from the home yard and immediate neighborhood.
The study also comments on the potential for other interfering effects: “The overall analysis should be viewed as a forensic exercise to learn as much as possible from this decade-long health response effort. Caution should be exercised in considering individual results, as these were not designed experiments” (von Lindern et al. 2003).
The committee agrees with the warning to interpret the results cautiously. Indeed, the lack of any control group necessarily resulted in the methodology assigning the observed decrease in blood lead concentrations to the environmental changes caused by the interventions. Moreover, even if the reductions in BLLs observed in the box were due to the interventions, extrapolation to other locations within the Coeur d’Alene River basin may not be warranted—for example because of differences in behaviors and opportunities for exposure within and outside the box.
Thus, there are 12 reports from a variety of locations that might provide some information on the effects of soil removal and replacement. We provide very short summaries of some salient information from the reports and the conclusions of the original authors in Box 5-2 at the end of this chapter. The committee located no further reports during informal searching of the published literature.
Overall, the magnitude of the effect that various remedial actions have on BLLs is not well defined. In this regard, the conclusion of Lorenzana et al. (2003) is especially appropriate when considering the effect of soil replacement:
The outcomes of the intervention studies suggest that various approaches to intervention of the dust ingestion pathway, alone or in combination,
BOX 5-2 Summary of Twelve Studies Concerning the Efficacy of Yard Remediation We provide here very short summaries of some salient information from the reports and conclusions of the original authors. Baltimore arm of the Urban Soil Lead Demonstration Project (EPA 1993c). Source. No single identified source. Soil lead contamination primarily due to lead paint. Data. Six rounds of blood lead sampling in a population of children aged 6 months to 6 years, with interventions between rounds 3 and 4. Door-to-door recruitment into the study was used. At the first round, 212 children were recruited in the study area and 196 in the control area, a total of 408. By round 3, just 270 children were tested due to attrition and additional enrollment; further attrition occurred in subsequent rounds (no further children were enrolled). Interventions. Exterior lead paint was stabilized and contaminated soil was replaced (lead concentration > 500 mg/kg within property boundaries, with 6 inches of soil replaced and sodded or seeded). Household members were excluded from the property during these operations. Change in surface-soil lead concentration. A reduction of 550 mg/kg (“trimean” measure).a Results. Just before intervention, the arithmetic mean blood level in round 3 testing in the study area was 11.1 μg/dL, and in the control area it was 10.2 μg/dL (the committee estimates the corresponding geometric mean concentrations to be about 9.6 and 9.0 μg/dL respectivelyb). Similar summary statistics postremediation are not provided, although the results of extensive modeling are summarized, and a data compilation is available (EPA 1996a). Study conclusions. “Statistical analysis of the data from the Baltimore lead in Soil Project provides no evidence that the soil abatement has a direct impact on the blood lead level of children in the study.” In view of the presence of lead-based paint in both abated and control areas, it was reported that the conclusion might be more precisely stated as “in the presence of lead-based paint in the children’s homes, abatement of soil lead alone provides no direct impact on the BLLs of children.” Other interfering effects. Lead-based paint was present in both abated and control areas. A smaller decrease in soil lead concentration was achieved than originally was desired in the design of the study (>1,000 mg/kg was hoped for). Cincinnati arm of the Urban Soil Lead Demonstration Project (EPA 1993d). Source. Soil lead contamination primarily due to lead-based paint. Data. Three areas—A, B, and C—were examined, with nine phases of monitoring over a 2-year period, including seven phases with blood lead measurements. A total 307 children were involved, the focus being on 173 children less than 6 years of age who were in the initial recruitment. Interventions. Soil replacement, interior dust abatement (including carpet and some upholstered furniture replacement), and exterior dust abatement were used. Between phases 1 and 2, area A was abated for soil, interior dust, and exterior dust, and area B was abated for interior dust only. Between phases 5 and 6, area B was abated for soil and exterior dust. Area C was not abated during the study (it was abated afterward, but no monitoring was performed afterward). |
Change in surface-soil lead concentration. In area A, geometric mean lead concentration in the top 2 cm core-composite samples decreased from 200 mg/kg preabatement to 54 mg/kg postabatement. In area B, geometric mean lead concentration in the top 2 cm core-composite samples decreased from 161 to 60 mg/ kg. The committee estimates that these correspond approximately to changes in arithmetic mean concentrations from 690 to 120 mg/kg in area A and from 410 to 90 mg/kg in area B.c Results. Immediately after abatement, small but nonsignificant reductions were observed in blood lead concentrations (for example, after abatement in area A, the geometric mean blood lead decreased from 8.9 to 7.0 μg/dL), but these reductions were transient and vanished by the next phase of sampling. Moreover, similar or larger variations were observed in the control area C. Study conclusions. “There was no evidence that blood lead levels were reduced by soil lead or dust abatement in area A. There was a slight reduction (net reduction over control area of 0.6 μg/dL in Area B that may be attributed to interior dust abatement (this difference was not statistically significant).” Other interfering effects. Relatively small reductions in soil concentrations. The study was carried out primarily in multifamily housing units rehabilitated and lead-abated two decades earlier. However, these housing units were intermixed with nonrehabilitated units. Soil was not primarily associated with individual buildings. Boston arm of the Urban Soil Lead Demonstration Project (EPA 1993b; Weitzman et al. 1993; Aschengrau et al. 1994, 1997). Source. Soil lead contamination probably primarily due to lead-based paint. Data. BLLs in 152 children initially aged up to 4 years and with BLLs from 10 to 24 μg/dL (or living in housing units containing a previously selected child with a BLL in that range), selected also according to geographical area and certain housing conditions, randomly assigned to a study group (group S, 54 children) or to comparison groups A (51 children) and B (47 children). In phase I (EPA 1993b, Weitzman et al. 1993), BLL was measured preabatement, and approximately 6 and 11 months later, the latter an average of about 9 months after abatement. In phase II (Aschengrau et al. 1994, 1997), BLLs were measured at approximately 22 months, an average of about 9 months after the second round of abatements. Interventions. In phase I, group S homes had soil replacement, interior dust abatement, and loose interior lead-based paint stabilization; group A homes had interior dust abatement and loose interior lead-based paint stabilization; and group B homes had loose interior paint stabilization. In phase II, comparison groups A and B had soil replacement, and residential lead-based paint removal was offered to all three groups. Change in surface-soil lead concentration. Average soil concentration in group S was reduced from approximately 2,255 to 160 mg/kg.d Results.e In phase I, the mean BLLs of group S decreased from 13.10 to 10.65 μg/dL at 11 months, those of group A from 12.37 to 11.49 μg/dL, and those of group B decreased from 12.02 to 11.35 μg/dL (the 11-month point was considered most appropriate to minimize seasonal effects). The reduction in group S was significantly larger than in groups A and B but lower than that incorporated in the study hypothesis. Adjusting the results in various ways did not change these conclusions significantly. In phase II, the mean decline in BLLs in groups A and B was |
larger than seen in group S in phase I, and fairly complex analyses were applied to estimate the effect of soil replacement. Study conclusions. “The combined results from both phases suggest that a soil lead reduction of 2060 mg/kg is independently associated with a 2.25 to 2.7 μg/dL decline in blood levels” implicitly, after approximately 2 years. Other interfering effects. In phase I, paint stabilization and dust cleanup ef fects cannot be entirely separated from soil replacement. In phase II, seasonal effects, the secular effects of aging, and selection biases cannot be ruled out, and there was no control group. Final results depend to some extent on the modeling assumptions made. Toronto Soil and Dust (Langlois et al. 1996). Source. A secondary lead smelter operated throughout the period of study. Data. BLLs collected in six cross-sectional surveys of children less than 6 years old in a study area in South Riverdale (SR), two cross-sectional surveys of a sociodemographically similar comparison area in South Riverdale (SRC), also of children less than 6 years old, and four surveys in the school-based Ontario Blood Lead Study (OBLS) (children aged 3-6) distant from the source. Surveys were carried out in 1984, 1987, 1988, 1989, 1990, and 1992 (SR); in 1988 and 1990 (SRC), and in 1984, 1988, 1990, and 1992 (OBLS). Response rates varied from 75% to 32% and decreased over time. Interventions. Most of the 970 properties in SR with soil concentrations of lead exceeding 500 mg/kg had the top 30 cm of soil replaced in 1988. In 1989, professional housecleaning was offered to all 1,029 households in the soil testing area in SR, and 717 households agreed. Change in surface-soil lead concentration. Not stated (soil lead concentrations were measured and were used in an analysis of variance). Results. BLLs declined in all three areas surveyed. Mean values varied from 14 to 3.9 μg/dL in SR over the period of 1984-1992 and from 11.9 to 3.5 μg/dL in OBLS over the same period. Study conclusions. The decrease in blood lead during the 1980s was consistent with observations from other areas, with the most-likely major responsible factors being the reduction in lead in gasoline and in canned food. Three study observations of community-level averages suggested the possibility of an effect of interventions—a reduction of BLLs in SR below extrapolated values, significant changes in time trends after 1988, and a more rapid decline after 1988 in BLLs in SR compared with SRC. However, individual data gave a different impression, because blood lead concentrations in individual children who did not experience any abatement action in their household decreased faster than blood lead concentrations for children experiencing abatement. Overall findings “were equivocal and did not strongly support or refute a beneficial abatement effect.” Other interfering effects. The concentration of air lead levels in Toronto declined over the study period and more rapidly during 1987-1988; decreased emissions from the smelter also may have played a part. Rouyn-Noranda Soil (Gagne 1994). Source. A 2,500-ton-per-day copper smelter operating since 1927. |
Data. BLLs from three surveys in the Notre Dame district within 1 km of the smelter (in 1978, 1989, and 1991) of 2- to 5-year-olds (except in 1991, 1-year-olds were included). Interventions. No interventions were considered necessary in 1978, because the BLL (21 μg/dL, geometric mean; 95th percentile, 29 μg/dL) was below the CDC guideline of 30 μg/dL at that time. In 1990-1991, all residential lots with soil lead concentration exceeding 500 mg/kg, including 80% of the 710 lots in the Notre Dame district, had soil replaced to a depth of 10 cm and then grassed or covered with gravel. Change in surface-soil lead concentration. Not stated. Mean soil lead concentration in 1989 was 700 mg/kg. Results. In 1978, geometric mean BLL was 21 μg/dL in a sample of 29 children. In 1989, geometric mean blood level was 10 μg/dL (in 117 of 124 eligible children, 94%), and this decreased to 7.3 μg/dL for 2- to 5-year-olds in 1991 (87 children 2 to 5 years old, 95% participation in 1- to 5-year-olds overall). Study conclusions. These results were considered indirect evidence of the efficiency of soil decontamination in reducing BLLs. Other interfering effects. Smelter emissions were declining over the period, from 850 tons/year in 1988 just before the study to 300 tons/year in 1991. In 1991, 24 of 29 children with a BLL exceeding 10 μg/dL lived in the portion of the district nearest to the smelter, with significantly more dustfall than the remainder of the district. It was hypothesized that exposure to air lead and/or actual lead dustfall on hard surfaces would explain the difference in blood lead between children living in and out of this portion of the district. Age distributions were not reported or corrected, and differences could have biased results. St. Jean-Sur-Richelieu Soil and Dust (Goulet et al. 1996). Source. A battery-reclamation plant, presumably emitting lead dust (the distribution of contamination corresponded to the prevailing winds). Data. In September 1989, the BLLs of children 0-10 years of age within 600 m of the plant were measured (81.6% participation rate). A second survey in August 1991 measured the BLLs of 101 children aged 6 months to 10 years (79.2% participation rate) living within 150 m of the plant. Interventions. Asphalting of the plant yard, contaminated soil replacement, professional home cleaning, street dust cleaning, public health education campaign. Change in surface-soil lead concentration. Not stated. The median lead concentration of soil samples within 200 m of the site was 500 mg/kg, ranging up to 5,040 mg/kg, before replacement of soils with lead concentrations less than 500 mg/kg. Results. For children 6 months to 5 years old, a reduction in geometric mean blood lead from 9.8 to 5.5 μg/dL; for those 6 months to 10 years old, a reduction from 9.2 to 5.0 μg/dL. Results were similar for those children measured in both surveys. Study conclusions. The lead-poisoning prevention program reached its main objective to lower mean BLL of children to the 5-8 μg/dL range. Other interfering effects. Other remedial actions were taken. The plant was shut down one month before the first blood lead survey. Two measured oral |
activities of children (pica and putting things in their mouths) were significantly decreased in the 1991 children compared with the 1989 children. Various demographic factors, including age distributions and differential response rates, could have biased results. Port Pirie, South Australia, Study (Heyworth et al. 1993; Calder et al. 1994; Maynard et al. 2003). Source. Continuing operation of the Pasminco Port Pirie, one of the largest primary lead-zinc smelters in the world. Data. During the first 10-year lead program, beginning in 1984, school-based screening for blood lead was offered to children up to 7 years old every 6 months. Between approximately 500 and 1,000 children participated in each 6-month cycle. Since 1995 (during a second lead program) screening has been census-based and annual for children up to 5 years, with approximately 95% participation. Interventions. In the first lead program, interior and exterior lead-based paint abatement, interior and exterior cleaning and sealing against dust ingress, soil replacement, greening, active discouragement of use of rainwater for drinking and cooking and provision of clean water, community education, and smelter environmental controls. In the second program, buying and removal from use of properties nearest to the smelter, continuing education, dust control, behavior modification, targeted residential modifications, and a continuing investigative program at the smelter to identify and control emissions. Change in surface-soil lead concentration. Not stated.f Soil with lead greater than 5,000 mg/kg of lead was replaced, and assistance was provided to homeowners to cover soil measuring 1,250 to 5,000 mg/kg, with only educational advice provided for lower concentrations. Results. Geometric mean BLLs for children up to 7 years old declined from 17.8 μg/dL in 1984-1985 to 12.5-13.0 μg/dL in 1991, and for children up to 5 years old from 13.6 μg/dL in 1993 to 10.6 μg/dL in 1999. There is considerable variation in BLLs, with children nearer the smelter having highest blood leads; the variation in 1999 was from a geometric mean of approximately 19.8 μg/dL in the highest residential location to 8.2 μg/dL in the lowest tested area. The largest reductions have occurred in the least-affected areas. Study conclusions. House decontamination (removal of dust, abatement of paint, repairs to reduce dust entry, soil replacement) “produced a transient reduction in blood lead, levels subsequently increased again after 6-12 months” (Heyworth et al. 1993, as cited in Maynard et al. 2003). Other interfering effects. The many other efforts to reduce exposure cited above, together with apparently continuing efforts to reduce smelter emissions. Analysis is complicated by the voluntary nature of the testing, the lack of preintervention data, and the lack of a control group (Heyworth et al. 1993). Midvale, Utah (Lanphear et al. 2003). Source. A former smelter (closed 1958) and milling operation (closed 1971) and the associated tailings piles with high concentrations of lead and arsenic. Contamination was by wind, through transport on workers’ clothing, and through use of tailings on residential properties. |
Data. Two cross-sectional surveys of children aged 6-72 months in Midvale; in 1989 a random sample (112 children, 90% participation), and in 1998 a full population sample (215 children, 70% participation). Interventions. The tailings were covered with a clay cap in 1993. From 1993 to 1996 soil replacement was performed in yards with soil concentrations exceeding 500 mg/kg lead. Change in surface-soil lead concentration. The decline in average “foundation soil lead” was 488 mg/kg in the intervention group (542-54 mg/kg, a significant reduction), whereas in the control group it was 49 mg/kg (from 144 to 95 mg/kg, not significant). Results. In 1989, the geometric mean blood lead of the 73 children in homes with average soil concentrations greater than 500 mg/kg that were subsequently abated was 5.6 μg/dL, and in the 39 children in homes with mean soil concentration less than 500 mg/kg that were not subsequently abated, it was 3.9 μg/dL. In 1998, the geometric mean blood lead of the 167 children in homes that were abated was 3.0 μg/dL, and that of the 31 children in homes that were not abated was 2.6 μg/dL. Socioeconomic status differed between abated and nonabated homes both in 1989 and 1998, but mouthing behaviors did not. Adjustment for age, mouthing behavior, economic status, and year of study suggested a 2.3 μg/ dL decline in blood lead concentration associated with soil abatement, but this decline was not statistically significant. A similar analysis for children aged 6-36 months gave a statistically significant decrease of 2.5 μg/dL, equivalent to 3.5 μg/ dL per 1,000 mg/kg reduction in soil lead. Study conclusions. “Soil abatement was associated with a significantly greater reduction in blood lead concentration than expected among children ages 6 to 36 months who had not been exposed to lead-contaminated yards in early childhood. In contrast, soil abatement was not associated with a greater reduction in blood lead concentrations than expected for children ages 36 to 72 months.” Other interfering effects. The study assigns the entire effect to soil abatement, but there is no discussion of any assessment of whether the capping of the tailings pile had an independent effect (for example, through reduction of the effect of windblown dustg). The possible effect of interior and exterior lead-based paint was also not apparently modeled—tabular data presented show significant differences between remediated and unremediated groups in an index of both interior and exterior lead paint, and significant declines in both indices between 1989 and 1998; no mention is made of the meaning of these indices or of their potential importance. Jasper County, Missouri (EPA 2002; MDHSS/ATSDR 2004). Source. A primary lead smelter (the Eagle-Picher smelter in Joplin, Missouri) that operated into the 1970s, together with mining and milling wastes.h Data. Two cross-sectional surveys, in 1991 and 2000, of BLLs in the same geographical areas. Random samples of children (213 in 2000, 225 in 1991) aged 6-72 months were obtained, but with low response rates (36% in 1991, 34% in 2000). Interventions. Yard soil replacement, educational efforts, bottled water in some locations. |
Change in surface-soil lead concentration. Mean soil concentration was 599 mg/kg in 1991 and 519 mg/kg in 2000. These results are not comparable, because of different sampling methods and different sampling frames (all homes in 2000, whereas in 1991 only a random sample together with children with blood lead exceeding 10 μg/dL). Results. Arithmetic mean BLL in 1991 was 6.24 μg/dL, and in 2000, it was 3.82 mg/dL. Geometric mean values are not given, but the committee estimated them from the information provided as 4.9 μg/dL in 1991 and 3.3 μg/dL in 2000.i Study conclusions. “Although it is not possible to determine the individual contribution of the soil remediation compared with the health education and paint stabilization, it is reasonable to conclude that the substantial soil remediation actions contributed significantly to the reduction in numbers of children with elevated BLLs.” Other interfering effects. Several other lead-related environmental indicators were substantially changed between surveys, including measures of indoor and outdoor paint levels, and there appeared to be a substantial rebuilding rate, with approximately one-third of the houses less than 10 years old in both 1991 and 2000. Lead water-pipe use declined from 9.1% to 1.9%. Data on a somewhat augmented sample of children in 2000 (including an area outside that sampled in 1991) indicate that fewer than one-third of the homes in which the surveyed children live had soil remediation. There were no 1991 to 2000 comparative analyses that attempted to take account of any of these environmental changes. Sampling strategies differed somewhat between 1991 and 2000 (in 1991, two children were sampled from 33 homes, whereas in 2000 only one child was sampled from each home); modifying the 1991 sample by randomly selecting only one child per home reduced the arithmetic mean 1991 blood level from 6.24 to 5.85 μg/dL (geometric mean approximately 4.8 μg/dL). The Bunker Hill Box at the Coeur d’Alene River Basin Superfund Site (TerraGraphics 2000; Sheldrake and Stifelman 2003; von Lindern et al. 2003). Source. Smelter emissions (the smelter closed in 1981) and mining waste. Data. More than 4,000 blood samples in children aged 9 months to 9 years over a period of approximately 12 years from 1988, obtained by door-to-door survey with $20 payment for participation. Estimated participation rate ranged from 42% to 58% from 1990 to 1998 (average 50%). Interventions. Community education programs. Soil removal and replacement in yards (soil lead concentration >1,000 mg/kg), public areas, and rights-of-way. Stabilization of barren areas contributing to fugitive dusts. Final demolition of the industrial complex. Removal of contaminated soils and mining wastes associated with the industrial areas. Change in surface-soil lead concentration. Multiple measures of soil concentration have been tracked and changed in different ways in different communities in the site. On a site-wide basis, the geometric mean yard-soil exposure metric decreased from 2,292 mg/kg in 1988 to 182 mg/kg in 1998. The geometric community soil concentration decreased from 1,528 mg/kg in 1988 to 297 mg/kg in 1998. The geometric mean neighborhood (200 feet) soil concentration decreased from 2,119 mg/kg in 1998 to 325 mg/kg in 1998. |
Results. Geometric mean BLL decreased from 8.5 μg/dL in 1988 to 4.0 μg/dL in 1998 (and continued to decrease to 2.7 μg/dL in 2001). Study conclusions. “Repeat measures analysis assessing year to year changes found that the remediation effort (without intervention)j had approximately a 7.5 μg/dL effect in reducing a 2-year-old child’s mean blood lead level over the course of the last ten years. Those receiving intervention had an additional 2-15 μg/dL decrease. Structural equations models indicate that from 40 to 50% of the blood lead absorbed from soils and dusts is through house dust with approximately 30% directly from communitywide soils and 30% from the home yard and immediate neighborhood.” Other interfering effects. “The overall analysis should be viewed as a forensic exercise to learn as much as possible from this decade-long health response effort. Caution should be exercised in considering individual results, as these were not designed experiments” (von Lindern et al. 2003). Indeed, the lack of any control group necessarily resulted in the methodology assigning the observed decrease in BLLs to the environmental changes caused by the interventions. Galena, Cherokee County, Kansas (EPA 1996b, 2000a; ATSDR 2004b). Source. Primarily smelter emissions (one or more smelters operated in the town from 1890 through 1960 [Breggin et al. 1999]), with possibly some import of mining wastes for construction, fill, and landscaping material. Data. In 1991, BLLs for 52 of 63 children aged 6 or younger and environmental sample results (soil, dust, paint) for their 52 homes. Also in 1991, environmental samples from a random sample of homes of children of all ages, and blood lead values for 128 children aged 6 or younger from a control area. In 2000, BLLs of 100 children aged 6 months to 6 years and environmental samples from their 72 homes, 31 of which had been remediated and 41 not. The estimated response rates of eligible children were 26% (for all 63 children) in 1991 and 33% in 2000. Interventions. Excavation of residential soils exceeding 800 mg/kg lead or 75 mg/kg cadmium to a depth of 1 foot or until the soil concentration does not exceed 500 mg/kg lead or 25 mg/kg cadmium; or of garden soil exceeding 500 mg/kg lead or 75 mg/kg cadmium. In addition, health education, institutional controls, and an operation and maintenance program were part of the intended interventions.k Change in surface-soil lead concentration. For remediated homes, soil lead concentrations declined from 1,660 mg/kg to 345 mg/kg (n = 30), while for non-remediated homes, soil lead concentration was not significantly different (448 mg/ kg in 1991 and 491 mg/kg in 2000, n = 30). It was not specified whether these were arithmetic or geometric means, although the committee infers that geometric means were used. Results. In 1991, the 52 children from Galena had a geometric mean BLL of 4.13 μg/dL, higher than the 3.13 μg/dL for the 128 children in the control area. In 2000, the 100 children from Galena had a geometric mean blood level of 2.29 μg/ dL (there was no comparison group in 2000). Study conclusions. “… both blood and soil lead levels have significantly decreased” and “There was no significant difference in mean BLLs in children living |
in either remediated or non-remediated homes in 2000. The reduction in BLLs from 1991 to 2000 in Galena was better than that expected, based on the U.S. population. These results suggest the effectiveness of the remediation and education effort in reducing BLLs in children in Galena.” Other interfering effects. Other interventions are mentioned above. The low response rate could have biased results. The lack of any comparison group in 2000 makes interpretation difficult. There was no attempt to estimate the effect of soil removal independent of other actions. Tar Creek Superfund Site, Ottawa County, Oklahoma (EPA 2000b; ATSDR 2004a). Source. Extensive chat (mining waste) piles in residential areas and use of chat as a construction product and lead-based paint. Data. A combination of blood lead results obtained between 1995 and 2004 from the Oklahoma Child Lead Poisoning Prevention Program, Tribal Efforts Against Lead surveys, and the Ottawa Lead Poisoning Prevention Program. Interventions. Residential and play-area soil removal and replacement, community and healthcare provider education, blood lead screening efforts, distribution of HEPA vacuums to households with children having elevated BLLs. Change in surface-soil lead concentration. Not evaluated. Results. Declines in measures of BLLs, including geometric mean blood levels (from about 4.8/6.7 μg/dL in 1995/1996 to 2.7/3.0 μg/dL in 2002/2003), and the fraction of children with blood lead level over 10 μg/dL. Study conclusions. No evaluation was made of the effectiveness of soil removal/remediation efforts; it was assumed that remedial efforts had been effective in producing the observed decline in BLLs and that “Existing programs should be evaluated to determine how they may have contributed to this decline.” |
contributed to declines in blood lead levels in children living in areas heavily contaminated with lead…. However, the effects of confounding factors and the lack of control … made it difficult to assess the magnitude of the contribution of intervention and the relative contributions of the various interventional approaches.
At best, the evidence available that soil replacement contributes to declines in BLLs is suggestive, as may be seen from the 12 reports discussed in Box 5-2 at the end of this chapter. Theoretically, because of the practical certainty of some ingestion of soil and dust, removal of soil should have some effect on BLLs. However, the magnitude of that effect is clearly small enough to be difficult to measure and may well be substantially smaller than would be predicted
by models such as the IEUBK as usually used to estimate the effects of soil and dust lead from the types of measurements usually made on soil and dust.
The experience with lead from gasoline,16 the observations around operating smelters summarized in Box 5-2, and the observation of large changes in blood lead in response to fluctuations in smelter emissions (Hilts 2003; Morrison 2003)17 suggest that more attention should be paid specifically to
16 |
The effect of air lead, primarily from gasoline, on BLLs was two to three times larger than would be expected from inhalation alone (EPA 1986) but without concomitant changes in measured soil concentrations. |
17 |
The effect of possible reduction of emissions from the Rouyn-Noranda smelter due to the recent (2002-2003) strike might be observable in BLLs of the adjacent population. |
the surface films of dust with which we come in contact rather than the larger samples generally obtained by soil sampling or vacuuming.
Institutional Controls
Institutional control programs18 are critical for the long-term protection of human health. Once yards, recreational sites, and other properties have been remediated, opportunities for disruption of protective remedial barriers still exist. In 1995, the Idaho Panhandle Health District (PHD) was given the authority by the legislature to issue rules governing the management of contaminants. Public outreach and education play an important role offering protection to individuals. However, institutional control programs, such as the one coordinated by the PHD, can ensure that building, construction, renovation, and soil excavation activities do not lead to human exposure to soil contaminants. Those programs include important components that will need to be maintained over time.
Contractor Licensing
The PHD licenses all contractors involved in soil excavations, building renovations, and other comparable activities that might disrupt existing barriers to human exposures. Contractors are provided education and must pass a test that involves questions about methods of contaminant management and the reasons that they are important.
Large Work Permits
The PHD issues work permits for a variety of activities, including planned developments, land-clearing activities, excavations, and property improvements, all of which might expose contaminated materials. PHD work permits are required before municipal work permits can be approved.
Interior Work Permits
The PHD issues interior work permits, which are required for activities that include ceiling or attic work that might lead to exposure to contaminated dust, work in crawl spaces that contain contaminated soils, installation or removal of insulation, air conditioning or furnace duct work, and excavation of contaminated soil from an interior site.
Inspections
The PHD also carries out inspections of work performed under interior or large work projects. Inspection of approvals and reasons for disapprovals are recorded into a database tracking system.
Collectively, this institutional control program, with its “cradle-to-grave” approach, has outstanding characteristics and components that have been designed to work synergistically. The approach gives the PHD the capability to provide incentives and enforcement to commercial and residential activities that potentially might lead to recontamination and human exposure to hazardous materials. Prolonged funding of this program will be a critical component of the long-term success of any remedial efforts.
ADHERENCE OF THE PROPOSED ACTIONS TO SUPERFUND GUIDANCE
Summary of the Guidance
The Coeur d’Alene River basin was designated as a Superfund site and listed on the National Priorities List in 1983; thus, all assessments and decisions made pertaining to the site fall under the authority of Superfund. HHRA is a key part of Superfund site cleanup. Baseline risk to humans under the status quo at the site, as well as under potential remedial actions, is estimated in order to establish remedies that will protect public health in the present and into the future. Risk assessments constitute one source of information that enters the remedial decision-making process, also known as risk management. They identify how much cleanup is desirable, and then a feasibility study is conducted to assess the likely effectiveness and cost of alternative methods for reducing these risks. The agency selects a preferred alternative on the basis of these assessments, and then, after public comment, formalizes its final risk-management decision in the record of decision (ROD). These processes are described extensively in Chapter 8 of this report.
EPA Superfund risk assessments and the level of protectiveness conferred by decisions are characterized by the following (in keeping with federal guidance). Decisions assume “reasonable maximum exposures,” rather than worst-case bounds on exposure. Site-specific information forms the basis for assessments where available; however, it is necessary to rely on default assumptions about values for which data are scarce or nonexistent.
Adherence to the Guidance
Regarding human health protection and compliance with ARARs, the HHRA for the Coeur d’Alene River basin generally satisfies the guidance laid out under Superfund.
-
Baseline human health risks attributable to lead and arsenic were adequately established in the HHRA for the Coeur d’Alene River basin, including both waste site sources and other sources.
-
ARARs and other factors to be considered (TBCs) were considered in establishing this health risk. There are no ARARs relating to BLLs or the use of the IEUBK; however, the following were identified as TBC:
-
BLLs were compared with CDC criteria (specifically, a blood lead level of 10 μg/dL) in making this assessment.
-
The IEUBK model was used to predict BLLs as is required by Superfund Guidance.
-
The results of the IEUBK model indicated that for young children living in the basin, there was often a greater than 5% likelihood of their BLLs exceeding the CDC criterion.
-
Although generally satisfying Superfund guidelines, available site-specific information about subsistence lifestyle exposures, such as consumption of the water potato by Coeur d’Alene tribe members was not adequately addressed. Further, exposures from sources outside the residential environment, such as during recreational swimming, during water sports, and from consumption of local produce, were not fully addressed in the assessment. The existence of additional routes of exposure may account for the finding of higher than predicted BLLs in children in the lower Coeur d’Alene River basin.
CONCLUSIONS AND RECOMMENDATIONS
This committee was charged with examining the assessment and apportionment of risks to humans from multiple contaminant exposures related to waste site sources as well as other sources (for example, lead exposure via soil and house paint dust). Other relevant components of the charge included the following: “What techniques should be used to identify contaminants of concern and estimate the human health risks attributable to waste-site sources? In this case, were risks attributable to sources other than mining and smelting activities adequately analyzed?”
The committee has reached several conclusions and recommendations.
Conclusion 1
Human health risk estimates generated for the basin were developed following EPA guidance. Intakes of lead to which current and future populations of children might be exposed were estimated within a reasonable degree of uncertainty. Consequently, the HHRA is correct in concluding that environmental lead exposure poses elevated risk to the health of some Coeur d’Alene River basin residents.
Conclusion 2
EPA followed guidance for determining human health risk from exposure to metals. Arsenic-related excess cancer risks potentially exceed one in a million throughout the Coeur d’Alene River basin. One subpopulation had estimated arsenic-related excess cancer risk exceeding 1 in 10,000. Following EPA guidance, risk estimates for metals other than arsenic and lead (antimony, cadmium, iron, manganese, mercury, and zinc) considered individually were sufficiently low to be excluded from subsequent analysis.
Use of risk estimates derived from modeling techniques is appropriate in the absence of human data. However, given the magnitude and costs of the remedial activities driven by these model-based risk estimates, the availability of biological indicators of actual human exposure to arsenic would substantially strengthen the justification for arsenic remediation.
Recommendation
The risks of arsenic in the Coeur d’Alene River basin were determined by estimating human exposures based on arsenic concentrations in environmental samples. The committee recommends that EPA continue to support research on biomarkers of human arsenic exposure as these could strengthen exposure evaluations in future HHRAs.
Conclusion 3
EPA’s analyses consider aggregate risks from multiple contaminant exposures in a manner consistent with current risk assessment practices.
The agency has also analyzed how the risks of elevated BLLs are associated with exposures from waste materials and leaded paint to a greater extent than is normally done for such a site. Currently accepted risk assessment methods do not include procedures for such apportionment of risks, and EPA has not attempted such a quantitative apportionment. However, their analyses do provide support for the conclusion that lead associated
with mining wastes is a significant source of increased BLLs, although lead paint is also a significant source for children likely to be exposed to that source.
Conclusion 4
There are logical reasons to believe that yard remediations decrease exposure to lead, but the scientific evidence supporting substantial beneficial effects is currently weak. Similarly, there is suggestive evidence of efficacy within the Bunker Hill box and river basin. Thus, the strategy for yard remediation is supportable. However, the long-term effectiveness of this remedy in the Coeur d’Alene River basin is questionable because of the possibility, even likelihood, of recontamination.
Recommendation
Long-term support of institutional controls programs should be provided to avoid undue human health risks from recontamination. Moreover, an evaluation of the efficacy of yard remediation should be supported by ongoing environmental and blood lead monitoring efforts.
Conclusion 5
Universal blood lead screening of children aged 1-4 years is indicated for this community given the prevalence of high levels of environmental lead. The current practice of annual fixed-site screening is suboptimal and produces results with too much potential for selection bias to evaluate public health intervention strategies used in the basin.
Shifting the design from a fixed site to a more widespread screening program utilizing the local health care community likely would increase participation. This type of screening program would provide a participant population that is less likely to be biased. Such a practice could be timed to coincide with other medically indicated health care screening tests conducted by primary care physicians. For example, screening for iron deficiency anemia commonly is conducted for children 1-5 years of age by performing a complete blood count. Blood lead screening could be timed to coincide with this blood draw, thereby minimizing inconvenience to the family and child. Linking the screening program to pediatric well-child visits likely will increase participation, provide built-in follow up for children with elevated BLLs, and be more convenient for families. These health surveillance activities could be conducted or sponsored by local, state, or federal (for example, ATSDR) entities.
Recommendation
The committee recommends that annual blood lead screening of all children aged 1-4 years living in the basin be initiated in conjunction with local health care providers. Results should be used to evaluate the efficacy of the environmental interventions.
Conclusion 6
American Indians who practice traditional lifestyles likely would have higher risks than other residents of the Coeur d’Alene River basin. The contamination itself likely interferes with the ability of tribal members to live subsistence lifestyles.
The committee agrees with relevant statements in the HHRA—for example, that “it is clear that a subsistence-based lifestyle requires environmental lead levels orders of magnitude lower than those measured throughout the floodplain of the Coeur d’Alene River,” and the conclusion that “Estimated lead intake rates for these scenarios are too high to predict BLLs with confidence. Predictions for BLLs associated with subsistence activities … would significantly exceed all health criteria for children or adults.”
Conclusion 7
There is strong scientific evidence that living in or near a Superfund site is associated with increased psychological stress. Chronic psychological stress may have health effects in addition to those related to chemical exposures.
Recommendation
Health interventions that address chronic stress may have significant community benefits. These should be implemented before, or concurrent with, cleanup efforts.
Conclusion 8
Children of aged 1-4 years are the group at highest risk for lead exposure. The committee found it inappropriate that the HHRA presented aggregate data on childhood lead screening for children aged 0-9 years of age.
Children less than 1 year old are at very low risk for lead poisoning because of their relative lack of mobility. Likewise, hand-to-mouth activity falls dramatically at about 4 years of age. Children 5-9 years of age are less
likely to have elevated lead levels. Although in many cases the data in the HHRA were further stratified to 0-5 years and 6-9 years, there was an inexplicable tendency to lump these age groups together. We strongly discourage such a practice because it is misleading and tends to underestimate the risk among the correct target group.
REFERENCES
AAP (American Academy of Pediatrics). 1995. Lead Screening Practices of Pediatricians. Periodic Survey of Fellows No. 28. Division of Health Policy Research, American Academy of Pediatrics. July 1995 [online]. Available: http://www.aap.org/research/periodicsurvey/ps28exs.htm [accessed Feb. 4, 2005].
Aleksandrov, A.A., O.N. Polyakova, and A.S. Batuev. 2001. The effects of prenatal stress on learning in rats in a Morris maze. Neurosci. Behav. Physiol. 31(1):71-74.
Aschengrau, A., A. Beiser, D. Bellinger, D. Copenhafer, and M. Weitzman. 1994. The impact of soil lead abatement on urban children’s BLLs: Phase II results from the Boston lead-In-Soil Demonstration Project. Environ. Res. 67(2):125-148.
Aschengrau, A., A. Beiser, D. Bellinger, D. Copenhafer, and M. Weitzman. 1997. Residential lead-based-paint hazard remediation and soil lead abatement: Their impact among children with mildly elevated BLLs. Am. J. Public Health 87(10):1698-1702.
ATSDR (Agency for Toxic Substances and Disease Registry). 1995. Public Health Assessment, National Zinc Company, Bartlesville, Washington County, OK. Cerclis No. OKD000829440. U.S. Department of Health and Human Services, Public Health Service, Agency for Toxic Substances and Disease Registry, Atlanta, GA. February 21, 1995 [online]. Available: http://www.atsdr.cdc.gov/HAC/PHA/zinc/nzc_toc.html [accessed Feb. 2, 2005].
ATSDR (Agency for Toxic Substances and Disease Registry). 2000. Coeur d’Alene River Basin Environmental Health Exposure Assessment, Final Report. U.S. Department of Health and Human Services, Agency for Toxic Substances and Disease Registry, Division of Health Studies, Atlanta, GA.
ATSDR (Agency for Toxic Substances and Disease Registry). 2004a. Report To Congress; Tar Creek Superfund Site, Ottawa County, Oklahoma. Centers for Disease Control and Prevention, Agency for Toxic Substances and Disease Registry, Atlanta, GA [online]. Available: http://www.atsdr.cdc.gov/sites/tarcreek/tarcreekreport-toc.html [accessed May 12, 2005].
ATSDR (Agency for Toxic Substances and Disease Registry). 2004b. Final Report: Follow-Up Study to Investigate BLLs in Children in Galena, Kansas. Kansas Department of Health and Environment, Topeka, KS. December 2004.
Bates, M.N., A.H. Smith, and K.P. Cantor. 1995. Case-control study of bladder cancer and arsenic in drinking water. Am. J. Epidemiol. 141(6):523-530.
Battelle. 1995. Review of Studies Addressing Lead Abatement Effectiveness. EPA 747-R-95-006. Prepared by Battelle, Columbus, OH, for the Technical Programs Branch, Chemical Management Division, Office of Pollution Prevention and Toxics, U.S. Environmental Protection Agency, Washington, DC. July.
Battelle. 1998. Review of Studies Addressing Lead Abatement Effectiveness: Updated Edition. EPA 747-B-98-001. Prepared by Battelle, Columbus, OH, for the Technical Programs Branch, Chemical Management Division, Office of Pollution Prevention and Toxics. U.S. Environmental Protection Agency, Washington, DC [online]. Available: http://www.epa.gov/lead/finalreport.pdf [accessed Feb. 3, 2005].
Bellinger, D., A. Leviton, C. Waternaux, H. Needleman, and M. Rabinowitz. 1987. Longitudinal analyses of prenatal and postnatal lead exposure and early cognitive development. N. Engl. J. Med. 316(17):1037-1043.
Bellinger, D.C., K.M. Stiles, and H.L. Needleman. 1992. Low-level lead exposure, intelligence and academic achievement: A long-term follow-up study. Pediatrics 90(6):855-861.
Bellinger, D., A. Leviton, E. Allred, and M. Rabinowitz. 1994. Pre- and postnatal lead exposure and behavior problems in school-aged children. Environ. Res. 66(1):12-30.
Breggin, L., J. Pendergrass, and J. van Berg. 1999. Protecting Public Health at Superfund Sites: Can Institutional Controls Meet the Challenge? Environmental Law Institute [online]. Available: http://www.elistore.org/reports_detail.asp?ID=543 [accessed April 19, 2005].
Bromet, E., and L. Dunn. 1981. Mental health of mothers nine months after the Three Mile Island accident. Urban Soc. Change Rev. 14(2):12-15.
Calder, I.C., E.J. Maynard, and J.S. Heyworth. 1994. Port Pirie lead abatement program, 1992. Environ. Geochem. Health 16(3/4):137-145.
Canfield, R.L., C.R. Henderson, Jr., D.A. Cory-Slechta, C.F. Cox, T.A. Jusko, and B.P. Lanphear. 2003. Intellectual impairment in children with blood lead concentrations below 10 microg per deciliter. N. Engl. J. Med. 348(16):1517-1526.
Caussy, D. 2003. Case studies of the impact of understanding bioavailability: Arsenic. Ecotoxicol. Environ. Saf. 56(1):164-173.
CDC (Centers for Disease Control and Prevention). 1991. Preventing Lead Poisoning in Young Children: A Statement by the Centers for Disease Control. U.S. Department of Health and Human Services, Public Health Service, Centers for Disease Control and Prevention, Atlanta, GA [online]. Available: http://aepo-xdv-www.epo.cdc.gov/wonder/prevguid/p0000029/p0000029.asp [accessed Feb. 2, 2005].
CDC (Centers for Disease Control and Prevention). 2004. Children’s BLLs in the United States. Childhood lead Poisoning Prevention Program, National Center for Environmental Health, Centers for Disease Control and Prevention, Atlanta, GA [online]. Available: http://www.cdc.gov/nceh/lead/research/kidsBLL.htm [accessed Feb. 4, 2005].
Cheng, Y., J. Schwartz, D. Sparrow, A. Aro, S.T. Weiss, and H. Hu. 2001. Bone lead and blood lead level in relation to baseline blood pressure and the prospective development of hypertension: The Normative Aging Study. Am. J. Epidemiol. 153(2):164-171.
Cory-Slechta, D.A., M.B. Virgolini, M. Thiruchelvam, D.D. Weston, and M.R. Bauter. 2004. Maternal stress modulates the effects of developmental lead exposure. Environ. Health Perspect. 112(6):717-730.
Davidson, L.M., I. Fleming, and A. Baum. 1986. Post-traumatic stress as a function of chronic stress and toxic exposure. Pp. 57-77 in Trauma and Its Wake: Vol. 2. Traumatic Stress Theory, Research and Intervention, C.R. Figley, ed. New York: Brunner/Mazel.
Dietrich, K.N., K.M. Krafft, R.L. Bornschein, P.B. Hammond, O. Berger, P.A. Succop, and M. Bier. 1987. Low-level fetal lead exposure effect on neurobehavioral development in early infancy. Pediatrics 80(5):721-730.
Duggan, M.J., and M.J. Inskip. 1985. Childhood exposure to lead in surface dust and soil: A community health problem. Public Health Rev. 13(1-2):1-54.
Duggan, M.J., M.J. Inskip, S.A. Rundle, and J.S. Moorcroft. 1985. Lead in playground dust and on the hands of schoolchildren. Sci. Total Environ. 44(1):65-79.
Eagle-Picher (Eagle-Picher Holdings, Inc). 2002. Form 10-K/A No. 2, Annual Report Pursuant to Section 13 or 15(d) of the Securities Exchange Act of 1934 For the Fiscal Year Ended November 30, 2001. Commission File No. 333-49957-01. Eagle-Picher Holdings, Inc., Cincinnati, OH. September 20, 2002 [online]. Available: http://www.eaglepicher.com/NR/rdonlyres/67C23B14-4534-422C-A6ED-6E213DC3A2DA/0/2001_10K.pdf [accessed Feb. 2, 2005].
Edelstein, M.R. 1988. Contaminated Communities: The Social and Psychological Impact of Residential Toxic Exposure. Boulder, CO: Westview Press.
EMC2 and Phelps Dodge Corporation. 2001. First Five-Year Review Report For The National Zinc Corporation Site, Bartlesville, Oklahoma, OKD000829440. Prepared by Environmental Management Consultants Corporation and Phelps Dodge Corporation, for The Oklahoma Department of Environmental Quality, Oklahoma City, OK. October 2001 [online]. Available: http://www.epa.gov/superfund/sites/fiveyear/f02-06015.pdf [accessed Feb. 3, 2005].
EPA (U.S. Environmental Protection Agency). 1986. Air Quality Criteria for Lead, Vol. IV. EPA 600/8-83-028dF. Environmental Criteria and Assessment Office, Office of Health and Environmental Assessment, Office of Research and Development, U.S. Environmental Protection Agency, Research Triangle Park, NC. June 1986.
EPA (U.S. Environmental Protection Agency). 1989. Risk Assessment Guidance for Superfund: Vol. 1. Human Health Evaluation Manual (Part A). Interim Final. EPA/540/1-89/002. Office of Emergency and Remedial Response, U.S. Environmental Protection Agency, Washington, DC. December 1989 [online]. Available: http://www.epa.gov/superfund/programs/risk/ragsa/index.htm [accessed Feb. 3, 2005].
EPA (U.S. Environmental Protection Agency). 1991a. Risk Assessment Guidance for Superfund Volume 1: Human Health Evaluation Manual; Supplemental Guidance; “Standard Default Exposure Factors.” Interim Final. OSWER Directive 9285.6-03. PB91-921314. Office of Emergency and Remedial Response, Toxic Integration Branch, U.S. Environmental Protection Agency, Washington, DC [online]. Available: http://www.hanford.gov/dqo/project/level5/hhems.pdf [accessed Feb. 3, 2005].
EPA (U.S. Environmental Protection Agency). 1991b. Record of Decision (ROD) - Bunker Hill Mining and Metallurgical Complex, EPA ID: IDD048340921 OU1 Smelterville, ID. EPA/ROD/R10-91/028. U.S. Environmental Protection Agency. Washington, Region 10. August 30, 1991 [online]. Available: http://www.epa.gov/superfund/sites/rods/fulltext/r1091028.pdf [accessed Feb. 4, 2005].
EPA (U.S. Environmental Protection Agency). 1992a. Guidance on Risk Characterization for Risk Managers and Risk Assessors. Memorandum to Assistant and Regional Administrators, from F. Henry Habicht II, Deputy Administrator, Office of the Administrator, U.S. Environmental Protection Agency, Washington, DC. February 26, 1992 [online]. Available: http://www.epa.gov/superfund/programs/risk/habicht.htm [accessed Feb. 3, 2005].
EPA (U.S. Environmental Protection Agency). 1992b. Supplemental Guidance to RAGS: Calculating the Concentration Term. Publication. No. 9285.7-081. PB92-963373. Office of Solid Waste and Emergency Response, U.S. Environmental Protection Agency, Washington, DC [online]. Available: http://www.deq.state.or.us/wmc/tank/documents/epa-ucls.pdf [accessed Feb. 3, 2005]
EPA (U.S. Environmental Protection Agency). 1993a. Superfund’s Standard Default Exposure Factors for the Central Tendency and Reasonable Maximum Exposure. Preliminary review draft. U.S. Environmental Protection Agency, Washington, DC.
EPA (U.S. Environmental Protection Agency). 1993b. Urban Soil Lead Abatement Demonstration Project, Vol. II., Part 2. Boston Report. EPA/600/AP-93/001b. Environmental Criteria and Assessment Office, Office of Health and Environmental Assessment, Office of Research and Development, U.S. Environmental Protection Agency, Washington, DC. July 1993.
EPA (U.S. Environmental Protection Agency). 1993c. Urban Soil Lead Abatement Demonstration Project. Vol. III: Part 2. Baltimore Report. EPA/600/AP-93/001c. Environmental Criteria and Assessment Office, Office of Health and Environmental Assessment, Office of Research and Development. U.S. Environmental Protection Agency, Washington, DC. July 1993.
EPA (U.S. Environmental Protection Agency). 1993d. Urban Soil Lead Abatement Demonstration Project. Vol. IV. Cincinnati Report. EPA/600/AP-93/001d. Environmental Criteria and Assessment Office, Office of Health and Environmental Assessment, Office of Research and Development, U.S. Environmental Protection Agency, Washington, DC. July 1993.
EPA (U.S. Environmental Protection Agency). 1994. Revised Interim Soil Lead Guidance for CERCLA Sites and RCRA Corrective Action Facilities. Memorandum: OSWER Directive 9355.4-12. Office of Solid Waste and Emergency Response, U.S. Environmental Protection Agency, Washington, DC [online]. Available: http://www.epa.gov/superfund/programs/lead/products/oswerdir.pdf [accessed Feb. 3, 2005].
EPA (U.S. Environmental Protection Agency). 1995. Guidance for Risk Characterization. Science Policy Council, U.S. Environmental Protection Agency. Washington, DC. February 1995 [online]. Available: http://epa.gov/osa/spc/htm/rcguide.htm [accessed Feb. 3, 2005].
EPA(U.S. Environmental Protection Agency). 1996a. Urban Soil Lead Abatement Demonstration Project. EPA Data Dictionary with Associated Data Sets. EPA/600/C-96/002. Office of Research and Development, U.S. Environmental Protection Agency, Washington, DC. September 1996.
EPA (U.S. Environmental Protection Agency). 1996b. EPA Superfund Record of Decision: Cherokee County, EPA ID: KSD980741862, OU 07, Cherokee County, KS. EPA/ROD/ R07-96/082. Record of Decision System, Superfund Information System, U.S. Environmental Protection Agency [online]. Available: http://www.epa.gov/superfund/sites/rods/fulltext/r0796082.pdf [accessed April 19, 2005].
EPA (U.S. Environmental protection Agency). 1999. EPA Superfund Record of Decision: California Gulch, EPA ID: COD980717938, OU 09, Leadville, CO. EPA/ROD/R08-99/ 055. U.S. Environmental Protection Agency, Denver, CO [online]. Available: http://www.epa.gov/superfund/sites/rods/fulltext/r0899055.pdf [accessed May 17, 2005].
EPA (U.S. Environmental Protection Agency). 2000a. Second Five-Year Review Report for Cherokee County Superfund Site, Cherokee County, Kansas. U.S. Environmental Protection Agency, Region 7, Kansas City, KS. September 2000 [online] Available: http://www.epa.gov/superfund/sites/fiveyear/f00-07001.pdf [accessed April 19, 2005].
EPA (U.S. Environmental Protection Agency). 2000b. Five-Year Review, Tar Creek Superfund Site, Ottawa County, Oklahoma. U.S. Environmental Protection Agency, Region 6. April 2000 [online]. Available: http://www.epa.gov/earth1r6/6sf/pdffiles/5yeartarcreek.pdf [accessed April 18, 2005].
EPA (U.S. Environmental Protection Agency). 2002. First Five-Year Review Report for the Oronogo-Duenweg Mining Belt Site, Jasper County, Missouri. September 2002. U.S. Environmental Protection Agency, Region 7, Kansas City, KS [online]. Available: http://www.epa.gov/superfund/sites/fiveyear/f02-07007.pdf [accessed Feb. 3, 2005].
EPA(U.S. Environmental Protection Agency). 2004a. IRIS Database for Risk Assessment. National Center for Environmental Assessment, Office of Research and Development, U.S. Environmental Protection Agency [online]. Available: http://www.epa.gov/iris/ [accessed Feb. 3, 2005].
EPA (U.S. Environmental Protection Agency). 2004b. EPA Responses to NAS Questions (May 25, 2004).
EPA (U.S. Environmental protection Agency). 2005. Superfund Program Cleanup Proposal Butte Priority Soils Operable Unit of the Silver Bow Creek/Butte Area Superfund Site. U.S. Environmental Protection Agency Region 8, Montana Office [online]. Available: http://www.epa.gov/region8/superfund/sites/mt/FinalBPSOUProposedPlan.pdf [accessed May 17, 2005].
Factor-Litvak, P. 1992. Maternal and Fetal Outcomes Following Prenatal Exposure to Lead. Ph.D. Dissertation, Columbia University, New York.
Figley, C.R. 1986. Traumatic stress: The role of the family and social support system. Pp. 39-54 in Trauma and Its Wake: Vol. 2. Traumatic Stress Theory, Research and Intervention, C.R. Figley, ed. New York: Brunner/Mazel.
Fleming, R., A. Baum, M.M. Gisriel, and R.J. Gatchel. 1982. Mediating influences of social support on stress at Three Mile Island. J. Human Stress 8(3):14-22.
Frisone, D.F., C.A. Frye, and B. Zimmerberg. 2002. Social isolation stress during the third week of life has age-dependent effects on spatial learning in rats. Behav. Brain Res. 128(2):153-160.
Gagne, D. 1994. BLLs Noranda children following removal of smelter-contaminated yard soil. Can. J. Public Health 85(3):163-166.
Gibbs, M.S. 1986. Psychopathological consequences of exposure to toxins in the water supply. Pp. 47-70 in Exposure to Hazardous Substances: Psychological Parameters, A.H. Lebovits, A. Baum, and J.E. Singer, eds. Advances in Environmental Psychology, Vol. 6. Hillsdale, NJ: L. Erlbaum Associates.
Golier, J., and R. Yehuda. 1998. Neuroendocrine activity and memory-related impairments in posttraumatic stress disorder. Dev. Psychopathol. 10(4):857-869.
Goulet, L., J. Gaudreau, and A. Messier. 1996. Results of a lead decontamination program. Arch. Environ. Health 51(1):68-72.
Graziano, J.H., E.S. Siris, N. LoIacono, S.J. Silverberg, and L. Turgeon. 1985. 2,3-Dimercaptosuccinic acid as an antidote for lead intoxication. Clin. Pharmacol. Ther. 37(4):431-438.
Graziano, J.H., D. Popovac, P. Factor-Litvak, P. Shrout, J. Kline, M.J. Murphy, Y. Zhao, A. Mehmeti, X. Ahmedi, B. Rajovic, Z. Zvicer, D.U. Nenezic, N.J. Lolacono, and Z. Stein. 1990. Determinants of elevated blood lead during pregnancy in a population surrounding a lead smelter in Kosovo, Yugoslavia. Environ. Health Perspect. 89:95-100.
Graziano, J.H., N.J. Lolacono, T. Moulton, M.E. Mitchell, V. Slavkovich, and C. Zarate. 1992. Controlled study of meso-2,3-dimercaptosuccinic acid for the management of childhood lead intoxication. J. Pediatr. 120(1):133-139.
Guilarte, T.R., C.D. Toscano, J.L. McGlothan, and S.A. Weaver. 2003. Environmental enrichment reverses cognitive and molecular deficits induced by developmental lead exposure. Ann. Neurol. 53(1):50-56.
Harper, B.L., B. Flett, S. Harris, C. Abeyta, and F. Kirschner. 2002. The Spokane Tribe’s multipathway subsistence exposure scenario and screening level RME. Risk Anal. 22(3): 513-526.
Harris, S.G., and B.L. Harper. 1997. A Native American exposure scenario. Risk Anal. 17(6):789-795.
Harris, S., and B.L. Harper. 2001. Lifestyles, diets, and Native American exposure factors related to possible lead exposures and toxicity. Environ. Res. 86(2):140-148.
Heyworth, J., I. Calder, D. Roder, P. Baghurst, and T. McMichael. 1993. Evaluation of the lead Decontamination Programme in the lead smelting town of Port Pirie (South Australia). Int. J. Environ. Health Res. 3(3):149-160.
Hilts, S.R. 2003. Effect of smelter emission reductions on children’s BLLs. Sci. Total Environ. 303(1-2):51-58.
Hora, S.C. 1992. Acquisition of expert judgment: Examples from risk assessment. J. Energ. Eng. 118(2):136-148.
Idaho Department of Commerce. 2004. County and Community Profiles of Idaho [online]. Available: http://www.idoc.state.id.us/idcomm/profiles/ [accessed Feb. 2, 2005].
Janakiraman, V., A. Ettinger, A. Mercado-Garcia, H. Hu, and M. Hernandez-Avila. 2003. Calcium supplements and bone resorption in pregnancy: A randomized crossover trial. Am. J. Prev. Med. 24(3):260-264.
Karagas, M.R., T.A. Stukel, J.S. Morris, T.D. Tosteson, J.E. Weiss, S.K. Spencer, E.R. Green berg. 2001. Skin cancer risk in relation to toenail arsenic concentrations in a U.S. population-based case-control study. Am. J. Epidemiol. 153(6):559-565.
Karagas, M.R., T.D. Tosteson, J.S. Morris, E. Demidenko, L.A. Mott, J. Heaney, and A. Schned. 2004. Incidence of transitional cell carcinoma of the bladder and arsenic exposure in New Hampshire. Cancer Causes Control. 15(5):465-472.
Kimbrough, R.D., M. LeVois, and D.R. Webb. 1994. Management of children with slightly elevated BLLs. Pediatrics 93(2):188-191.
Kissel, J.C., K.Y. Richter, and R.A. Fenske. 1996. Factors affecting soil adherence to skin in hand-press trials. Bull. Environ. Contam. Toxicol. 56(5):722-728.
Kroll-Smith, J.S., and S.R. Couch. 1990. The Real Disaster is Above Ground: A Mine Fire and Social Conflict. Lexington, KY: University of Kentucky Press.
Langlois, P., L. Smith, S. Fleming, R. Gould, V. Goel, and B. Gibson. 1996. BLLs in Toronto children and abatement of lead-contaminated soil and house dust. Arch. Environ. Health 51(1):59-67.
Lanphear, B.P., T.D. Matte, J. Rogers, R.P. Clickner, B. Dietz, R.L. Bornschein, P. Succop, K.R. Mahaffey, S. Dixon, W. Galke, M. Rabinowitz, M. Farfel, C. Rohde, J. Schwartz, P. Ashley, and D.E. Jacobs. 1998. The contribution of lead-contaminated house dust and residential soil to children’s BLLs. A pooled analysis of 12 epidemiologic studies. Environ. Res. 79(1):51-68.
Lanphear, B.P., K. Dietrich, P. Auinger, and C. Cox. 2000. Cognitive deficits associated with blood lead concentrations <10 microg/dL in US children and adolescents. Public Health Rep. 115(6):521-529.
Lanphear, B.P., P. Succop, S. Roda, and G. Henningsen. 2003. The effect of soil abatement on BLLs in children living near a former smelting and milling operation. Public Health Rep. 118(2):83-91.
LCCCHD (Lewis and Clark City-County Health Department). 2005. East Helena Lead Education and Abatement Program. Lewis and Clark City-County Health Department, East Helena, MT [online]. Available: http://www.co.lewis-clark.mt.us/health/lead.php [accessed May 17, 2005].
LeDoux, J.E. 2002. Synaptic Self: How Our Brains Become Who We Are. New York: Viking.
Levine, A.G., and R.A. Stone. 1986. Threats to people and what they value: Residents’ perceptions of the hazards of Love Canal. Pp. 109-130 in Exposure to Hazardous Substances: Psychological Parameters, A.H. Lebovits, A. Baum, and J.E. Singer, eds. Advances in Environmental Psychology, Vol. 6. Hillsdale, NJ: L. Erlbaum Associates.
Lev-Ran, A. 2001. Human obesity: An evolutionary approach to understanding our bulging waistline. Diabetes Metab. Res. Rev. 17(5):347-362.
Lewis, D.R., J.W. Southwick, R. Ouellet-Hellstrom, J. Rench, and R.L. Calderon. 1999. Drinking water arsenic in Utah: A cohort mortality study. Environ. Health Perspect. 107(5):359-365.
Lorenzana, R.M., R. Troast, M. Mastriano, M.H. Follansbee, and G.L. Diamond. 2003. Lead intervention and pediatric BLLs at hazardous waste sites. J. Toxicol. Environ. Health A 66(10):871-893.
Louekari, K., U.M. Mroueh, L. Maidell-Munster, S. Valkonen, T. Tuomi, and K. Savolainena. 2004. Reducing the risks of children living near the site of a former lead smeltery. Sci. Total Environ. 319(1-3):65-75.
Louis, E.D., E.C. Jurewicz, L. Applegate, P. Factor-Litvak, M. Parides, L. Andrews, V. Slavkovich, J.H. Graziano, S. Carroll, and A. Todd. 2003. Association between essential tremor and blood lead concentration. Environ. Health Perspect. 111(14):1707-1711.
Louis, E.D., L. Applegate, J.H. Graziano, M. Parides, V. Slavkovich, and H.K. Bhat. In press. Interaction between blood lead concentration and delta-amino-levulinic acid dehydratase gene polymorphisms increases the odds of essential tremor. Movement Disorders.
Markowitz, M.E., P.E. Bijur, H.A. Ruff, K. Balbi, and J.F. Rosen. 1996. Moderate lead poisoning: Trends in BLLs in unchelated children. Environ. Health Perspect. 104(9):968-972.
Maynard, E., R. Thomas, D. Simon, C. Phipps, C. Ward, and I. Calder. 2003. An evaluation of recent BLLs in Port Pirie, South Australia. Sci. Total Environ. 303(1-2):25-33.
McDermott, R. 1998. Ethics, epidemiology and the thrifty gene: Biological determinism as a health hazard. Soc. Sci. Med. 47(9):1189-1195.
McEwen, B.S. 2001. Plasticity of the hippocampus: Adaptation to chronic stress and allostatic load. Ann. NY Acad. Sci. 933:265-277.
MDHSS/ATSDR (Missouri Department of Health and Senior Services and Agency for Toxic Substances and Disease Registry). 2004. Jasper County, Missouri Superfund Site Childhood Follow-up Lead Exposure Study. Missouri Department of Health and Senior Services and Agency for Toxic Substances and Disease Registry [online]. Available: http://www.dhss.mo.gov/EnvirConsult/Jasper_report_1.htm [accessed Feb. 2, 2005].
Meyer, M.A., and J.M. Booker. 1991. Eliciting and Analyzing Expert Judgment: A Practical Guide. New York: Academic Press.
Morrison, A.L. 2003. An assessment of the effectiveness of lead pollution reduction strategies in North Lake Macquarie, NSW, Australia. Sci. Total Environ. 303(1-2):125-138.
Needleman, H.L., J.A. Riess, M.J. Tobin, G.E. Biesecker, and J.B. Greenhouse. 1996. Bone lead levels and delinquent behavior. JAMA 275(5):363-369.
Needleman, H.L., C. McFarland, R.B. Ness, S.E. Fienberg, and M.J. Tobin. 2002. Bone lead levels in adjudicated delinquents. A case control study. Neurotoxicol. Teratol. 24(6):711-717.
Nelson, C.A., and L.J. Carver. 1998. The effects of stress and trauma on brain and memory: A view from developmental cognitive neuroscience. Dev. Psychopathol. 10(4):793-809.
Niemuth, N.A., B.J. Wood, and B.D. Schultz. 2001. Estimated change in blood lead concentration in control populations. Arch. Environ. Health 56(6):542-551.
Nightingale, S.L. 1991. Succimer (DMSA) approved for severe lead poisoning. JAMA 265(14): 1802.
NRC (National Research Council). 1999. Arsenic in Drinking Water. Washington, DC: National Academy Press.
NRC (National Research Council). 2001. Arsenic in Drinking Water 2001 Update. Washington, DC: National Academy Press.
NTP (National Toxicology Program). 2000. 9th Report on Carcinogens. National Toxicology Program, U.S. Department of Health and Human Services, Research Triangle Park, NC.
NTP (National Toxicology Program). 2005. 11th Report on Carcinogens. Fact Sheet. National Toxicology Program, U.S. Department of Health and Human Services, Research Triangle Park, NC [online]. Available: http://ntp.niehs.nih.gov/ntpweb/index.cfm?objectid=BD1528EF-F1F6-975E-7C0FAE691D3FD9EB [accessed May 24, 2005].
O’Connor, T.G., J. Heron, and V. Glover. 2002a. Antenatal anxiety predicts child behavioral/emotional problems independently of postnatal depression. J. Am. Acad. Child. Adolesc. Psychiatry 41(12):1470-1477.
O’Connor, T.G., J. Heron, J. Golding, M. Beveridge, and V. Glover. 2002b. Maternal antenatal anxiety and children’s behavioural/emotional problems at 4 years. Report from the Avon Longitudinal Study of Parents and Children. Br. J. Psychiatry 180:502-508.
Osedowski, M. 2001. Risk perceptions and First Nations communities. Int. J. Circumpolar Health 60(2):180-183.
Potula, V., J. Serrano, D. Sparrow, and H. Hu. 1999. Relationship of lead in drinking water to bone lead levels twenty years later in Boston men: The Normative Aging Study. J. Occup. Environ. Med. 41(5):349-355.
Rodriguez, R.R., N.T. Basta, S.W. Casteel, F.P. Armstrong, and D.C. Ward. 2003. Chemical extraction methods to assess bioavailable arsenic in soil and solid media. J. Environ. Qual. 32(3):876-884.
Rogan, W.J., K.N. Dietrich, J.H. Ware, D.W. Dockery, M. Salganik, J. Radcliffe, R.L. Jones, N.B. Ragan, J.J. Chisolm Jr., and G.G. Rhoads. 2001. The effect of chelation therapy with succimer on neuropsychological development in children exposed to lead. N. Engl. J. Med. 344(19):1421-1426.
Roizen, R. 2002. Federal agency fails to paint a convincing picture on paint. Shoshone News-Press (Feb. 9):5 [online]. Available: http://www.roizen.com/tech/paint.html [accessed Feb. 3, 2005].
Sapolsky, R.M. 2000. Stress hormones: Good and bad. Neurobiol. Dis. 7(5):540-542.
Schneider, J.S., M.H. Lee, D.W. Anderson, L. Zuck, and T.I. Lidsky. 2001. Enriched environment during development is protective against lead-induced neurotoxicity. Brain Res. 896(1-2):48-55.
Schwartz, J. 1994. Low-level lead exposure and children’s IQ: A meta-analysis and search for a threshold. Environ. Res. 65(1):42-55.
Sheldrake, S., and M. Stifelman. 2003. A case study of lead contamination cleanup effectiveness at Bunker Hill. Sci. Total Environ. 303(1-2):105-123.
Sheppard, S.C., and W.G. Evenden. 1994. Contaminant enrichment and properties of soil adhering to skin. J. Environ. Qual. 23(3):604-613.
Sousa, N., and O.F. Almeida. 2002. Corticosteroids: Sculptors of the hippocampal formation. Rev. Neurosci. 13(1):59-84.
Southerland, E. 2004. Coeur d’Alene Basin Overview of Remedial Decisions. Presentation at the First Meeting on Superfund Site Assessment and Remediation in the Coeur d’Alene River Basin, January 22, 2004, Washington, DC.
Stanek, E.J. III, and E.J. Calabrese. 1995. Soil ingestion estimates for use in site evaluations based on the best tracer method. Human Ecol. Risk Assess. 1(2):133-156.
Stefanko, M., and J. Horowitz. 1989. Attitudinal effects associated with an environmental hazard. Popul. Environ. 11(1):43-58.
Steinmaus, C., Y. Yuan, M.N. Bates, and A.H. Smith. 2003. Case-control study of bladder cancer and drinking water arsenic in the western United States. Am. J. Epidemiol. 158(12):1193-1201.
Stone, R., and A. Levine. 1985. Reactions to collective stress: Correlates of active citizen participation at Love Canal. Pp. 153-177 in Beyond the Individual: Environmental Approaches and Prevention, A. Wandersman, and R. Hess, eds. New York: Haworth Press.
TerraGraphics. 2000. Final 1999 Five Year Review Report Bunker Hill Site. Prepared for Idaho Department of Health and Welfare Division of Environmental Quality, Boise, ID, by TerraGraphics Environmental Engineering, Inc., Moscow, ID. April 2000.
TerraGraphics/URS Greiner/CH2M Hill. 2001. Final Human Health Risk Assessment for the Coeur d’Alene River Basin Extending from Harrison to Mullan on the Coeur d’Alene River and Tributaries, Remedial Investigation/Feasibility Study. Prepared for Idaho Department of Health and Welfare, Division of Health, Idaho Department of Environmental Quality, U.S. Environmental Protection Agency Region X, Seattle, WA, by Terra Graphics Environmental Engineering, Inc, URS Greiner in association with CH2M Hill [online]. Available: http://www.epa.gov/r10earth/offices/sf/BH_HHRA_final/TableOfContents.pdf [accessed Jan. 3, 2005].
Tucker, P. 2002. Report of the Expert Panel Workshop on the Psychological Responses to Hazardous Substances. Office of the Director, Division of Health Education and Promotion, Agency for Toxic Substances and Disease Registry, Atlanta, GA [online]. Available: http://www.atsdr.cdc.gov/HEC/PRHS/psych5ed.pdf [accessed May 12, 2005].
Turpeinen, R., M. Virta, and M.M. Haggblom. 2003. Analysis of arsenic bioavailability in contaminated soils. Environ. Toxicol. Chem. 22(1):1-6.
Unger, D.G., A. Wandersman, and W. Hallman. 1992. Living near a hazardous waste facility: Coping with individual and family distress. Am. J. Orthopsychiatry 62(1):55-70.
Vallee, M., S. MacCari, F. Dellu, H. Simon, M. Le Moal, and W. Mayo. 1999. Long-term effects of prenatal stress and postnatal handling on age-related glucocorticoid secretion and cognitive performance: A longitudinal study in the rat. Eur. J. Neurosci. 11(8):2906-2916.
van Wijnen, J.H., P. Clausing, and B. Brunekreef. 1990. Estimated soil ingestion by children. Environ. Res. 51(2):147-162.
von Lindern, I. 2004. Background for Assessing Lead Health Response Actions in the Silver Valley of Idaho. Presentation at the First Meeting on Superfund Site Assessment and Remediation in the Coeur d’Alene River Basin, January 22, 2004, Washington, DC.
von Lindern, I., S. Spalinger, V. Petroysan, and M. von Braun. 2003. Assessing remedial effectiveness through the blood lead: Soil/dust lead relationship at the Bunker Hill Superfund Site in the Silver Valley of Idaho. Sci. Total Environ. 303(1-2):139-170.
Wasserman, G.A., J.H. Graziano, P. Factor-Litvak, D. Popovac, N. Morina, A. Musabegovic, N. Vrenezi, S. Capuni-Paracka, V. Lekic, and E. Preteni-Redjepi. 1994. Consequences of lead exposure and iron supplementation on childhood development at age 4 years. Neurotoxicol. Teratol. 16(3):233-240.
Wasserman, G.A., B. Staghezza-Jaramillo, P. Shrout, D. Popovac, and J. Graziano. 1998. The effect of lead exposure on behavior problems in preschool children. Am. J. Public Health 88(3):481-486.
Weitzman, M., A. Aschengrau, D. Bellinger, R. Jones, J.S. Hamlin, and A. Beiser. 1993. Lead-contaminated soil abatement and urban children’s BLLs. JAMA 269(13):1647-1654.
Wright, R.O., S.W. Tsaih, J. Schwartz, A. Spiro, K. McDonald, S.T. Weiss, and H. Hu. 2003. Lead exposure biomarkers and mini-mental status exam scores in older men. Epidemiology 14(6):713-718.
Zaharia, M.D., J. Kulczycki, N. Shanks, M.J. Meaney, and H. Anisman. 1996. The effects of early postnatal stimulation on Morris water-maze acquisition in adult mice: Genetic and maternal factors. Psychopharmacology (Berl) 128(3):227-239.