3
Toxicokinetics and Modes of Action of Formaldehyde
This chapter provides the committee’s review of the draft IRIS assessment that is relevant to formaldehyde toxicokinetics, carcinogenic modes of action, pharmacokinetic models, and biologically based dose-response (BBDR) models.1 The committee comments on the Environmental Protection Agency (EPA) analysis of the fate of inhaled formaldehyde in the respiratory tract (portal of entry) and at more distant sites reached through systemic circulation, the use of formaldehyde-induced cross-links as biomarkers, and the ability of formaldehyde to cause systemic genotoxic effects. The committee also reviews EPA’s use of the computational pharmacokinetic models and BBDR models that have been developed for formaldehyde and considers EPA’s analysis of the sensitivity of low-dose BBDR-model estimates to small changes in model design or model inputs.
The discussion provided here is not intended to be exhaustive but rather focuses on the evidence presented in the draft IRIS assessment that was used to support EPA’s key conclusions. It also dwells on the inhalation pathway rather than other exposure pathways because the inhalation pathway is the focus of the draft IRIS assessment. In conducting its review, the committee attempted to answer several central questions underlying the approach taken by EPA, including the following:
-
Is formaldehyde an endogenous chemical?
-
What is the immediate fate of inhaled formaldehyde?
-
Is inhaled formaldehyde available systemically?
-
Can formaldehyde-related effects alter its toxicokinetics?
-
Are formaldehyde-induced cross-links useful biomarkers of exposure?
-
Can inhaled formaldehyde have systemic genotoxic effects?
-
Are useful computational pharmacokinetic models for formaldehyde inhalation available?
-
Has a mode of action for formaldehyde carcinogenesis been identified?
-
What is the status of BBDR models for formaldehyde?
-
Should the BBDR models available for formaldehyde be used in EPA’s quantitative assessment?
Some of those questions could be answered by weighing the evidence from research studies considered in the draft IRIS assessment; others could not be answered by the committee with high confidence.
Overall, the committee found that the chapters describing the toxicokinetics, modes of action, and various models are well organized and that the draft IRIS assessment accurately reflects the current understanding of the toxicokinetics of inhaled formaldehyde and provides a thorough review of the metabolism, cytotoxicity, and genotoxicity of formaldehyde. The literature review in the draft IRIS assessment appears to be up to date and to include all major and recent studies published as of the release date.
TOXICOKINETICS
Is Formaldehyde an Endogenous Chemical?
The committee notes that EPA satisfactorily describes the current understanding of endogenous formaldehyde. It is well established that formaldehyde is produced endogenously by enzymatic and nonenzymatic pathways or as a detoxification product of xenobiotics during cellular metabolism (ATSDR 1999). There is also broad agreement that formaldehyde originating from metabolic or dietary sources is normally present at low concentrations in all tissues, cells, and bodily fluids. The concentration of endogenous formaldehyde in the blood of rats, monkeys, and humans is about 0.1 mM (Heck et al. 1985; Casanova et al. 1988). Background concentrations in the liver and nasal mucosa of the rat are 2-4 times those in the blood (Heck et al. 1982). Endogenous tissue formaldehyde concentrations are similar to concentrations (about 0.05 mM) that induce genotoxicity and cytolethality in vitro (Heck and Casanova 2004).
Heck et al. (1985) did not observe an increase in blood formaldehyde concentrations in rats and humans after exposure to inhaled formaldehyde at 14.4 ppm (2 hr) or 1.9 ppm (40 min), respectively. Subchronic studies conducted in rhesus monkeys have also shown that blood formaldehyde concentration was not measurably altered by exposure to airborne formaldehyde at 6 ppm for 6 hr/day 5 days/week for 4 weeks (Casanova-Schmitz et al. 1984).
Formaldehyde has also been measured in exhaled breath, but the interpretation of some measurements made with mass spectrometry has been questioned (Spanel and Smith 2008; Schripp et al. 2010). Spanel and Smith (2008) showed that a trace contaminant (up to 1%) of the reagent gas used in real-time mass-spectrometric methods—specifically proton-transfer reaction mass spectrometry (PTR-MS) and selected ion flow tube mass spectrometry (SIFT-MS)—reacts with endogenous methanol and ethanol that is normally found in exhaled breath to produce the same main ion (mass-to-charge ratio of 31) as is used to measure formaldehyde. Thus, they concluded that up to 5 ppb of the formaldehyde concentration determined in the exhaled breath of humans reported in earlier studies that did not account for this confounding may be due to methanol or ethanol and not formaldehyde; that is, 1% of total background concentrations of methanol or ethanol of about 500 ppb would be misclassified as formaldehyde. The committee concurs with EPA’s concerns as to whether some published exhaled-breath measurements of formaldehyde are analytically valid. The committee also notes that this methodologic problem is inconsistently addressed by EPA in its reanalysis of the exhaled-breath experiments. The committee concludes, however, that regardless of the methodologic issue related to breath analysis, formaldehyde is normally present at a few parts per billion in exhaled breath after the measurement error associated with a trace contaminant in the reagent gas used in previous mass-spectrometric methods is taken into account.
The committee concludes that formaldehyde is an endogenous compound and that this finding complicates assessments of the risk posed by inhalation of formaldehyde. The committee emphasizes that the natural presence of various concentrations of formaldehyde in target tissues remains an important uncertainty with regard to assessment of the additional dose received by inhalation.
What Is the Immediate Fate of Inhaled Formaldehyde?
Formaldehyde has been the subject of multiple toxicokinetic studies in rodents, dogs, and monkeys and of numerous in vitro and biomonitoring studies in humans. Although there may be quantitative differences between species, the fate of formaldehyde is qualitatively similar among species. The draft IRIS assessment provides an extensive and thorough review of the literature on the fate of formaldehyde in the body.
Formaldehyde is highly water-soluble and exists in water almost exclusively in a reversible hydrated form (methanediol). Thus, less than 0.1% of formaldehyde can be considered “free” once it enters the body. Formaldehyde is highly reactive at the site of entry and reacts readily with macromolecules, including DNA to form DNA-protein (DPX) and DNA-DNA (DDX) cross-links. Formaldehyde is oxidized to formate by a low-Km (400-μM) mitochondrial aldehyde dehydrogenase-2 (ALDH2) or via a two-enzyme system that converts nonenzymatically formed glutathione adducts (S-hydroxymethylglutathione) to the intermediate S-formylglutathione, which is then metabolized to formate and
glutathione by S-formylglutathione hydrolase. Those metabolic processes contribute to the short biologic half-life of formaldehyde.
Inhaled formaldehyde is absorbed primarily in the upper airways because of its high water solubility, metabolism, and reactivity. Inhaled formaldehyde in the nasal cavity initially contacts the mucus layer lining the epithelium (Figure 3-1). Once in the mucus layer, formaldehyde undergoes a reversible reaction with water to form methanediol. Albumin in the mucus that lines the human nasal epithelium (Figure 3-1) forms an additional barrier to the systemic absorption of formaldehyde (Bogdanffy et al. 1987). The solubility of formaldehyde in mucus and the ciliary movement and ingestion of mucus may account for the removal of as much as 42% of the inhaled dose in rodents (Schlosser 1999). Diffusion is the dominant transport mechanism for formaldehyde through the mucus layer. Some inhaled formaldehyde passes through the mucus layer to reach the epithelium where its transformation and removal occur by enzymatic reactions with the nasal tissue and nonenzymatic reactions with glutathione and macromolecules, including proteins and DNA.
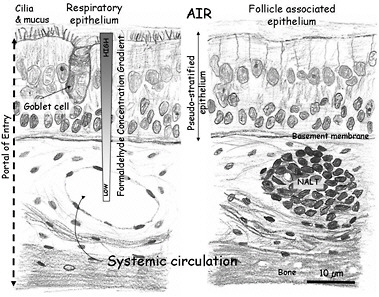
FIGURE 3-1 Schematic representation of the mammalian nasal epithelium. Inhaled formaldehyde reacts rapidly with macromolecules in the tissue and the albumin in the mucus that lines the respiratory epithelium; these reactions result in a steep concentration gradient. Formaldehyde crossing the basement membrane can react further with macromolecules in the submucosal layer or reach the systemic circulation. The figure also shows a representation of the nasal-associated lymphoid tissue (NALT) that is generally present near the ethmoid turbinates on either side of the nasal septum and near the ventral nasopharyngeal duct. The NALT is one putative site of formaldehyde interactions with lymphoid tissues, but direct evidence that supports this hypothesis is lacking.
Removal of formaldehyde from the air by the upper respiratory tract is efficient. For example, nasal uptake of inhaled formaldehyde in dogs is nearly complete (Egle 1972). Penetration of formaldehyde to more distal airways was not observed in formaldehyde-exposed dogs even in the presence of increased breathing rates or exposure to formaldehyde at high concentrations (Egle 1972). In rats and mice, which are obligate nasal breathers with a highly complex nasal-airway geometry and large ratio of surface area to lumen volume, most inhaled formaldehyde (average, about 97%) is also taken up in the nasal cavity (Patterson et al. 1986). Computer models predict that systemic delivery of formaldehyde in rodents is extremely low and would not increase formaldehyde concentrations in tissues (Franks 2005). The results of those simulations are supported by animal studies that show that formaldehyde inhalation in rodents or nonhuman primates did not alter blood formaldehyde concentrations. Studies using DPX formation as an exposure surrogate corroborate that conclusion. For example, Casanova-Schmitz et al. (1984) found that exposure of rats to 14C- and 3H-labeled formaldehyde at 15 ppm did not result in DPX formation in bone marrow. However, they did observe non-linear DPX formation in the nasal mucosa at concentrations of 2 ppm or greater.
In humans, oral breathing bypasses the uptake in the nasal epithelium; this breathing pattern leads to increased delivery of formaldehyde to the intermediate regions of the respiratory tract (that is, from the oral cavity to the upper conducting airways of the lungs) (Overton et al. 2001). Nasal uptake of formaldehyde is also reduced as ventilation rates increase.
The committee concludes that the immediate fate of inhaled formaldehyde is primarily absorption at the site of first contact where it undergoes extensive local metabolism and reactions with macromolecules, despite species differences in uptake resulting from different breathing patterns (for example, oronasal breathing in humans vs nasal breathing in rodents). Although the site of absorption in the respiratory tract may depend on airway anatomy (simple vs complex), breathing pattern (nasal vs oronasal), and ventilation rate, the net result is that inhaled formaldehyde predominantly remains in the respiratory epithelium.
Is Inhaled Formaldehyde Available Systemically?
As mentioned earlier, several studies have demonstrated that inhaled formaldehyde has at most little systemic bioavailability. Some experiments examined the disposition of 14C-labeled formaldehyde, and others used techniques of analytic chemistry—such as gas or liquid chromatography coupled to mass spectrometry (for example, GC-MS)—to evaluate changes in formaldehyde concentrations in blood or tissue after inhalation. The studies have found that formaldehyde undergoes rapid elimination in blood with virtually no increase in “free” formaldehyde in blood or systemic tissues. Although the studies demonstrate
relatively long retention of the 14C radiolabel, this observation most likely reflects incorporation and turn over of the so-called 1-carbon pool. As noted by EPA, “measuring the distribution of the absorbed formaldehyde based on 14C-radiolabeling and GC-MS studies alone is problematic because it is difficult to resolve (through these studies) whether it is free, reversibly bound, irreversibly bound, formate, one-carbon pool, etc. This is of significance with regard to understanding the availability of the absorbed formaldehyde” (EPA 2010, p. 3-12). The committee shares EPA’s concern.
A series of studies using dual-labeled (14C/3H) formaldehyde in rats has been performed to address the analytic concern (Casanova-Schmitz and Heck 1983; Casanova-Schmitz et al. 1984). The draft IRIS assessment accurately summarizes the main conclusions reached from those experiments, namely that “labeling in the nasal mucosa was due to both covalent binding and metabolic incorporation,” that “DPX [were] formed at 2 ppm or greater in the respiratory mucosa,” and that “formaldehyde did not bind covalently to bone marrow macromolecules at any exposure concentration” (up to 15 ppm) (EPA 2010, p. 3-12). The labeling of bone marrow macromolecules was found by the investigators to be due entirely to metabolic incorporation of the radiolabels, not to direct covalent binding of intact formaldehyde. The committee views those findings as supporting the hypothesis that inhaled formaldehyde is not delivered systemically under the exposure conditions used in the studies (0.3-15.0 ppm, 6 hr) (EPA 2010).
The committee also found that the more contemporary work performed by Lu et al. (2010) that examined formaldehyde-induced DNA adducts and DDX cross-links provided no direct evidence of systemic availability of inhaled formaldehyde. The Lu et al. (2010) study used 13CD2-labeled formaldehyde and showed that 13CD2-formaldehyde-DNA adducts and DDX were confined to the nasal cavity of exposed F344 rats, even though they examined much more DNA isolated from bone marrow, lymphocytes, and other tissues at distant sites for the adducts. The male Fischer 344 rats were exposed to [13CD2]-formaldehyde at 10 ppm for 1 or 5 days (6 hr/day) with a single nose-only unit.
The strongest data cited by EPA in support of systemic delivery of inhaled formaldehyde come from several studies in which antibodies to formaldehyde-hemoglobin and formaldehyde-albumin adducts were detected in blood from exposed workers, smokers, and laboratory animals. The studies did not definitively demonstrate, however, whether adduct formation occurs at a site distant from the portal of entry. For example, it is not known whether the adducts could be formed in the airway submucosal capillary beds or reflect systemic delivery of formaldehyde. Moreover, the draft IRIS assessment does not evaluate the antibody work as critically as the direct chemical-analysis approaches. The committee found that the draft does not offer a sufficient basis for EPA’s reliance on the antibody data to support the hypothesis that formaldehyde (or its hydrated form, methanediol) may reach sites distal to the portal of entry and produce effects at those sites.
Questions have arisen regarding the possibility that formaldehyde reaches distal sites as methanediol. However, although equilibrium dynamics indicate that methanediol would constitute more than 99.9% of the total free and hydrated formaldehyde, the experimental data described above provide compelling evidence that hydration of formaldehyde to methanediol does not enhance delivery of formaldehyde beyond the portal of entry to distal tissues. Furthermore, Georgieva et al. (2003) used a pharmacokinetic modeling approach that explicitly accounted for the competing processes of hydration, dehydration, diffusion, reactivity with macromolecules, and metabolism and demonstrated that hydration-dehydration reaction rates determined from equilibrium studies in water are not applicable in biologic tissues, given that their use in the model resulted in simulations that were inconsistent with the available data. For example, the calculated dehydration rate from equilibrium dynamics studies in water was so small relative to other competing rates that too little formaldehyde would be available to account for the measured DPX rates. Thus, the data provide a strong indication that the hydration-dehydration reaction should not be rate-limiting and can thus be ignored in modeling the disposition of inhaled formaldehyde in nasal tissues.
EPA also suggested that systemic delivery of formaldehyde-glutathione adducts and latter release of free formaldehyde may result in delivery of formaldehyde to sites distal to the respiratory tract. However, experimental data supporting that hypothesis are lacking, as acknowledged by the draft IRIS assessment. In fact, additional data based on even more sensitive analytic methods published since the draft assessment was released casts further doubt on the hypothesis that formaldehyde reaches the systemic distribution in a form that can react with macromolecules in tissues remote from the portal of entry (Lu et al. 2011; Moeller et al. 2011; Swenberg et al. 2011).
The committee also found two divergent statements regarding systemic delivery of formaldehyde in the draft IRIS assessment. Some parts of the draft assume that the high reactivity and extensive nasal absorption of formaldehyde restrict the systemic delivery of inhaled formaldehyde to the upper respiratory tract (for example, EPA 2010, p. 4-371). Under that assumption, systemic responses—including neurotoxicity, reproductive toxicity, and leukemia—are unlikely to arise from the direct delivery of formaldehyde (or methanediol) to a distant site in the body, such as the brain, the reproductive tract, and the bone marrow. Other portions of the document presume systemic delivery of formaldehyde (or its conjugates) and use this presumption to account in part for the systemic effects (see, for example, p. 4-1, lines 16-19; p. 4-472, line 18; Section 4.5.3.1.8; and p. 6-23, line 31). The committee found the inconsistency to be troubling, and the divergent assumptions are not justified.
The committee concludes that the issue of whether inhaled formaldehyde can reach the systemic circulation is extremely important in assessing any risk of adverse outcomes at nonrespiratory sites associated with inhalation of formaldehyde. Moreover, the committee concludes that the weight of evidence suggests that it is unlikely for formaldehyde to appear in the blood as an intact molecule,
except perhaps after exposures at doses that are high enough to overwhelm the metabolic capability of the tissue at the site of entry. Thus, although many sensitive and selective investigative approaches have been used, systemic concentrations from inhaled formaldehyde are indistinguishable from endogenous background concentrations. The committee, however, notes the importance of differentiating between systemic delivery of formaldehyde and systemic effects. The possibility remains that systemic delivery of formaldehyde is not a prerequisite for some of the reported systemic effects seen after formaldehyde exposure. Those effects may result from indirect modes of action associated with local effects, especially irritation, inflammation, and stress.
Can Formaldehyde-Related Effects Alter Its Toxicokinetics?
Formaldehyde is an irritant gas in humans and animals. Rodents exposed to sufficiently high formaldehyde concentrations develop reflex bradypnea, decreased body temperature, reduced oxygen demand, and reduced nasal mucociliary clearance. For example, the minute volume decreased by 45% in rats and 75% in mice during formaldehyde inhalation at 15 ppm for 10 min compared with controls (Chang et al. 1983). The species difference may contribute to the difference between rats and mice in the incidence of nasal lesions. At identical exposures, mice were found to receive a lower effective dose at the target tissue in the nasal cavities than rats because mice have a greater reduction in minute ventilation in response to sensory irritation of the respiratory tract. The lower effective dose of mice was verified by the observation that mice had smaller increases in formaldehyde-induced cell proliferation in the nasal mucosa than rats. The findings could explain why higher formaldehyde exposure concentrations were needed to induce the same degree of toxic effects (such as nasal tumors) in mice as occurs in rats at lower exposures (Chang et al. 1983). The committee notes that the CFD models of Kimbell et al. (2001a,b) do not account for potential effects of sensory irritation on ventilation inasmuch as only two mass-transfer coefficients, one for mucus-coated and one for non-mucus-coated epithelial regions of the nose, were used in all simulations to derive uptake into nasal tissues. However, later models that account for DPX cross-links and cytotoxicity (Conolly et al. 2000, 2002, 2003, 2004; Georgieva et al. 2003) relied on animal data that were obtained at concentrations that potentially caused irritation to derive parameters associated with metabolism and reactivity; thus, the potential effect of altered ventilation was indirectly compensated for in those model simulations.
EPA also hypothesized that airway remodeling induced by formaldehyde exposure might alter formaldehyde dosimetry. For example, squamous epithelium absorbs considerably less formaldehyde than other epithelial types (Kimbell et al. 1997). Metaplasia of the anterior nasal epithelium to a squamous epithelial phenotype occurs in rats exposed repeatedly to formaldehyde at 3 ppm or higher (Kimbell et al. 1997). Although EPA identified the consequences of
metaplasia for dosimetry, the committee concludes that this issue is not particularly important at the low exposure concentrations relevant to derivation of a reference concentration (RfC).
The committee agrees with EPA’s conclusion that “certain formaldehyde-related effects have the potential to modulate its uptake and clearance” (EPA 2010, p. 3-5). Some of the effects, such as changes in mucociliary function and altered nasal epithelium, could occur in humans. However, reflex bradypnea and related modulating effects seen in rodents do not occur in phylogenetically higher animals (nonhuman primates) or humans. Thus, formaldehyde exposures at concentrations relevant for an RfC or unit risk are unlikely to alter its toxicokinetics.
Are Formaldehyde-Induced Cross-Links Useful Biomarkers of Exposure?
As noted earlier, formaldehyde readily forms DPX and DDX cross-links. A variety of analytic chemistry approaches, including radiolabeled formaldehyde tracers, have been used to evaluate formaldehyde binding to macromolecules and to differentiate binding from metabolic incorporation. For example, rats given intravenous injections of 14C-formaldehyde or 14C-formate, a metabolite of formaldehyde that contributes to the one-carbon pool, develop similar blood radioactivity profiles; this suggests that labeling of blood macromolecules is due to metabolic incorporation rather than adduct formation (Heck and Casanova 2004).
DPX formation in human white blood cells after in vitro exposure to formaldehyde (0-10 mM) for 1.5 hr rose linearly with increasing formaldehyde concentrations above 0.001 mM (Shaham et al. 1996). The kinetics of DPX formation in vivo (and in some in vitro systems) appear more complicated and reflect a balance between DPX formation and repair processes. Overall, DPX have been detected in upper and lower respiratory tracts of rodents and nonhuman primates. For example, Casanova and colleagues (Casanova et al. 1989, 1994) conducted formaldehyde inhalation studies in male F344 rats to determine DPX formation. In one study, DPX concentrations were measured from the nasal lateral meatus, medial meatus, and posterior meatus after inhalation of 14C-formaldehyde (Casanova et al. 1994). The sites were selected because they were associated with a high tumor incidence in a formaldehyde bioassay (Monticello et al. 1989, 1991). The other study measured DPX concentrations in nasal mucosal tissue taken from the entire nasal cavity (Casanova et al. 1989). Formation of DPX demonstrated a nonlinear dose-response relationship within the nasal epithelium; statistically significant increases occurred after exposure at concentrations of 0.3 ppm and higher.
Although the mechanisms for DPX-induced cell death have not been established, DPX formation has been used as a key event linking external exposures with cellular responses (mutagenicity, cytotoxicity, and compensatory cellular regeneration) in the development of pharmacokinetic and BBDR mod-
els. Pharmacokinetic models that describe in vivo DPX formation have included saturable pathways to describe enzymatic formaldehyde metabolism, a first-order pathway to represent formaldehyde's reaction with tissue constituents, and first-order binding to DNA (Hubal et al. 1997; Conolly et al. 2000). Yang and co-workers (2010) have suggested that a single, saturable pathway competing with DNA binding could describe the DPX data fairly well in rodents exposed to formaldehyde at 15 ppm or less. The models predict that less than 1% is covalently bound as DPX in the airway (Casanova et al. 1991; Heck and Casanova 1994).
DPX have also been reported in circulating lymphocytes from formaldehyde-exposed people (Shaham et al. 1996, 1997, 2003). For example, Shaham et al. (1996) measured DPX concentrations in human white blood cells from 12 workers exposed to formaldehyde and from eight controls. The authors found a statistically significant difference in the DPX concentrations in lymphocytes between the formaldehyde workers and the unexposed controls. However, only four of the 12 exposed workers had DPX concentrations above the upper range of the controls. The authors also reported a linear relationship between years of exposure and the amount of DPX and concluded that DPX may be used as a biomarker of formaldehyde exposure. Several limitations of the study have been identified by others (Casanova et al. 1996). Most notably, the control group’s exposure history, including smoking prevalence, was poorly defined. Shaham et al. (1996) presented minimal exposure-assessment data, so the study was not used quantitatively by EPA. The committee agrees with EPA’s decision.
The concentrations of DPX formed by formaldehyde in nasal airways have been modeled and used by EPA as an internal dose surrogate to update its health assessment of formaldehyde (EPA 1991; Hernandez et al. 1994). On the basis of different model assumptions that are discussed in more detail later in this chapter, EPA changed the values of the parameters in the DPX model (Subramaniam et al. 2007), which was used to derive internal dose-related points of departure for human extrapolation. On the basis of the evidence, the draft IRIS assessment states that “DNA protein cross-links (DPXs) formed by formaldehyde (covalently bound in this case) have been regarded as a surrogate dose metric for the intracellular concentration of formaldehyde [Casanova et al. 1989, 1991; Hernandez et al. 1994]. This is particularly relevant because of the nonlinear dose response for DPX formation due to saturation of enzymatic defenses at high concentrations [Casanova et al. 1989, 1991]. Thus, the ability to measure DPX is an important development” (EPA 2010, p. 3-12). The committee agrees with the use of DPX as a biomarker of exposure in the draft IRIS assessment.
Can Inhaled Formaldehyde Have Systemic Genotoxic Effects?
Formaldehyde is a genotoxic (DNA-reactive) chemical. Formaldehyde-induced DNA damage is postulated to lead to mutations and clastogenesis, critical cytogenetic events in the carcinogenic mode of action. The evidence of for-
maldehyde genotoxicity includes DPX cross-links, chromosomal aberrations, micronuclei, and sister-chromatid exchanges. A large number of in vitro tests for genotoxicity—including bacterial mutation, DNA strand breaks, chromosomal aberrations, and sister-chromatid exchange assays—are positive when formaldehyde is used. The studies on genotoxicity of formaldehyde, particularly those involving in vivo exposures of humans and animals, have provided strong evidence that formaldehyde genotoxicity occurs in the nasal mucosa and peripheral (circulating) blood lymphocytes. The database on genotoxicity assessment using peripheral blood lymphocytes from exposed human cohorts is appreciable and contains studies from different countries and various exposure scenarios and spans a period of more than 20 years. Some more recent studies (Costa et al. 2008) provide evidence of clastogenicity of formaldehyde coupled with individual exposure assessment. Although studies in humans showed some inconsistent results regarding the extent and form of the cytogenetic changes associated with formaldehyde exposure, the overall body of evidence suggests that inhaled formaldehyde has an effect that may be detected in blood cells in the systemic circulation. The committee notes that it is unknown whether formaldehyde genotoxicity arises from interactions that occur at the site of contact—for example, in nasal-associated lymphoid tissue in the nasal mucosa (Figure 3-1)—or as the result of local circulation of lymphocytes in blood that perfuses portal-of-entry tissues.
Overall, the draft IRIS assessment concludes that formaldehyde may act through a mutagenic mode of action and that this effect is not restricted to the site of entry (EPA 2010). As noted earlier, multiple lines of evidence support the conclusion that formaldehyde is mutagenic. The committee agrees with EPA and further notes that EPA’s conclusion is consistent with the current Guidelines for Carcinogen Risk Assessment (EPA 2005).
The committee acknowledges that the database on the cytogenetic effects of formaldehyde in humans is supportive of EPA’s second conclusion, that the mutagenic action of formaldehyde is not restricted to tissues at the point of contact. However, available data are insufficient to support definitive conclusions on several key issues. First, exposure assessment in the relevant human studies was generally lacking, and the effects observed occurred in highly exposed workers. In the absence of understanding of the shape of the dose-response curve for cytogenetic changes at low doses, it is difficult to extrapolate the findings to environmental exposures. Second, the mechanism of cytogenetic effects in circulating blood cells is not established—an uncertainty that complicates the committee’s ability to link exposure with effects at distant sites. That data gap is especially problematic given the growing body of evidence that formaldehyde is not available systemically in any reactive form. Thus, it can only be hypothesized that systemic effects, such as cytogenetic effects in circulating blood lymphocytes, originate by as yet unproven mechanisms in portal-of-entry tissues.
The committee concludes that there is great uncertainty and associated controversy regarding the following issues: the ability of formaldehyde to cause DNA damage at distal (that is, other than portal-of-entry) sites, the relative con-
tributions of DNA adducts vs cross-links to carcinogenesis, the ability of formaldehyde exposures at ambient concentrations to increase the burden of endogenous formaldehyde-induced DNA damage that can contribute to carcinogenesis at local or distant sites, and the consistency of the database on formaldehyde-induced genotoxicity among species.
Are Useful Computational Pharmacokinetic Models for Formaldehyde Inhalation Available?
EPA’s Guidelines for Carcinogen Risk Assessment state that “toxicokinetic modeling is the preferred approach for estimating dose metrics from exposure. Toxicokinetic models generally describe the relationship between exposure and measures of internal dose over time. More complex models can reflect sources of intrinsic variation, such as polymorphisms in metabolism and clearance rates. When a robust model is not available, or when the purpose of the assessment does not warrant developing a model, simpler approaches may be used” (EPA 2005, p. 3-1). Anatomically based three-dimensional (3D) computational-fluid-dynamics (CFD) models of rat, monkey, and human nasal passages have been developed to predict interspecies nasal dosimetry of inhaled formaldehyde (Table 3-1). Mass-transfer coefficients calibrated against total nasal uptake were used as boundary conditions in CFD models to determine site-specific formaldehyde flux rates (Kimbell et al. 1993, 2001a,b). As is consistent with experimental data, regional flux rates predicted by the models depend on airflow characteristics, exposure concentration, and the absorption properties of the nasal lining.
CFD model estimates of nasal uptake can range from about 60% to more than 90% of inhaled formaldehyde depending on exposure conditions and species. Reduced nasal uptake does occur at high air concentrations, but this would not be predicted with the current CFD model (Kimbell et al. 1993, 2001a,b), because it did not include a saturable mechanism for tissue metabolism of formaldehyde in the mass-transfer boundary condition. In humans, about 90% absorption of inhaled formaldehyde is predicted to occur in the nose on the basis of a single CFD model with pharmacokinetic parameters scaled from animals at resting ventilation; this estimate decreases to about 60% with light exercise and 55% with heavy exercise (Kimbell et al. 2001b).
CFD models were combined with biologically based pharmacokinetic (PK) models to describe site-specific and regional uptake of formaldehyde along conducting airways of rats and monkeys at sites that correspond to areas that have measured DPX, cell proliferation, pathology, or tumor formation (Casanova et al. 1991; Kimbell et al. 1993; Hubal et al. 1997; Conolly et al. 2000; Kimbell et al. 2001a,b). Some models incorporate the flux of formaldehyde into cells of nasal passages as the model input (Hubal et al. 1997; Conolly et al. 2000). The most advanced models provide an estimate of cellular formaldehyde
TABLE 3-1 Analysis of 3D CFD Models by Kimbell et al. (2001a,b) and Overton et al. (2001) for Rat, Monkey, and Human Airways
Component |
Model Strengths |
Model Weaknesses |
CFD model design |
|
|
Site-specific fluxes of formaldehyde |
|
|
Component |
Model Strengths |
Model Weaknesses |
|
|
|
Human respiratory tract |
|
|
CFD-PK models |
|
|
Abbreviations: CFD, computational fluid dynamics; PK, pharmacokinetic; DPX, DNA-protein crosslinks; BBDR, biologically based dose-response; and ADH3, alcohol dehydrogenases. |
concentrations based on CFD model predictions of flux, estimated epithelial surface areas, assumed tissue thicknesses in low-tumor and high-tumor regions, and the rate of formaldehyde reaction with cellular constituents via a saturable enzyme-mediated metabolism, a nonenzymatic first-order reaction with cellular macromolecules, or a first-order reaction with DNA to form DPX. Once formed, DPX were eliminated actively or by normal degradation at a constant rate. The models also predict a steep, decreasing concentration gradient between the mucus layer and each successive layer of cells from the airway to the vasculature as a result of formaldehyde reaction with extracellular and cellular glutathione, proteins, other macromolecules, and metabolizing enzymes. The draft IRIS assessment provides a comprehensive overview of the CFD PK models that are available for formaldehyde.
The draft IRIS assessment raises the criticism that the nasal CFD models are based on a single geometry for each species. Thus, the models do not address variability that arises from differences in airway anatomy. A recent paper by Garcia et al. (2009) evaluated the effect of individual differences in airway geometry on airflow and uptake of reactive gases, such as formaldehyde. Although the sample was small (five adults and two children), the individual differences in airway geometry alone caused the potential flux rates to vary by a factor of only 1.6 over the entire nose and by a factor of 3-5 at various distances along the septal axis of the nose. The committee agrees with EPA that although the sample was small, the estimates of individual variability are consistent with default uncertainty factors applied to internal dose metrics that account for human variability. Another EPA criticism is that idealized geometries (cylinders) rather than real geometries are used to represent the human larynx, trachea, and lung with no evaluation of potential variability associated with actual airway geometry.
EPA also raised a concern that high formaldehyde concentrations (3 ppm or higher) can reduce minute volumes, alter mucus flow, or change absorption by tissue remodeling and that the existing models capture these effects inconsistently. The committee notes that mass-transfer coefficients in the CFD models are used to capture the effects of exposure at irritating concentrations (15 ppm) at which respiratory depression occurs (Kimbell et al. 2001a,b); this allows the models to describe the experimental data adequately at high exposure concentrations. However, the committee also notes that the mass-transfer coefficients used in the human models were optimized on the basis of the rat models.
Despite the concerns raised, EPA used the CFD models to derive human-equivalent concentrations (HECs) on the basis of formaldehyde flux rates. The CFD-based flux rates over the entire nose of the rat (excluding the vestibule and olfactory region) were used to calculate the HEC by multiplying the average flux for the rat at a no-observed-adverse-effect level by a dose-duration adjustment—(5/7)(6/24)—to represent continuous exposures. EPA also calculated benchmark concentrations by using flux rate as the internal dose in dose-response assessments. The models were used to evaluate whether the lower airways in the human are potential targets for formaldehyde toxicity and carcino-
genicity. To do that, EPA used the one-dimensional human-airway model of Overton et al. (2001) to determine the risk of lower respiratory airway tumors, assuming equal sensitivity of cells in this region to equivalent formaldehyde fluxes determined for the nose. EPA restricted application of the models to the experimental range used in animal studies to determine an internal dose-based point of departure for humans but did not use them to extrapolate to low exposures.
The committee disagrees with EPA’s findings that CFD models are not useful for low-dose extrapolations. In fact, flux results from the CFD models can easily be scaled from an exposure of 1 ppm—as given by Kimbell et al. (2001a,b) and Overton et al. (2001)—to lower concentrations because of the linear flux-concentration relationship that was used by the authors. Therefore, the committee recommends that the CFD-based approach also be used to extrapolate to low concentrations, that the results be included in the overall evaluation, and that EPA explain clearly its use of CFD modeling approaches.
The committee concludes that sufficiently robust pharmacokinetic models for formaldehyde exist and agrees with EPA that the CFD models can and should be used in the IRIS assessment. Furthermore, it finds that the CFD models were fairly evaluated and that the sources of uncertainty in dose metrics used in dose-response assessments were appropriately treated.
CARCINOGENESIS: HAS A MODE OF ACTION OF FORMALDEHYDE BEEN IDENTIFIED?
Mode of action is defined by EPA as a sequence of key events and processes, starting with the interaction of an agent with a cell and continuing through operational and anatomic changes that result in an adverse outcome (EPA 2005). A key event is an empirically observable precursor step that is itself a necessary element of the mode of action or is a biologically based marker of such an element (Boobis et al. 2009). Knowledge of the mode of action can inform the risk-assessment process. Indeed, mode of action in the assessment of potential carcinogens is a main focus of EPA’s cancer guidelines (EPA 2005). In the absence of sufficient, scientifically justifiable information on mode of action, EPA generally takes public-health-protective, default positions regarding the interpretation of toxicologic and epidemiologic data.
The draft IRIS assessment provides an exhaustive summary of the studies on genotoxicity of formaldehyde. The literature review appears to be up to date and includes all major and recent studies. The relevant chapter is well organized by type of DNA damage and then by evidence of clastogenicity from in vitro and in vivo sources. More weight is placed on studies in human cells and in exposed human cohorts, however small each study may be. Data are presented in informative and well-organized tables that provide a high-level summary of the extensive database on each subject. The summary statement and the entire chapter are well balanced and include both positive and negative studies. The conclu-
sion that formaldehyde is genotoxic and mutagenic in model systems and in mammals, including humans, is supported by the data and is in accordance with the weight of evidence required by EPA’s cancer guidelines (EPA 2005).
Cytotoxicity and compensatory cell proliferation also appear to play important roles in the carcinogenic mode of action of formaldehyde-induced nasal tumors. Substantial nonlinearity in dose-response relationships was observed in animal studies of DPX cross-links, cytotoxicity, and compensatory cell proliferation (Swenberg et al. 1983; Monticello et al. 1996). There is a strong site concordance between formaldehyde uptake, cytotoxicity, cell proliferation, and tumor formation. Furthermore, no tumors were observed at concentrations that did not also cause cytotoxicity. The draft IRIS assessment discusses this alternative mode of action but relies on the mutagenic mode of action to justify low-dose linear extrapolations in the assessment of formaldehyde-induced nasal tumors.
In the case of hematopoietic cancers, particularly leukemia, much less is known about potential modes of action other than mutagenicity, which has been demonstrated in vitro and in a few studies of occupationally exposed humans. Although EPA postulated that formaldehyde could reach the bone marrow either as methanediol or as a byproduct of nonenzymatic reactions with glutathione, numerous studies described above have demonstrated that systemic delivery of formaldehyde is highly unlikely at concentrations below those which overwhelm metabolism according to sensitive and selective analytic methods that can differentiate endogenous from exogenous exposures. As a result, EPA could only speculate that circulating hematopoietic stem cells that percolate through nasal capillary beds or nasal-associated lymphoid tissues may be the target cells for mutations and clastogenic effects that eventually result in lymphohematopoietic cancers. Experimental evidence of either mechanism is lacking.
Although EPA followed its guidelines for assessing the risk of cancer associated with a mutagenic mode of action, it acknowledged that major uncertainties and controversy remain regarding application of linear models for low-dose extrapolations for a chemical that is formed endogenously and is too reactive to be measured in the body apart from portal-of-entry tissues. As discussed in the following section on BBDR modeling, the committee recommends that, for transparency and completeness, EPA consider providing alternative calculations that factor in nonlinearity associated with the cytotoxicity-compensatory cell-proliferation mode of action and assess the strengths and weaknesses of each approach.
The committee concludes that two primary modes of action have been observed to contribute to formaldehyde-induced carcinogenicity in nasal tissues: mutagenicity and cytotoxicity with compensatory cell proliferation. There is no doubt that formaldehyde is a DNA-reactive chemical that produces DNA adducts (DPX cross-links and DDX cross-links) that, if not repaired, can lead to mutations and clastogenesis.
USE OF A BIOLOGICALLY BASED DOSE-RESPONSE MODEL
EPA’s Guidelines for Carcinogen Risk Assessment state that “in the absence of sufficient data or understanding to develop…a robust, biologically based model, an appropriate policy choice is to have a single preferred curve-fitting model for each type of data set” (EPA 2005, p. 1-10). The guidelines acknowledge that many curve-fitting models have been developed and that the ones that fit the observed data reasonably well may lead to wide differences in estimated risk at the lower end of the observed range. The guidelines also recognize that several competing models could be developed and further state that “if critical analysis of agent-specific information is consistent with one or more biologically based models as well as with the default option, the alternative models and the default option are both carried through the assessment and characterized for the risk manager. In this case, the default model not only fits the data, but also serves as a benchmark for comparison with other analyses” (EPA 2005, p. 1-9). The committee notes that the use of default and alternative models for formaldehyde risk assessment remains controversial.
What Is the Status of Biologically Based Dose Response Models for Formaldehyde?
A series of papers described the development of a biologically based model for formaldehyde in rats (Conolly et al. 2003) and humans (Conolly et al. 2004). A consistent focus of formaldehyde modeling has been the low-dose linear formation of DPX as a key component in the mode of action of cytotoxicity and carcinogenesis (Conolly et al. 2000). The model improves on previous CFD-derived estimates of nasal-airway flux and DPX formation in the anterior portion of the rat nose (Hubal et al. 1997) by using a more complete version of the rat-nose and nasal-airway models for the monkey and human as described by Kimbell et al. (2001a,b) and Overton et al. (2001). The whole-nose DPX data were supplemented with regional-DPX data on the F344 rat (Casanova et al. 1994) and rhesus monkey (Casanova et al. 1991) to correspond with areas of high and low tumor incidence. The key linkage (internal dose metric) between exposure and DPX is the concentration of formaldehyde in nasal tissue. The local tissue concentration is determined from CFD-derived formaldehyde flux rates, the thickness of the epithelium, and saturable metabolism and first-order clearance from the tissue. DPX concentrations are calculated by assuming first-order rates of binding to DNA and first-order rates of repair of DPX (thus, the low-dose linear relationship with exposure and higher than linear increase in DPX at concentrations above saturable metabolism by alcohol dehydrogenases). The Conolly et al. (2003, 2004) models can be used to predict the location and magnitude of cell proliferation in respiratory tissues in response to formaldehyde exposure conditions (concentration and duration) and respiratory rates (resting or working conditions).
Conolly and colleagues (2003) used rat data on both formaldehyde-induced DPX and cytotoxicity-compensatory cell proliferation as modes of action to link regional dosimetry with rat tumor responses. Dose-dependent tumor responses were predicted by using the Moolgavkar-Venzon-Knudsen (MVK) two-stage clonal-growth model of cancer (Conolly et al. 2003), in which DPX formation was assumed to increase the probability of mutations that lead to squamous-cell carcinoma in rat nasal tissue. The clonal-growth model includes mutation rates (mutagenic mode of action) of normal and intermediate cells and rates of birth and death of normal and intermediate cells (cytotoxicity-compensatory cell proliferation) that lead to the formation of malignant cells and, after a delay, tumor responses. The clonal-growth model was calibrated against data collected from a 2-year pathogenesis bioassay (Monticello et al. 1996) and extrapolated to humans to predict additional cancer risk associated with continuous environmental exposure to formaldehyde (Conolly et al. 2004).
The assumptions used by Conolly et al. (2003, 2004) to develop their models were extensively reviewed and evaluated by EPA for their potential effect on model predictions in the draft IRIS assessment (Table 3-2). For example, the only structural difference between the human model and the rat model is that the human model includes the one-dimensional full-respiratory-tract model of Overton et al. (2001) to determine flux rates as the dose metric. Rather than a focus only on predicting nasal tumors, the human model was used to predict the risk of all human respiratory tract tumors. The human-DPX model was scaled up from the rat and monkey models on the basis of allometric relationships of physiology and metabolism to body weight. For the human two-stage clonal-growth model, baseline mutation rates were calibrated against human lung-cancer incidence data, and formaldehyde-specific parameters associated with mutation rates and growth advantages of intermediate cells were assumed to be the same as those developed for the rat model. Although Conolly et al. (2003) clearly noted the need for additional research to address uncertainties and variability issues associated with this model (as is the case with any model), they believed that they made conservative choices—use of the hockey-stick model for cell proliferation rather than the best-fit J-shaped model and steady-state inhalation formaldehyde flux rates and oronasal breathing under various working conditions as the internal dose driver rather than realistic cyclic breathing rates.
A major strength of the human model is that its developers were consistently clear about the model structures, the assumptions used to simplify an otherwise complicated process, and how the model parameters were measured, fixed on the basis of relevant data or known system constraints, or estimated from in vivo data. The developers were also open about sources of variability and uncertainty in their models and further demonstrated the utility of a unifying model to help to identify aspects that have the most influence on predictions. Thus, the model is “transparent” (if technically challenging) and available for
TABLE 3-2 Overview of the Conolly et al. BBDR Models
Model Component |
Model Assumptions |
Potential Effect |
Possible Model Refinements |
Parameters associated with saturable metabolism, first-order clearance, and first-order DNA binding |
Parameters were all optimized from the regional DPX concentration data in rats and monkeys rather than independently determined and then verified against the DPX data. Estimates of the first-order loss of DPX (repair) were arbitrarily set to the lowest value that ensured complete clearance of DPX in 18 hr for the highest exposure (that is, time from end of one 6-hr exposure at 15 ppm to start of next 6-hr exposure). |
The first-order rate constant for DNA binding accounts for a small fraction of formaldehyde tissue clearance; thus, uncertainty is associated with estimates of saturable metabolism and the first-order clearance process that dominate the disposition of formaldehyde in respiratory tissues. |
Additional experimental data would be needed to independently develop and evaluate the key process associated with formaldehyde disposition in nasal tissues. As such data are developed, fewer parameters would need to be optimized, and overall uncertainty in modeling tissue dose would be reduced. |
Nasal blood flow |
Blood flow in the nasal submucosa was not considered in the development of the BBDR models because existing data showed that no detectable increase in blood formaldehyde occurs after inhalation exposures. |
The presence of albumin and hemoglobin adducts suggests that formaldehyde can penetrate into the blood perfusing the nasal submucosa (although formaldehyde’s reactivity has precluded its penetration and detection in systemic blood). Thus, local blood flow could have an effect on the optimization of metabolism and clearance rate constants; this is a testable hypothesis. |
Explicitly define regional nasal blood flow in the model and determine its sensitivity to current parameter estimates. |
|
Species-specific blood-flow data are sparse; a wide range of flows (0.1-1% of cardiac output) have been used in other models. |
Tissue thickness |
Use of measured tissue-thickness averages for the monkey gave visually poor fits to the DPX data during optimization of the clearance parameters. |
Directed Monte Carlo approach was used to optimize tissue thicknesses to values within one standard deviation of the measured mean; this finding indicated that the parameters associated with the clearance and binding of formaldehyde are sensitive to the estimates of tissue thickness. |
Better estimates of actual tissue thickness (and better dissection techniques for DPX measurements) would mean that this variable would not have to be optimized and would improve confidence in the other optimized parameters. |
Flux bins |
Nasal flux bins represent percentage of nasal surface areas that achieve a particular formaldehyde flux that fall within 20 equally divided intervals of flux ranging from 0 to the maximum rate on the airway surface; thus, no geometric location or site specificity is implied. |
Flux bins are based on 20 equally spaced distributions of formaldehyde flux rates and not representative of specific locations in the nose. Thus, site-specific flux rates are not matched to site-specific DPX measurements to derive estimates of metabolism, binding, and clearance processes in the nose. |
Better definitions of CFD-model boundary conditions—including linkage to PBPK models for localized tissue concentrations in mucus, epithelium, and submucosa similar to that in models already developed for other reactive gases, such as acrolein and hydrogen sulfide—would improve the optimization of metabolism, binding, and clearance parameters and reduce the uncertainty by improving the correlation between regional dosimetry and DPX binding data. |
|
|
The 25 flux bins for the rest of the respiratory tract used in this model are associated with anatomic regions rather than predicted flux ranges. |
|
Two statistical models were evaluated that relate time- and site-averaged cell proliferation data to formaldehyde exposure |
J-shaped model provided the best fit to the data. |
The J-shaped model implies that at low formaldehyde exposure concentrations, cell proliferation is less than control levels. Although that model provided a better description of the data, its application in the BBDR models would result in |
None; both models (J-shaped and hockey-stick) should be carried through the simulations as was done by Conolly et al. The simulations should be compared with the EPA default no-threshold low-dose extrapolation assumption, and the results clearly |
Hockey-stick model was considered more conservative because it removed the low-dose decrease in cell proliferation and |
Model Component |
Model Assumptions |
Potential Effect |
Possible Model Refinements |
|
replaced it with a threshold that was fixed at the lowest concentration that produced a measurable increase in cell proliferation. |
fewer tumors predicted at low exposures than predicted in controls. Thus, the hockey-stick model, which implies a threshold for tumors, was also carried forward in the BBDR models. |
compared and evaluated as to the relative strengths, weaknesses, and effects of each approach. |
Formaldehyde flux rates derived from the CFD models used to provide input into the BBDR models for rats and humans |
Same assumptions, strengths, and weaknesses of the CFD models apply to the Conolly et al. models (see Table 3-1) |
Flux rates were based partly on two mass-transfer coefficients (one for mucus-coated and one for nonmucus-coated epithelium) that were estimated from data on nasal extraction of inhaled formaldehyde in rats. The mass-transfer coefficients were assumed to be constant across ventilation rate, formaldehyde concentration, region of the nose, and species. Because regional and species differences in metabolism would be expected, site-specific flux determinations used as inputs into the BBDR models would also be expected to be affected by the assumed mass-transfer coefficients. |
See Table 3-1 and previous refinements in which direct coupling of PK models as boundary conditions on the CFD model would decrease the uncertainties associated with site-specific estimates of metabolism, binding, and clearance parameters that affect the inputs into the BBDR models. |
Abbreviations: DPX, DNA-protein crosslinks; BBDR, biologically based dose-response; CFD, computational fluid dynamics; PK, pharmacokinetic; PBPK, physiologically based pharmacokinetic; and EPA, Environmental Protection Agency. |
evaluation and testing of alternative approaches (as was done by EPA). The committee examined key assumptions associated with the Conolly et al. models and estimated the likely effect of changes in the assumptions on model output (Table 3-3).
EPA agreed that the Conolly et al. (2003) model provided a better fit to the time-to-tumor data in the animal bioassays than other models that EPA could use in a cancer risk assessment. EPA also expressed confidence in the dosimetry modeling for flux rates and DPX formation in the rat and monkey, although it expressed concern that one model is used as representative of a population and another is an idealized model for the human lung.
EPA largely disagreed with many of the other conclusions presented in Conolly et al. (2003). It did not agree with the interpretation that DPX formation (and therefore mutagenicity) at low doses was negligible and did not contribute to an increase in nasal cancer risk. The committee notes that although Conolly and co-workers (2003, 2004) assumed that cytotoxicity-compensatory cell proliferation was the dominant mode of action in predicted tumor responses, they were careful to use an upper bound on values of rat parameters to force the model to calculate additional risk due to other mechanisms, such as mutagenicity.
EPA conducted a reanalysis of the Conolly et al. models, which was reported in a series of papers published in 2005-2010 (Crump et al. 2005, 2008, 2010; Subramaniam et al. 2007, 2008), and explored the uncertainties and sensitivities of the dynamic response components of the Conolly et al. formaldehyde models to changes in several key model parameters and assumptions. EPA’s reanalysis was consistent with its cancer guidelines that specify that the uncertainties and variability in model parameters must be understood and articulated so that predictions of adverse responses and extrapolations to human exposures can be appropriately characterized from the standpoint of human health protection (EPA 2005). Thus, EPA’s reanalysis focused on several key components in the Conolly et al. models that can have substantial effects on predicted human tumor formation and low-dose extrapolations when the models are used below the range of experimental observations.
EPA’s analysis (EPA 2010) evaluated the following:
-
Choice of background nasal-tumor incidence data in rats and total respiratory-tumor incidences in humans, which were used to define basal mutation rates for normal and intermediate cells.
-
Choices of model structure and associated parameters used to describe DPX-formation data and cell-proliferation data collected in rats and monkeys as a function of time and exposure (that is, linear vs J-shaped vs hockey-stick-shaped dose-response curves).
TABLE 3-3 Effects of Different Parameters on Predicted Results of the Conolly et al. BBDR Models
Parameter Assumptions |
Likely Effect on Model |
Regenerative cell-proliferation data were directly related to cytotoxicity |
Moderate; the model results were sensitive to the model parameters used, but the assumption that regenerative cell proliferation is directly related to formaldehyde-induced cytotoxicity is reasonable. |
Site-to-site variation in cell proliferation in the rat nose does not vary in concordance with site-specific flux rates as determined by the CFD model |
Moderate; only two mass-transfer coefficients were used to drive the uptake in all areas of the nose. That ignores the potential for site-specific local differences in tissue thickness and clearance processes that affect uptake. |
Cell proliferation followed a J-shaped dose-response relationship |
Minimal; although the cell-proliferation data suggest a J-shaped dose-response relationship, both a J-shaped and a hockey-stick model were used in the BBDR models. |
Human cells were equally sensitive to the same internal dose surrogate as rats and were the basis of model parameters |
Minimal; because the relationships between cell proliferation and simulated formaldehyde flux rates were similar in rats and monkeys, it is reasonable to assume that they would be similar in humans. Subramaniam et al. (2008) suggest that in the exposures at which tumors were seen, the mutagenic mode of action contributed up to 74% of the added tumor probability, whereas Conolly et al. (2003, 2004) showed little contribution by this mode of action in the human when they used an upper bound on the DPX proportionality constant rather than the maximum likelihood estimate of zero from the rat data. |
Number of cells at risk was proportional to body weight |
Unknown; it is not known how sensitive the BBDR models are to changes in the number of cells at risk by body weight. |
DPX was a promutagenic lesion that was proportional to DPX burden |
High; the current parameter estimates that Conolly et al. (2003) optimized from the data, using a maximum likelihood function, suggest that the proportionality constant for DPX adding to the mutation rate of a normal (or intermediate) cell should be zero or close to zero. That suggests that DPX is not directly related to the key events leading to mutation and carcinogenicity per se. Because this is the only low-dose linear relationship between exposure and a biomarker of response, EPA contends that the low-dose extrapolations should be linear through zero dose. For example, Subramaniam et al. (2007) examined alternative choices to parameters associated with DPX clearance and suggested that in the exposures at which tumors were seen, the mutagenic mode of action could contribute up to 74% of the added tumor probability. Because too few parameters were experimentally fixed and too many optimized against one data set, confidence in deciding whether the Conolly et al. or the Subramaniam et al. approach is the most scientifically defensible is not high. |
|
In a follow-on analysis, Crump et al. (2008) made an arbitrary change in the DPX-based effect on initiated cell replication by theorizing that if an initiated cell is created by a specific mutation that impairs cell-cycle control, there may be a mitigation of cell replication that is observed in the low-dose cell proliferation of normal cells (that is, in the negative vs baseline replication portion of the J-shaped dose-response curve) and hence a shift of the cell division of an initiated cell in the model toward greater rates at low doses. They argued that this theoretical possibility provides biologic motivation to test the effect on the MVK model because of the absence of data for doing otherwise. The change disconnects the birth and death rates of initiated cells from constraints used by Conolly et al. based on normal cells. The committee concludes that this change is contrary to the explanation provided by Monticello et al. (1996), who suggested that it is not a mutation in cell-cycle check points that results in lower cell-division rates than control at low exposures but rather an increase in the time that it takes for DNA-repair processes to eliminate the DPX before the cell can resume the process of cell division that leads to a cell-division rate at low exposures that is below that seen under basal conditions. These are two fundamentally different mechanisms with different connotations for risk—the mutagenic one chosen by EPA and the DNA-repair mode of action supported by several other publications on DPX cited by Conolly et al. (2003, 2004) and Monticello et al. (1996). Because they argue that there are no data to refute these assumed and arbitrary adjustments of the Conolly et al. models, they state that the onus is on others to show that such small changes cannot occur (that is, prove a negative before the authors would accept the contention that the Conolly et al. models are at all conservative as Conolly et al. suggested). That standard cannot be met. |
Basal mutation rates for normal and intermediate cells are unknown and had to be optimized from sparse information using several assumptions |
High; the MVK model is very sensitive to the choice of controls and their effect on basal mutation rates. |
There were zero squamous cell carcinomas in control rats in the two bioassays used to define the basal mutation rates of normal and intermediate cells in the two-stage, MVK dose-response model. Conolly et al. (2004) used results from the full National Toxicology Program historical control database. That is a point of contention by EPA, which believes that only historical controls from inhalation bioassays (and those in the same laboratory as the formaldehyde study) can be used in a relevant comparison. Squamous cell carcinomas are so rare that some leeway in approximating basal rates may have to be accepted, even though EPA’s point is technically correct. |
|
|
When mutation rates, cell-division rates, and mutation intensity vary as a function of age (that is, not treated as constants over all ages), the Hoogenveen solution can lead to more errors than alternative models that respect the nonhomogeneity of the model parameters (Crump et al. 2005). Although EPA may be technically |
Parameter Assumptions |
Likely Effect on Model |
|
correct, it was unclear to the committee whether there is a significant effect on the Conolly et al. (2004) prediction unless their parameter values are substantially changed, which would open the Crump et al. and Subramaniam et al. models up to an equally fair criticism of their assumptions and choices in model parameters. |
|
Estimating parameters for basal mutation rates for a normal to intermediate and intermediate to malignant transformation in humans is subject to even more uncertainty than in the rat. |
|
Crump et al. (2008) made seemingly arbitrary changes in the birth and death rates of initiated cells, claiming that there are no data to suggest that they cannot do that and that their changes were small relative to changes in this relationship at high formaldehyde flux rates. Although no changes in the predicted data range were observed, the effect of the low-dose and high-dose extrapolations was huge. |
Intermediate-cell parameter values were related to normal-cell parameter values and constrained by relevant cell-proliferation and tumor data |
Moderate. The proportionality constant for relating DPX to the human cell-mutation rate (KMU) was adjusted from the upper bounds on the rat value rather than the maximum likelihood estimate of the rat value, which was zero, by a ratio of the basal mutation rate of human to rat; this assumes that the mutation mechanism is the same in control vs formaldehyde exposure and that DPX can be viewed as a promutagenic event. Those assumptions suggest that human cells are more difficult to mutate than rat cells on the basis of their evaluation of the literature, and they force the model to include clonal growth at exposures below those known to cause cell proliferation in the rat. The authors view the assumptions as conservative. |
Changes in epithelial cell thickness due to formaldehyde exposure had no effect on DPX measurements used to calibrate the BBDR models |
Moderate. The first-order clearance of DPX could be slower than that used by Conolly et al. (2003, 2004). Over time, epithelial tissue in targeted regions of the nose thickens. The thickening could conceivably dilute DPX concentrations in the measured tissues to such an extent that residual concentrations 18 hr after exposure are not different from those in naïve animal s, and this would affect the determination of DPX clearance rates. |
Abbreviations: DPX, DNA-protein crosslinks; BBDR, biologically based dose-response; CFD, computational fluid dynamics; EPA, Environmental Protection Agency; and MVK, Moolgavkar-Venzon-Knudson. |
-
Assumptions used in defining parameters associated with mutation, birth, and death rates of intermediate cells, cells that cannot be directly measured or even identified histologically (that is, intermediate cells in the MVK 2-stage model structure are a surrogate for potentially multiple stages of transformed but not yet malignant cells).
Other sources of variability and uncertainty were also explored, but the three noted were the main focus of EPA’s reanalysis. The committee agrees that the sensitivity analysis added value to the interpretation of the Conolly et al. models, although it raised questions about the degree to which manipulations of the range of model parameter values can and should be performed to reflect potentially divergent outcomes. EPA’s reanalysis also identified a flaw in one of the numeric approaches used in the original models and corrected it to improve the reliability of the simulation—another value added.
Alternative model structures fitted to the data used by Conolly et al. can yield different low-dose extrapolation results (Crump et al. 2005, 2008; Subramaniam et al. 2007, 2008). For example, Subramaniam et al. (2007) assessed the sensitivity of model-predicted tumor response to two major Conolly et al. assumptions. First, Conolly et al. pooled all National Toxicology Program historical controls to establish basal mutation rates. Second, data obtained by lumping animals found to have tumors at scheduled sacrifice with animals that died as a result of tumors were used to estimate formaldehyde-specific mutation rates. Subramaniam et al. also examined the choice for the first-order clearance of DPX used by Conolly et al. to match in vivo data with a slower clearance determined in vitro and how this could still be consistent with the in vivo data. The purpose of the Subramaniam et al. work was to explore whether the mutagenic mode of action, as exemplified by DPX as a precursor, has an insignificant role in predicted tumor responses. Their analysis indicated that in the range of exposures in which tumors occur, the mutagenic mode of action could have contributed up to 74% of the added tumor probability, whereas Conolly et al. (2003, 2004) showed very little contribution by this mode of action in the human when they used an upper bound on the DPX proportionality constant rather than the maximum-likelihood estimate of zero from the rat data. The committee agrees with EPA that existing data are insufficient to establish the potential biologic variability in model parameters associated with the mutagenic mode of action adequately. However, because the mutagenic mode of action is the major reason for adopting the default low-dose linear extrapolation methods over application of the BBDR models in the draft assessment, the committee recommends that the manipulations that lead to such high contributions of mutagenicity to the mode of action for nasal tumors be reconciled with the observations that formaldehyde is endogenous, that nasal tumors are very rare in both rats and humans, and that no increases in tumor frequency have been observed in animal studies at formaldehyde exposure concentrations that do not also cause cytotoxicity.
In a follow-on paper, Crump et al. (2008) evaluated the sensitivity of model output to varying such parameters as mutation, birth, and death rates of
initiated cells and concluded that small changes in these parameters can result in similar fits to experimental data but yield markedly different low-dose extrapolations. The authors argued that—inasmuch as there are no data that define mutation, birth, and death rates of initiated cells, let alone data that identify what an initiated cell is—the onus is on others to demonstrate that the small changes that they made in the Conolly et al. (2004) model cannot occur. However, that can never be established with certainty. Although the committee finds that testing of alternative models and the variability in model parameters is consistent with EPA’s cancer guidelines, some of the more extreme model scenarios should not have been used as a basis for rejecting the BBDR approach. In particular, adjustments of parameter values associated with mutation, birth, and death rates of initiated cells used in EPA’s analysis of alternative models that yielded the most extreme deviations from the Conolly et al. (2004) low-dose extrapolations also produced unrealistically high added risks for humans at concentrations that have been observed in the environment of occupationally exposed workers (100% incidence at concentrations as low as about 0.1-1 ppm). Thus, the committee recommends that manipulations of model parameters that yield results that are biologically implausible or inconsistent with the available data be discarded and not used as a basis for rejecting the overall model.
The committee concludes that the existing BBDR models were developed from an impressive exposure- and time-dependent database on the modes of actions of formaldehyde in rats and monkeys; exquisitely detailed dosimetry models in rats, monkeys, and humans; and sparse data on humans that required scale-up of key model parameter values from animal studies and other biologic data and epidemiologic observations to constrain the human model predictions. The scope of the research makes this one of the best-developed BBDR models to date for any chemical, even with its acknowledged uncertainties. The committee also acknowledges that the draft IRIS assessment provides a thorough review of the BBDR models, the major assumptions underpinning the extrapolation to humans, and EPA’s own series of papers that evaluated the sensitivity of the BBDR models to these assumptions even though the committee may not agree with the validity of all the resulting manipulations.
Should the Biologically Based Dose-Response Models Be Used in the Environmental Protection Agency Quantitative Assessment?
As a result of the agency's reanalysis of the models, EPA chose not to use the full rat and human BBDR models to estimate unit risks. Instead, in a benchmark-dose approach, EPA used the CFD-derived determinations of formaldehyde flux to the entire surface of mucus-coated epithelium to derive a point of departure based on nasal cancers in rats. It then extrapolated to zero dose by using a default linearized multistage approach.
The committee is concerned about that approach for low-dose extrapolation. The committee found that the evaluations of the original models and EPA’s
reanalysis conflicted with respect to the intent or purpose of using the formaldehyde BBDR models in human health assessments. The conflict is evident in the discussions in the draft IRIS assessment. For example, the reanalysis by Subramaniam et al. is used to support the mutagenic mode of action of formaldehyde and to reduce support for using the BBDR models on the basis of the uncertainties in parameter estimation and assumptions in the models. In contrast, Conolly et al. (2003) focused their model parameter estimates to represent “best-fit,” using maximum likelihood estimates, whereas Subramaniam et al. and Crump et al. pushed parameter assumptions in a single direction to show that different assumptions that fit the experimental data can yield different results of low-dose extrapolation. The committee is concerned about the possibility that those adjustments of the Conolly et al. models may not be scientifically defensible. The committee was also struck by the relative lack of transparency in the draft IRIS assessment’s description of the decision to use the peer-reviewed BBDR models minimally.
The Conolly et al. (2003, 2004) rat and human dose-response models, as the authors themselves state, are biologically motivated and mechanistic. The main purpose of a mechanistic model is to predict as accurately as possible a response to a given exposure and to provide a rational framework for extrapolations outside the range of experimental data and between species. The biologic basis of the mechanistic models implies that parameters associated with anatomy, physiology, physical and chemical properties, biochemical interactions, and dynamic responses are constrained within biologic and physical limits; they are unlike purely empirical, statistical, or mathematical models that are fit to a given dataset.
A key feature of a biologically motivated mechanistic model is that all relevant data must be reconciled with the model and that, if they cannot be, a reasonable explanation for disagreements must be articulated. Model predictions must also be reconciled with plausible outcomes, which serve as final constraints on model structure and parameter estimates. Finally, and perhaps most important, the integrated model structure forces one to identify and articulate the greatest uncertainties and variability that would affect model outcome (that is, model sensitivity) and the focus of future research. That last feature is perhaps the greatest value of the model. Although several assumptions and parameter estimates were adjusted in the Conolly et al. (2003, 2004) models in a stated attempt to make the models more conservative (that is, to imply greater risk for a given exposure or to open the door to alternative modes of action), the main use of the models was to provide best estimates of risk that could then be adjusted to incorporate uncertainties and variability in a health-protective manner. In the view of the models’ developers, the adjustments of some of the model parameter values were sufficiently conservative and plausible. EPA disagreed with the contention that the Conolly et al. models were conservative and sought to evaluate and identify parameters that the models were most sensitive to and sources of uncertainty in the data and the models.
The committee acknowledges and agrees with EPA that the Conolly et al. (2003, 2004) models, like most models, contain weaknesses and tenuously supported assumptions. Conolly and co-workers felt that they made several conservative assumptions in their models— use of hockey-stick rather than J-shaped models for cell proliferation, use of overall respiratory tract cancer incidence in humans to calculate basal mutation rates, and use of an upper bound on the proportionality parameter relating DPX to mutation. EPA pushed that concept further by making even more conservative assumptions within the models that cumulatively resulted in radical departures from the results of the Conolly et al. models with regard to low-dose extrapolation of tumor incidence. The committee notes that EPA forced changes in the model parameter values in a direction that yielded more conservative results rather than one that yielded a best fit to the data.
The committee is also concerned that EPA directed substantial effort toward refuting many of the assumptions and conclusions of the Conolly et al. (2003, 2004) models rather than trying to fill the data gaps that were clearly articulated by the models. One of the major strengths of the Conolly et al. models is that the developers had to reconcile all the data or specify why they could not be reconciled. The integrated model structure allows one to identify uncertainties clearly and how they may affect model behavior. Conolly and co-workers were clear on that point and expressed the need for new data that could anchor many of the parameter values that had to be optimized from rather sparse data sets. Regardless, the committee recommends that for completeness and transparency the BBDR models published by Conolly et al. (2003, 2004), with the flaw in one numeric approach identified by EPA corrected, be used in the draft IRIS assessment and that the results be compared with those of the approach that was used in the draft assessment.
CONCLUSIONS AND RECOMMENDATIONS
The draft IRIS assessment of formaldehyde provides an exhaustive discussion of formaldehyde toxicokinetics, carcinogenic modes of action, and various models. Although the committee agrees with much of the narrative, several issues need to be addressed in the revision of the draft assessment. First, there is broad agreement that formaldehyde is normally present in all tissues, cells, and bodily fluids and that natural occurrence complicates any formaldehyde risk assessment. Thus, an improved understanding of when exogenous formaldehyde exposure appreciably alters normal endogenous formaldehyde concentrations is needed. One approach that EPA could use would be to complete an analysis of variability and uncertainty in measuring and predicting target-tissue formaldehyde concentrations among species. Only with such an analysis can one begin to identify and address openly and transparently the question of how much added risk for an endogenous compound is acceptable.
Second, inhaled formaldehyde, a highly reactive chemical, is absorbed primarily in the upper airways and remains predominantly in the respiratory epithelium. The weight of evidence indicates that formaldehyde probably does not appear in the blood as an intact molecule except at doses high enough to overwhelm the metabolic capability of the exposed tissue. The draft IRIS assessment presents divergent opinions regarding the systemic delivery of formaldehyde that need to be resolved.
Third, the committee agrees with EPA that formaldehyde is a genotoxic chemical and that it is therefore reasonable to conclude that formaldehyde may act through a mutagenic mode of action. However, cytotoxicity and compensatory cell proliferation also appear to play important roles in formaldehyde-induced nasal tumors. Although the draft IRIS assessment discusses that mode of action, it relies on the mutagenic mode of action to justify low-dose extrapolations. The committee recommends that EPA provide alternative calculations that factor in nonlinearities associated with the cytotoxicity-compensatory cell proliferation mode of action and assess the strengths and weaknesses of each approach.
Fourth, over the last decade, several models have been developed to help to evaluate the risks associated with formaldehyde exposure, and EPA extensively evaluated several of them. EPA did use the CFD models to derive human equivalent concentrations but restricted their application to the experimental range used in the animal studies and did not extrapolate to low exposures. The committee, however, recommends that the CFD models be used to extrapolate to low concentrations, that the results be included in the overall evaluation, and that EPA explain clearly its use of the CFD modeling approaches. Furthermore, EPA, on the basis of extreme alternative model scenarios, chose not to use the BBDR models developed by Conolly et al. (2003, 2004); however, the committee questions the validity of some of these scenarios and recommends that the BBDR models developed by Conolly and co-workers be used (with the flaw in one numeric approach identified by EPA corrected), that the results be compared with those of the approach currently presented in the draft IRIS assessment, and that the strengths and weaknesses of each approach be discussed.
Fifth, in rewriting the sections of the draft IRIS assessment that pertain to the topics reviewed in this chapter, EPA should consider the implications of the most recent work. References to older studies on DNA-adduct measurements may need to be reanalyzed in light of the most recent analytic techniques that achieved superior sensitivity (for example, Lu et al. 2010). In particular, the committee finds the recent study of Lu et al. (2010) to be highly informative and the first one to distinguish clearly between exogenous and endogenous formaldehyde-induced DNA adducts. Although the study does not challenge the notion that DNA adducts play only a minor, if any, role in formaldehyde genotoxicity and carcinogenicity, compared with DNA-protein cross-links, it adds to the evidence of the inability of formaldehyde to reach distant sites. Likewise, the positive study by Wang et al. (2009) is not adequately described in the draft IRIS assessment, nor is it clear to the committee why so much emphasis is placed on the study by Craft et al. (1987).
REFERENCES
ATSDR (Agency for Toxic Substances and Disease Registry). 1999. Toxicological Profile for Formaldehyde. U.S. Department of Health and Human Services, Public Health Services, Agency for Toxic Substances and Disease Registry, Atlanta, GA. July 1999 [online]. Available: http://www.atsdr.cdc.gov/ToxProfiles/tp111.pdf [accessed Jan. 5, 2011].
Bogdanffy, M.S., H.W. Randall, and K.T. Morgan. 1986. Histochemical localization of aldehyde dehydrogenase in the respiratory tract of the Fischer-344 rat. Toxicol. Appl. Pharmacol. 82(3):560-567.
Bogdanffy, M.S., P.H. Morgan, T.B.Starr, and K.T. Morgan. 1987. Binding of formaldehyde to human and rat nasal mucus and bovine serum albumin. Toxicol. Lett. 38(1-2):145-154.
Boobis, A.R., G.P. Daston, R.J. Preston, and S.S. Olin. 2009. Application of key events analysis to chemical carcinogens and noncarcinogens. Crit. Rev. Food Sci. Nutr. 49(8):690-707.
Casanova, M., H.d’A. Heck, J.I. Everitt, W.W. Harrington, Jr., and J.A. Popp. 1988. Formaldehyde concentrations in the blood of Rhesus monkeys after inhalation exposure. Food Chem. Toxicol. 26(8):715-716.
Casanova, M., D.F. Deyo, and H.d’A Heck. 1989. Covalent binding of inhaled formaldehyde to DNA in the nasal mucosa of Fischer 344 rats: Analysis of formaldehyde and DNA by high-performance liquid chromatography and provisional pharmacokinetic interpretation. Fundam. Appl. Toxicol. 12(3):397-417.
Casanova, M., K.T. Morgan, W.H. Steinhagen, J.I. Everitt, J.A. Popp, and H.d’A. Heck. 1991. Covalent binding of inhaled formaldehyde to DNA in the respiratory tract of rhesus monkeys: Pharmacokinetics, rat-to-monkey interspecies scaling, and extrapolation to man. Fundam. Appl. Toxicol. 17(2):409-428.
Casanova, M., K.T. Morgan, E.A. Gross, O.R. Moss, and H.d’A. Heck. 1994. DNA–protein cross-links and cell replication at specific sites in the nose of F344 rats exposed subchronically to formaldehyde. Fundam. Appl. Toxicol. 23(4):525-536.
Casanova, M., H.d’A. Heck, and D. Janszen. 1996. Comments on ‘DNA–protein crosslinks, a biomarker of exposure to formaldehyde—in vitro and in vivo studies' by Shaham et al. [letter]. Carcinogenesis. 17(9):2097-2101.
Casanova-Schmitz, M., and H.d’A. Heck. 1983. Effects of formaldehyde exposure on the extractability of DNA from proteins in the rat nasal mucosa. Toxicol. Appl. Pharmacol. 70(1):121-132.
Casanova-Schmitz, M., T.B. Starr, and H.d’A. Heck. 1984. Differentiation between metabolic incorporation and covalent binding in the labeling of macromolecules in the rat nasal mucosa and bone marrow by inhaled [14C]- and [3H]formaldehyde. Toxicol. Appl. Pharmacol. 76(1):26-44.
Chang, J.C., E.A. Gross, J.A. Swenberg, and C.S. Barrow. 1983. Nasal cavity deposition, histopathology, and cell proliferation after single or repeated formaldehyde exposures in B6C3F1 mice and F-344 rats. Toxicol. Appl. Pharmacol. 68(2):161-176.
Conolly, R.B., P.D. Lilly, and J.S. Kimbell. 2000. Simulation modeling of the tissue disposition of formaldehyde to predict nasal DNA-protein cross- links in Fischer 344 rats, Rhesus monkeys, and humans. Environ. Health. Perspect. 108(suppl. 5):919-924.
Conolly, R.B., J.S. Kimbell, D.B. Janszen, and F.J. Miller. 2002. Dose response for formaldehyde-induced cytotoxicity in the human respiratory tract. Regul. Toxicol. Pharmacol. 35(1):32-43.
Conolly, R.B., J.S. Kimbell, D. Janszen, P.M. Schlosser, D. Kalisak, J. Preston, and F.J. Miller. 2003. Biologically motivated computational modeling of formaldehyde carcinogenicity in the F344 rat. Toxicol. Sci. 75(2):432-447.
Conolly, R.B., J.S. Kimbell, D. Janszen, P.M. Schlosser, D. Kalisak, J. Preston, and F.J. Miller. 2004. Human respiratory tract cancer risks of inhaled formaldehyde: Dose-response predictions derived from biologically motivated computational modeling of a combined rodent and human dataset. Toxicol. Sci. 82(1):279-296.
Costa, S., P. Coelho, C. Costa, S. Silva, O. Mayan, L.S. Santos, J. Gaspar, and J.P. Teixeira. 2008. Genotoxic damage in pathology anatomy laboratory workers exposed to formaldehyde. Toxicology 252(1-3):40-48.
Craft, T.R., E. Bermudez, and T.R. Skopek. 1987. Formaldehyde mutagenesis and formation of DNA–protein crosslinks in human lymphoblasts in vitro. Mutat. Res. 176(1):147-155.
Crump, K.S., R.P. Subramaniam, and C.B. Van Landingham. 2005. A numerical solution to the nonhomogeneous two-stage MVK model of cancer. Risk Anal. 25(4):921-926.
Crump, K.S., C. Chen, J.F. Fox, C. Van Landingham, and R. Subramaniam. 2008. Sensitivity analysis of biologically motivated model for formaldehyde-induced respiratory cancer in humans. Ann. Occup. Hyg. 52(6):481-495.
Crump, K.S., C. Chen, W.A. Chiu, T.A. Louis, C.J. Portier, R.P. Subramaniam, and P.D. White. 2010. What role for biologically based dose-response models in estimating low-dose risk? Environ. Health Perspect. 118(5):585-588.
Egle, Jr., J.L. 1972. Retention of inhaled formaldehyde, propionaldehyde, and acrolein in the dog. Arch. Environ. Health. 25(2):119-124.
EPA (U.S. Environmental Protection Agency). 1991. Formaldehyde Risk Assessment Update, Final Draft. Office of Toxic Substances, U.S. Environmental Protection Agency, Washington, DC. June 11, 1991.
EPA (U.S. Environmental Protection Agency). 2005. Guidelines for Carcinogen Risk Assessment. EPA/630/P-03/001F. Risk Assessment Forum, U.S. Environmental Protection Agency, Washington, DC. March 2005 [online]. Available: http://www.epa.gov/raf/publications/pdfs/CANCER_GUIDELINES_FINAL_3-25-05.PDF [accessed Nov. 23, 2010].
EPA (U.S. Environmental Protection Agency). 2010. Toxicological Review of Formaldehyde (CAS No. 50-00-0) – Inhalation Assessment: In Support of Summary Information on the Integrated Risk Information System (IRIS). External Review Draft. EPA/635/R-10/002A. U.S. Environmental Protection Agency, Washington, DC [online]. Available: http://cfpub.epa.gov/ncea/iris_drafts/recordisplay.cfm?deid=223614 [accessed Nov. 22, 2010].
Franks, S.J. 2005. A mathematical model for the absorption and metabolism of formaldehyde vapour by humans. Toxicol. Appl. Pharmacol. 206(3):309-320.
Garcia, G.J., J.D. Schroeter, R.A. Segal, J. Stanek, G.L. Foureman, and J.S. Kimbell. 2009. Dosimetry of nasal uptake of water-soluble and reactive gases: A first study of interhuman variability. Inhal. Toxicol. 21(7):607-618.
Georgieva, A.V., J.S. Kimbell, and P.M. Schlosser. 2003. A distributed parameter model for formaldehyde uptake and disposition in the rat nasal lining. Inhal. Toxicol. 15(14):1435-1463.
Heck, H.d’A., and M. Casanova. 1994. Nasal dosimetry of formaldehyde: Modeling site specificity and the effects of preexposure. Inhal. Toxicol. 6(suppl.):159-175.
Heck, H.d’A, and M. Casanova. 2004. The implausibility of leukemia induction by formaldehyde: A critical review of the biological evidence on distant-site toxicity. Regul. Toxicol. Pharmacol. 40(2):92-106.
Heck, H.d'A., E.L. White, and M. Casanova-Schmitz. 1982. Determination of formaldehyde in biological tissues by gas chromatography/mass spectrometry. Biomed. Mass Spectrom. 9(8):347-353.
Heck, H.d'A., M. Casanova-Schmitz, P.B. Dodd, E.N. Schachter, T.J. Witek, and T. Tosun. 1985. Formaldehyde (CH2O) concentrations in the blood of humans and Fischer-344 rats exposed to CH2O under controlled conditions. Am. Ind. Hyg. Assoc. J. 46(1):1-3.
Hernandez, O., L. Rhomberg, K. Hogan, C. Siegel-Scott, D. Lai, G. Grindstaff, M. Henry, and J.A. Cotruvo. 1994. Risk assessment of formaldehyde. J. Hazard. Mater. 39(2):161-172.
Hubal, E.A., P.M. Schlosser, R.B. Conolly, and J.S. Kimbell. 1997. Comparison of inhaled formaldehyde dosimetry predictions with DNA-protein cross-link measurements in the rat nasal passages. Toxicol. Appl. Pharmacol. 143(1):47-55.
Kimbell, J.S., E.A. Gross, D.R. Joyner, M.N. Godo, and K.T. Morgan. 1993. Application of computational fluid dynamics to regional dosimetry of inhaled chemicals in the upper respiratory tract of the rat. Toxicol. Appl. Pharmacol. 121(2):253-263.
Kimbell, J.S., E.A. Gross, R.B. Richardson, R.B. Conolly, and K.T. Morgan. 1997. Correlation of regional formaldehyde flux predictions with the distribution of formaldehyde-induced squamous metaplasia in F344 rat nasal passages. Mutat. Res. 380(1-2):143-154.
Kimbell, J.S., R.P. Subramaniam, E.A. Gross, P.M. Schlosser, and K.T. Morgan. 2001a. Dosimetry modeling of inhaled formaldehyde: Comparisons of local flux predictions in the rat, monkey, and human nasal passages. Toxicol. Sci. 64(1):100-110.
Kimbell, J.S., J.H. Overton, R.P. Subramaniam, P.M. Schlosser, K.T. Morgan, R.B. Conolly, and F.J. Miller. 2001b. Dosimetry modeling of inhaled formaldehyde: Binning nasal flux predictions for quantitative risk assessment. Toxicol. Sci. 64(1):111-121.
Lu, K., L.B. Collins, H. Ru, E. Bermudez, and J.A. Swenberg. 2010. Distribution of DNA adducts caused by inhaled formaldehyde is consistent with induction of nasal carcinoma but not leukemia. Toxicol. Sci. 116(2):441-451.
Lu, K., B. Moeller, M. Doyle-Eisele, J. McDonald, and J.A. Swenberg. 2011. Molecular dosimetry of N2-hydroxymethyl-dG DNA adducts in rats exposed to formaldehyde. Chem. Res. Toxicol. 24: 159-161.
Moeller, B.C., K. Lu, M. Doyle-Eisele, J. McDonald, A. Gigliotti, and J.A. Swenberg. 2011. Determination of N2-hydroxymethyl-dG adducts in the nasal epithelium and bone marrow of nonhuman primates following 13CD2-formaldehyde inhalation exposure. Chem. Res. Toxicol. 24(2):162-164.
Monticello, T.M., K.T. Morgan, J.I. Everitt, and J.A. Popp. 1989. Effects of formaldehyde gas on the respiratory tract of rhesus monkeys. Pathology and cell proliferation. Am. J. Pathol. 134(3):515-527.
Monticello, T.M., F.J. Miller, and K.T. Morgan. 1991. Regional increases in rat nasal epithelial cell proliferation following acute and subchronic inhalation of formaldehyde. Toxicol. Appl. Pharmacol. 111(3):409-421.
Monticello, T.M., J.A. Swenberg, E.A. Gross, J.R. Leininger, J.S. Kimbell, S. Seilkop, T.B. Starr, J.E. Gibson, and K.T. Morgan. 1996. Correlation of regional and nonlinear formaldehyde-induced nasal cancer with proliferating populations of cells. Cancer Res. 56(5):1012-1022.
Overton, J.H., J.S. Kimbell, and F.J. Miller. 2001. Dosimetry modeling of inhaled formaldehyde: The human respiratory tract. Toxicol. Sci. 64(1):122-134.
Patterson, D. L., E.A. Gross, M.S. Bogdanffy, and K.T. Morgan. 1986. Retention of formaldehyde gas by the nasal passages of F-344 rats. Toxicologist 6: 55.
Schlosser, P.M. 1999. Relative roles of convection and chemical reaction for the disposition of formaldehyde and ozone in nasal mucus. Inhal. Toxicol. 11(10):967-980.
Schripp, T., C. Fauck, and T. Salthammer. 2010. Interferences in the determination of formaldehyde via PTR-MS: What do we learn from m/z 31? Int. J. Mass Spectrom. 289(2-3):170-172.
Shaham, J., Y. Bomstein, A. Meltzer, Z. Kaufman, E. Palma, and J. Ribak. 1996. DNA–protein crosslinks, a biomarker of exposure to formaldehyde—in vitro and in vivo studies. Carcinogenesis. 17(1):121-125.
Shaham, J., Y. Bomstein, A. Melzer, and J. Ribak. 1997. DNA-protein crosslinks and sister chromatid exchanges as biomarkers of exposure to formaldehyde. Int. J. Occup. Environ. Health. 3(2):95-104.
Shaham, J., Y. Bomstein, R. Gurvich, M. Rashkovsky, and Z. Kaufman. 2003. DNA-protein crosslinks and p53 protein expression in relation to occupational exposure to formaldehyde. Occup. Environ. Med. 60(6):403-409.
Spanel, P., and D. Smith. 2008. Quantification of trace levels of the potential cancer bio-markers formaldehyde, acetaldehyde and propanol in breath by SIFT-MS. J. Breath Res. 2(4):1-10.
Subramaniam, R.P., K.S. Crump, C. Van Landingham, P. White, C. Chen, and P.M. Schlosser. 2007. Uncertainties in the CIIT model for formaldehyde-induced carcinogenicity in the rat: A limited sensitivity analysis-I. Risk Anal. 27(5): 1237-1254.
Subramaniam, R.P., C. Chen, K.S. Crump, D. DeVoney, J.F. Fox, C.J. Portier, P.M. Schlosser, C.M. Thompson, and P. White. 2008. Uncertainties in biologically-based modeling of formaldehyde-induced respiratory cancer risk: Identification of key issues. Risk Anal. 28(4):907-923.
Swenberg, J.A., C.S. Barrow, C.J. Boreiko, H.d’A. Heck, R.J. Levine, K.T. Morgan, and T.B. Starr. 1983. Non-linear biological responses to formaldehyde and their implications for carcinogenic risk assessment. Carcinogenesis. 4(8):945-952.
Swenberg, J.A., K. Lu, B.C. Moeller, L. Gao, P.B. Upton, J. Nakamura, and T.B. Starr. 2011. Endogenous versus exogenous DNA adducts: Their role in carcinogenesis, epidemiology and risk assessment. Toxicol Sci. 120(Supl.1):S130-S145.
Wang, M., G. Cheng, S. Balbo, S.G. Carmella, P.W. Villalta, and S.S. Hecht. 2009. Clear differences in levels of a formaldehyde-DNA adduct in leukocytes of smokers and nonsmokers. Cancer Res. 69(18):7170-7174.
Yang, Y., B.C. Allen, Y.M. Tan, K.H. Liao, and H.J. Clewell III. 2010. Bayesian analysis of a rat formaldehyde DNA-protein cross-link model. J. Toxicol. Environ. Health A. 73(12):787-806.