3
Materials Research Opportunities
Materials research (MR) spans the spectrum from end-use application in manufactured components to fundamental studies of complex many-body phenomena. A classical approach to evaluating MR opportunities is organized along specific types or classes of materials, such as metallic alloys, semiconductors, superconductors, and ceramics; such a discipline-specific research focus strongly leverages current state-of-the-art knowledge and has a proven track record of successful MR advances as documented in part in Chapter 2. Alternatively, use-inspired research applications such as energy or data science can motivate broad crosscutting materials research and development (R&D) that lead to beneficial societal applications. Furthermore, fundamental discovery science endeavors can catalyze new scientific advances that reach beyond the current materials knowledge base and may launch entirely new material functionalities. These three approaches to MR are not mutually exclusive, and in general each approach benefits from knowledge gained from the other approaches. This chapter will outline examples of compelling MR opportunities anticipated to arise in the coming decade. These research opportunities are categorized according to classical material type at the start of this chapter (Sections 3.1 to 3.6), followed by several sections of use-inspired cross-disciplinary MR opportunities (Sections 3.7 to 3.9). Discovery science is not explicitly discussed in this chapter since by its nature it involves unfettered exploratory research.
3.1 METALS
This section describes in the first part fundamental studies of metals and alloys including high-entropy alloys (HEAs). Further discussions relate to nanostructured metallic alloys.
3.1.1 Fundamental Studies of Classical Metals and Alloys
Fundamental studies of metals and alloys underpin basic MR by illuminating with unprecedented clarity and fidelity the atomic and mesoscale processes associated with phase transformations and the formation and evolution of defects and interfaces, which govern the synthesis and performance of not only metals and alloys but also materials in general. Studies of metals have provided the basis for understanding the structure-dependent properties and processing of not only metals but also ceramics, semiconductors, composites, and hybrid materials across ever-broadening length and time scales.1 Buoyed by robust thermodynamic and structural databases, foundational advances in computational materials science and engineering coupled with in situ, in operando, and post-mortem three-dimensional (3D) and time-dependent four-dimensional (4D) characterization of metals and alloys are ushering in a digital age of materials development. During the next decade, fundamental research in metals and alloys is anticipated to continue to enable revolutionary new science and deeper understanding of material behavior, which will lead to new material devices and systems. Metals are ubiquitous, from steel, aluminum, and nickel-base superalloys for energy production, infrastructure, and transportation, to titanium and shape memory alloy biomedical implants, to metallic thin films and integrated circuit vias for thermal and electrical functionality, and nanoparticles for catalysts and cancer treatment. Promising research areas for the coming decade include coupled experimental and computational modeling studies performed at the same length and time scale (heretofore not possible owing to experimental and computational limitations), real-time analysis of in situ/in operando experimental characterization data (rather than conventional post-measurement detailed analyses), processing and compositional innovations to enable next-generation high-performance lightweight alloys, ultra-high-strength steels and refractory alloys, and design and fabrication of multifunctional highly architected materials systems. Emerging opportunities for advanced manufacturing of metals and other materials are discussed in Chapter 5. Two examples of metals research that have recently garnered significant attention and hold considerable promise are summarized below; to highlight continuing advances in our understanding of
___________________
1 C.A. Handwerker and T.M. Pollock, 2014, Emerging science and research opportunities for metals and metallic nanostructures, Journal of the Minerals, Metals & Materials Society 66(7):1321-1341.
processing-structure-property-performance relations. The choice of these topics is inherently subjective, and it is essential to maintain broad support for the field so as not to overlook the surprises that turn out to be strikingly important.
3.1.2 High-Entropy Alloys
HEAs, also known as compositionally complex or multiprincipal element alloys (MPEAs), inhabit a vast compositional space, and the advances attained in the past decade likely represent only the tip of the iceberg in the development of this promising class of alloys.2 HEAs differ from conventional alloys (where there is typically one predominant element known as the solvent and one or more minor concentration elements) in that five or more elements are present at comparable concentrations. It was originally believed that this might increase the configurational entropy, but the role of entropy is no longer thought to be important. Nevertheless, it is clear that other concentrated alloying effects not present in conventional alloys are at play. It appears that synergistic effects of multiple elements acting in parallel or in sequence produce results that are difficult to achieve in conventional alloys. For example, when it comes to mechanical behavior, one might be able to overcome the strength-ductility trade-off that is a long-standing dilemma in structural materials. With proper scientific understanding, one could envision tailoring the stacking fault energies, twin energies, phase instabilities, and so on to trigger multiple transformation toughening mechanisms. At ambient temperatures, this would result in high toughness and energy absorption, which could in turn be employed in crumple zones in automobiles, increased blast resistance, or reducing cyclic fatigue. To predict how different alloying elements contribute to strength and toughness, researchers need to understand how each element affects its local environment. Simply using Goldschmidt radii to calculate volume misfits is not applicable in concentrated alloys. The local “chemistry” and atomic interactions are important. At the other extreme, doing a full-blown density functional theory (DFT) calculation for each case is out of the question. It would help to have simple “correlations” for how different elements affect strength using physical parameters that are relatively easy to measure. At elevated temperatures, one might be able to utilize “sluggish” diffusion to kinetically limit phenomena that would otherwise be thermodynamically possible. While single-phase MPEAs are not likely to be useful as high-temperature materials, the development of multiphase MPEAs, similar to Ni-base superalloys, hold significant promise. A better understanding of solid solution effects in MPEAs could help researchers to realize better multicomponent phases. Additionally, certain phases, and reaction pathways between phases, may
___________________
2 D.B. Miracle and O.N. Senkov, 2017, A critical review of high-entropy alloys and related concepts, Acta Materialia 122:448-511.
exist only in higher-order systems. By accessing these, one might be able to create microstructures that are not possible in simpler (conventional) alloys. Doing this efficiently and cost effectively will require development of reliable experimental and computational thermodynamic databases that are currently very limited in both scope and breadth.
3.1.3 Nanostructured Metallic Alloys
Transformational advances in nanoscale materials synthesis, characterization, and modeling have spawned a plethora of nanoscience activities to exploit processing-structure-properties relations and create nanostructured materials that exhibit ultrahigh strength and other unusual properties.3 Nanoscale disturbance of dislocation-based plasticity underpins the improved strength, but may reduce overall ductility. The strength-ductility trade-off is evidenced by the fact that nanocrystalline metals typically have higher hardness and strength than conventional microcrystalline counterparts but generally exhibit much lower tensile ductility. Nanocrystalline metals are also susceptible to thermal and mechanical microstructural instabilities and have lower electrical conductivity. By comparison, nanotwinned metals provide strength enhancements similar to those for nanocrystalline materials while also maintaining a more beneficial balance of properties—for example, high ductility, improved microstructural stability, and low electrical resistivity.4,5 Numerous studies have demonstrated these enhancements in low-stacking-fault-energy metals and alloys and related them to the activation of hard and soft slip systems, interactions of dislocations with coherent twin boundaries, and detwinning. Recent reports of nanotwinned NiMoW alloys with strength several times greater than high-strength steel, enhanced microstructural stability, tailorable ductility, and electrical conductivities comparable to bulk alloys, extends the synthesis of nanotwinned materials to high-stacking-fault-energy, high-temperature alloys.6 Building on the extensive insights on nanoscale materials synthesis, characterization, and modeling performed during the past decade, there are several important concepts still to be resolved. In particular, experiments and
___________________
3 National Science Technology Council, 2017, The National Nanotechnology Initiative: Supplement to the President’s 2018 Budget, https://www.nano.gov/sites/default/files/NNI-FY18-Budget-Supplement.pdf.
4 L. Lu, Y. Shen, X. Chen, L. Qian, and K. Lu, 2004, Ultrahigh strength and high electrical conductivity in copper, Science 304(5669):422-426.
5 I.J. Beyerlein, X. Zhang, and A. Misra, 2014, Growth twins and deformation twins in metals, Annual Review of Materials Research 44:329-363.
6 G.-D. Sim, J.A. Krogstad, K.M. Reddy, K.Y. Xie, G.M. Valentino, T.P. Weihs, and K.J. Hemker, 2017, Nanotwinned metal MEMS films with unprecedented strength and stability, Science Advances 3(6):e1700685.
modeling to identify deformation mechanisms in nanotwinned materials, and the role of resolved stresses, and better understanding of microstructural evolution are still needed. Armed with this knowledge, an understanding of the influence of alloy chemistry and deposition parameters would allow for tailoring of nanotwins and properties in a wide variety of materials. In the coming decade, it may be possible to achieve tailored design and fabrication of nanostructured materials containing multiple hardening approaches (e.g., finely dispersed matrix nanoparticles in nanotwinned material) that would enable a dramatic improvement in high-strength, high-toughness materials.
3.2 CERAMICS, GLASSES, COMPOSITES, AND HYBRID MATERIALS
This section begins with a discussion of ceramics and inorganic glasses, the ubiquitous defect structures that determine their properties, and processing opportunities for greater energy efficiency. Also introduced are composites and hybrid materials, including hybrid nanocomposites and soft machines, a rich area of opportunity for MR demanding expertise in diverse materials types, and the interactions that occur at the interfaces between them.
3.2.1 Ceramics and Glasses
Emerging opportunities in ceramics and glass research were identified in a National Science Foundation (NSF)-sponsored workshop held in September 2016.7 In addition to the topics highlighted below are ceramic- and glass-related content in the section on materials for extreme environments (see Section 3.7.3), thermal barrier coatings (see Section 3.9.4), and additive manufacturing (AM; see Section 4.2.4).
Energy-Efficient Ceramic Processing
Historically, the densification of ceramic bodies is conducted using high-temperature sintering, which often requires hours for completion. Over the past decade, field-assisted processes, either by current flow or by noncontacting applied fields, have resulted in enhanced densification at significantly lower temperatures and shorter times. For conducting ceramics, the current assistance depends, at least in part, upon Joule heating, while field effects on the densification of insulating ceramics remain an area of debate. An understanding of point-defect formation and
___________________
7 K.T. Faber, T. Asefa, M. Backhaus‐Ricoult, R. Brow, J.Y. Chan, S. Dillon, W.G. Fahrenholtz, et al., 2017, The role of ceramic and glass science research in meeting societal challenges: Report from an NSF‐sponsored workshop, Journal of the American Ceramic Society 100(5):1777-1803.
migration in a noncontacting electric field, and alteration of space charge zones at grain boundaries and free surfaces, is ultimately required to allow microstructural tailoring for given applications.
A significant advantage of these methods, beyond their greater energy efficiency, is their ability to produce dense solids that cannot be made by other methods. These include nanocrystalline solids, which tend to coarsen during traditional high-temperature sintering, and ultra-high-temperature ceramics (see Section 3.7.3) whose melting points exceed 3000°C. In the latter, Joule heating by internal currents reduces the required temperature for densification. Further, the high heating rates and short processing times coupled, in some cases, with pressure can generate some intriguing nonequilibrium microstructures.
A second shift in ceramic processing adapted from classical hydrothermal techniques, known as cold sintering, is also gaining attention. Ceramic bodies have been shown to densify at temperatures as low as 25°C to 300°C via a transient liquid phase water coupled with high pressures.8 Ceramic-metal and ceramic-polymer composites are particularly attractive candidates for cold sintering, since the method provides a pathway to fabrication that precludes reaction between constituents or melting or decomposition of the second phase. Little is known, however, about the fundamental mechanisms of densification in the presence of the fugitive liquid phase at and near ambient temperatures.
The Defect Genome
Defects over multiple length scales have long played a role (and in many cases, a desirable role) in the performance of ceramic materials—point defects for transport properties; line defects for deformation; planar defects for ferroelastic, piezoelectric, and ferroelectric response; and volume defects for mechanical properties. Likewise, defect concentrations and their interactions over time and length scales are known to influence functionality. A “Defect Genome” initiative,9 whereby defects in ceramics over multiple length scales are characterized and their response under thermal gradients, stress, and electric or magnetic fields are understood and defect-defect interactions are made computationally and experimentally tractable, will add a new dimension to materials design. Ultimately, precise defect placement, coupled to atomic placement, would be the target.
As one example, in the continuing understanding of planar defects in polycrystalline materials, grain boundaries have a profound significance. The concept of
___________________
8 J. Guo, H. Guo, A.L. Baker, M.T. Lanagan, E.R. Kupp, G.L. Messing, and C.A. Randall, 2016, Cold sintering: A paradigm shift for processing and integration of ceramics, Angewandte Chemie 128:11629.
9 J. Balachandran, L. Lin, J.S. Anchell, C.A. Bridges, and P. Ganesh, 2017, Defect genome of cubic perovskites for fuel cell applications, Journal of Physical Chemistry C 121(48):26637-26647.
grain-boundary “complexions” was proposed in 2014. Grain boundaries behave as their own phase with discontinuous changes in mobility, diffusivity, and cohesion behavior, and are therefore differentiable from stand-alone materials. The changes lead to opportunities to tailor properties via processing routes or composition. Time-temperature-transformation diagrams specific to the grain-boundary complexions10 have already added new insight to phenomena such as abnormal grain growth.11 Understanding grain-boundary phase evolution with respect to grain phase evolution will help design composition and processing to achieve the goals of the Materials Genome Initiative (MGI).
A second timely example that would benefit from the Defect Genome is the family of oxide perovskites, structurally ABO3. Oxide perovskites are being investigated as catalysts in solar-to-fuel conversion reactions through solar-driven redox cycles,12 as well as for oxygen-transporting membranes. Perovskites can incorporate multiple A-site and B-site cations into their ABO3-δ lattice to form A1-xA’xB1-yB’yO3-δ structures. Because of the variety of the substitutional cations, a range of electronic, ionic, or magnetic properties can be attained. Moreover, oxygen activity and reactivity can be controlled via the stoichiometry of the final structure. Typically, three types of point defects can exist in the perovskites: electronic defects (in either undoped or doped structures), oxygen vacancies, and cation vacancies. Multiple cation types coupled with mixed defects create an immense phase and defect space, for pathways to exceptional properties and stability. For example, it has been demonstrated that bandgaps can be tailored by acceptor-doping in a variety of families: manganites, ferrites, cobaltates, chromates, and aluminates. By coupling the Materials Genome with the Defect Genome, a fundamental understanding of this class of oxides can be achieved along with pathways for the design of new perovskites.
Glasses
A paper from 2013 lays out several areas of glass research important to the glass industry, including, among others, structure-property relations, densification,
___________________
10 P.R. Cantwell, M. Tang, S.J. Dillon, J. Luo, G.S. Rohrer, and M.P. Harmer, 2014, Grain boundary complexions, Acta Materialia 62, doi:10.1016/j.actamat.2013.07.037.
11 P.R. Cantwell, M. Shuailei, S.A. Bojarski, G.S. Rohrer, and M.P. Harmer, 2016, Expanding time–temperature-transformation (TTT) diagrams to interfaces: A new approach for grain boundary engineering, Acta Materialia 106:78-86.
12 M. Kubicek, A.H. Bork, and J.L.M. Rupp, 2017, Perovskite oxides—a review on a versatile material class for solar-to-fuel conversion processes (review article), Journal of Materials Chemisry A, 5:11983-12000, doi:10.1039/C7TA00987A.
and strength in glass.13 Two new application areas mentioned in those studies are energy storage14 and quantum communications. Solid-state batteries can solve both energy density and fire-safety concerns compared to conventional Li-ion batteries (see, for example, Case 5 in Section 5.3). Both glassy and crystalline solid electrolyte materials, under study at multiple institutions, are designed to meet those needs and concerns.15 Early generations of solid electrolyte were based on LAMP chemistries (LAMP is lithium aluminum metal phosphate; the metal can be Ge, Al, etc.), but those materials were unable to stop dendritic growth leading to early failures of the batteries. Recent Li-stable chemistries include crystalline lithium lanthanum zirconium oxide (LLZO) and amorphous sulfide-based materials. LLZO stabilized in the cubic phase has high enough conductivity (~10−4 S/cm−1) to be practical, if point contact can be eliminated to prevent dendritic growth.
Another example where glasses are rapidly making inroads is in quantum communication, even though there are still needs such as improved single-photon generation and detection and low-loss optical transmission. Quantum communications will benefit from the wafer processing of optical components.16 Areas of active research include silicon on insulator-type structures, III-V materials, silica wafers with femtosecond laser written features, and nonlinear optical materials such as lithium niobates. Each type of material will need advanced processing, characterization, and modeling to achieve the desired performance goals.
3.2.2 Composites and Hybrid Materials
Composites
The introduction of advanced polymer resin matrix materials and high-performance fiber reinforcements will naturally provide increased structural performance (stiffness, strength, toughness), but future applications will require exploitation of improved tailorability of properties and multifunctional performance. Multidimensional fiber reinforcements that follow natural load paths across 3D space and have spatially optimized properties will enable increased structural efficiency over today’s 2D laminates. The joining of composite components organically, without fasteners, offers manufacturing and performance benefits and requires analysis
___________________
13 J.C. Mauro and E.D. Zanotto, 2014, Two centuries of glass research: Historical trends, current status, and grand challenges for the future, International Journal of Applied Glass Science 5:313-327.
14 J.W. Fergus, 2010, Ceramic and polymeric solid electrolytes for lithium-ion batteries, Journal of Power Sources 195(15):4554-4569.
15 B.V. Lotsch and J. Maier, 2017, Relevance of solid electrolytes for lithium-based batteries: A realistic view, Journal of Electroceramics 38(2-4):128-141.
16 A. Orieux and E. Diamanti, 2016, Recent advances on integrated quantum communications, Journal of Optics 18(8).
tools and in situ process control for the necessary confidence of bondline qualities. Innovations in composite materials constituents are needed to provide tailorable functionalities such as increased electrical and thermal conductivity, and acoustic performance. Early demonstrations of nanoscale tailorability and of integration with biomaterials opens up new horizons for composite behavior. And the development of composite materials that have adaptive properties, that is, properties that can change in a desirable and predictable manner over time, will provide new levels of component performance across many fields. Whereas historically composites have been largely optimized for structural performance, development and exploitation of the many innovative processing and fabrication approaches for composite materials can transform production systems and factories and fabrication costs. A variety of polymers beyond epoxies are mature enough to incorporate, including thermoplastic polymers. Multiple processing approaches to transform the polymer to a cured or consolidated state, other than autoclave or thermal processes, have promise and feasibility. Analysis and predictive tools that enable rapid evaluation and accurate prediction of complex behaviors of composites that have been tailored across multiple scales, dimensions, and constituents will be required to open up the true performance of this class of engineered materials.
To bring these materials to their full potential as described, a more robust and richer suite of multiscale modeling tools must be developed, including tools that model the processing—capturing the kinetics and stresses as the material is processed into its final structure—and tools that can predict the material behavior under its ultimate intended use environment and loading. Significant advances in specialized fields have been noteworthy, but the integration of modeling and simulation tools as well as their extension to a broader range of constituents is needed. Hybrids that bring together hard materials such as metal and ceramics with polymeric composite materials have been shown to withstand quite extreme environments. The properties that can be achieved by integrating and blending their extremely different properties (still such that the resulting hybrid material can be fabricated) is an emerging area that requires broader exploration to determine the limitations of these concepts. Exploration and maturation of multidimensional reinforcements and gradient morphologies will require not only advances in process simulation and multiscale modeling but also parallel and integrated manufacturing science research to explore the limitations of building such innovative engineered materials. The opportunity for computation as well as data analytics for this class of materials is great. Fundamental research in these complex engineered materials may provide foundations for other complex engineered materials such as metamaterials and biomaterials, collectively driving the cross-discipline training necessary for successful development of engineered and composite/hybrid materials.
Despite the commercialization of ceramic-matrix composites (CMCs) in the leading-edge aviation propulsion (LEAP) engine introduced in 2017 (see Box 2.4
and Case 2 in Section 5.3), state-of-the-art materials are limited to use temperatures of 1250-1300°C.17 Residual silicon, a remnant of the reactive melt-infiltration process of the SiC matrix, precludes its use at higher temperatures. Achieving operating temperatures greater than 1400°C for SiC-based systems is likely to require vapor deposition processes or preceramic polymers for silicon-free composites. Future strategies might include self-healing compositions or new melt-infiltration systems for more refractory systems. In the long term, new systems will require materials and microstructural architecture design to allow for use temperatures greater than 1540°C.
As operating temperatures increase, any airborne dust, such as sand or volcanic ash, is likely to deposit, melt, and react with CMC engine components. Although efforts to address this issue have been taken, no proven solutions are at hand. Recent efforts have had an emphasis on multilayer solutions—specifically, thermal barriers to reduce temperature and environmental barriers to prevent reactions. The problem is particularly troublesome because the reaction product—with molten dust and the current environmental barriers—is poorly matched with the underlying CMC, and hence, results in cracking and spallation. Resolutions to this will require new coating chemistries that will either preclude reaction with molten dusts or react quickly with them to form stable and adherent phases.
Hybrid Materials and Nanocomposites
In the past decade, single-junction solar cells made of organo-metal-trihalide perovskites18 have gone from 3.8 percent conversion efficiency in 2009 to a record 22 percent in 2016.19 The perovskites take the structure AMX3, where A is an organic cation, commonly methylammonium (MA) or formamidinium (FA), M is a metal such as lead (Pb), and X is a halide anion such as bromine or iodine. The first structures used were in the form of MAPbI3. However, the smaller bandgap of FAPbI3 has led to its use by some researchers. This level of dramatic improvement was achieved through modifying the layers adjacent to the methylammonium lead halide, improving the chemistries of the perovskite itself and employing novel structures. A separate layer for hole transport has been eliminated, as the perovskite can transport both electrons and holes. Improvements in thin film deposition
___________________
17 C.S. Corman, R. Upadhyay, S. Sinha, S. Sweeny, S. Wang, S. Biller, and K. Luthra, 2016, “General Electric Company: Selected Applications of Ceramics and Composite Materials,” p. 224 in Materials Research for Manufacturing (L.D. Madsen and E.B. Svedberg, eds.), Springer Series in Materials Science, https://www.springer.com/series/856.
18 M. Grätzel, 2017, The rise of highly efficient and stable perovskite solar cells, Accounts of Chemical Research 50(3):487-491, doi:10.1021/acs.accounts.6b00492.
19 J. Seo, J.H. Noh, and S.I. Seok, 2016, Rational strategies for efficient perovskite solar cells, Accounts of Chemical Research 49(3):562-572, doi:10.1021/acs.accounts.5b00444.
techniques and architecture structures, such as bicontinuous pillared structures, have also been employed. Both materials and process are fairly inexpensive in that wet chemistry and not high-temperature vacuum deposition, as in silicon solar cells, is used in manufacturing.
Perovskites transport both electrons and holes, which can be inadvertently recombined, decreasing efficiency or lifetime. If a single crystal-like structure, using inexpensive wet chemistry or other processing techniques, can be achieved, then defect interactions, especially at grain boundaries, can be reduced or eliminated. Stability of methylammonium-based perovskite solar cells remains an issue, as they degrade near 85°C; improvements are expected from encapsulation, structural, thermal, and atmospheric stability. Mixing MA and FA together can achieve both low- and high-temperature stability, but problems remain upon exposure to heat and humidity, as the compounds can be attacked by other chemistries in the stack. Eliminating the need for toxic elements is also a potential focus for future work. Some researchers are investigating A2BB′X6 halide double perovskites with silver and indium as the two cations.
One of the primary barriers inhibiting the full practical realization of hybrid nanocomposites is an insufficient ability to control the structure and dispersion state of polymer-nanoparticle mixtures.20 This stems from the fact that most inorganic nanoparticles are hydrophilic, while most polymers are hydrophobic, which inherently hinders polymer-nanoparticle miscibility, leading to poor nanoparticle dispersions. Increased miscibility can be achieved either by adding functional groups to polymer chains or by functionalizing the nanoparticles with small ligand molecules or large polymeric grafts. The latter strategy has led, in some instances, to the development of a new class of amphiphiles capable of self-assembling into a variety of superstructures reflecting their unique hydrophilic/hydrophobic balance. Additional routes for improving dispersion are to alter the processing parameters (e.g., use of extrusion to promote directional nanoparticle flow, selection of casting solvents exhibiting a preference for nanoparticle surfaces, etc.) to drive the systems into metastable states exhibiting favorable properties and long lives or to push them into equilibrium states that are far from quiescent using electric or magnetic fields.
With respect to polymer-nanoparticle hybrid materials and nanocomposites, setting out the behaviors of the phases in carefully outlined superstructures, as well as assembling the nanoparticles, has seen much advancement. Unfortunately, there remains perplexity around hard nanoparticles combined with thread-like polymers spanning comprehensive parameter space. Further, issues remain when creating functionalized nanoparticles. Despite thorough attempts, poor understanding of
___________________
20 S.K. Kumar, V. Ganesan, and R.A. Riggleman, 2017, Perspective: Outstanding theoretical questions in polymer-nanoparticle hybrids, Journal of Chemical Physics 147:020901.
the system dynamics, such as the various components’ mobilities, also persists. It is imperative that future work emphasize how external fields (electric, magnetic) affect active nanoparticle assembly. The definitive obstacle currently preventing practical applications of these hybrid materials involves understanding and controlling how the assembly states affect engineering properties that arise, and how to tune them.
Hydrogels swell in response to changes in humidity, temperature, pH, and salt concentration. To date, researchers have only scratched the surface of how geometry, chemistry, and electrostatics that generate large deformation can be used to stimulate intriguing and useful advances in hybrid materials. Another area that is receiving considerable attention is that of “soft robotics,” where the goal is to replace hard and stiff linkages and pneumatic actuators with softer, more compliant and active materials. The materials paradigm is that hard materials offer great structural capacity but very small displacements, while soft materials provide a much greater range of displacement but very low load-carrying capacity. Hybrids of hard and soft materials with distributed actuation appear to be one of the most promising solutions for multimaterials robots.
3.3 SEMICONDUCTORS AND OTHER ELECTRONIC MATERIALS
The directions for semiconductors and other electronic materials described in Chapter 2 provide much of the guidance for where opportunities will lie in this field for the next 5 to 10 years.21 Materials must enable electronics to tackle increasingly complex monolithic integration schemes and bring new functionality onto microprocessors, must allow for the construction of chips that take full advantage of 3D layouts and design, and must utilize advanced packaging solutions that improve the performance and form factor of heterogeneous electronics.
Many of the new and emerging devices promise dramatic advances in information technology in the post-Moore’s law era, but their success will depend on the properties of materials that are new to electronics and photonics, and the ability to integrate these materials into nm-scale device structures. To achieve this, one must consider not only the materials, but also the system in which they will be working. Furthermore, no one can predict what new possibilities for information technology will result from blue-sky research in areas such as topologically protected and quantum-coherent materials.
___________________
21 The committee thanks Thomas Theis, IBM (Emeritus), for his insightful thoughts and ideas in developing this portion of the report.
3.3.1 Device Miniaturization and Advances Beyond Miniaturization
Many of the device miniaturization efforts discussed in Chapter 2, such as continued efforts to develop extreme ultraviolet lithography fabrication capabilities and pursuit of advances in thin film ferroelectrics, will be a focus over the next few years. Research needed to advance information and communication technologies, in addition to continued miniaturization, are discussed in the following sections.
New Devices Combining the Functions of Memory and Logic
Magnetism has long been the basis for information storage devices such as the hard-disk drive and spin transfer torque magnetic random-access memory (STT-MRAM) discussed in Chapter 2, but recent materials discoveries have raised the possibility of magnetic devices for digital logic. With the digital state of each device represented by a persistent magnetic polarization, there would be no need to save the state of a computation before the power is turned off. This capability could be of immediate value in power-starved systems dependent on intermittent power sources, and in the longer term, it could profoundly change computer architecture. First, however, the energy efficiency of magnetic polarization switching must be made competitive with that of electronic switching. One exciting research frontier is the study and demonstration of mechanisms for voltage-controlled magnetism in ferromagnetic and antiferromagnetic materials.22
Neuromorphic Devices
Artificial neural networks are being aggressively explored as energy-efficient architectures for execution of machine learning algorithms. With the explosive growth in applications of machine learning, ANNs could become the most profound development in computer architecture in many decades. However, attaining their full potential may require the introduction of new devices that more compactly and efficiently implement key network functions. For example, much effort has gone into the development and demonstration of analog memory devices to store the weights of synaptic connections, but the material properties and resulting device characteristics are still far from ideal for this application.
___________________
22 See S. Fusil, V. Garcia, A. Barthelemy, and M. Bibes, 2014, Magnetoelectric devices for spintronics, Annual Review of Materials Research 44:91 and C. Song, B. Cui, F. Li, X. Zhou, and F. Pan, 2017, Recent progress in voltage control of magnetism: Materials, mechanisms, and performance, Progress in Materials Science 87:33.
3.3.2 Multifunctional Devices and the Internet of Things
The concept of the Internet of Things (IoT), a network of devices, machines, appliances, and other items embedded with sensors, actuators, other electronics, and software that continually communicate and interact, has been around for some time. The next 10 years will see IoT extend to more and more spaces and applications, triggering demands for commensurate technologies and the materials required to meet those needs.
Mines, industrial plants, and power facilities will be monitored for process controls; residential and commercial spaces for safety and convenience; farms for crop and other agricultural management; and hospitals for overall connectivity and patient service. Sensors will be needed to monitor patients’ conditions and symptoms; to test the air for pollution and the presence of odors and toxic gases; to test soils for the presence of nutrients, pollutants, and water; to monitor traffic control and security in cities; and to track water usage for canal management. Most of these sensors will need to be inexpensive and off-the-shelf, detecting light, motion, or pressure, but much more advanced sensors will also be needed and will have to be built at the frontiers of physics, chemistry, engineering, and biology, utilizing cutting-edge materials. As just one example, sensors for harsh environments, where temperatures remain higher than 200°C, will require new materials. STT-MRAM (discussed in Chapter 2) offers opportunities in the area of embedded memory as an alternative or replacement to NOR flash, as it is projected to work well in such harsh environments.
Many of the electronic devices that will meet these needs will be multifunctional, including microelectromechanical systems (MEMS) discussed in Chapter 2, and there will be materials challenges associated with supplying increasingly complex and capable devices. Materials selection for the structural elements of current MEMS devices is limited to a small subset of materials that are compatible with conventional very-large-scale integration (VLSI) fabrication technologies, with silicon being the dominant structural material. Suspended single-crystalline silicon or polysilicon elements, like those found in accelerometers, are easily fabricated with deep reactive ion etching and are dimensionally stable at room temperature. They cannot, however, be used at elevated temperatures because silicon creeps at elevated temperatures and junction leakage occurs at temperatures as low as 120°C.23 Ceramics such as silicon dioxide, silicon nitride, silicon carbide, and silicon-carbo-nitride have been incorporated into MEMS devices that can operate at higher temperatures, but wide application of these materials has been limited by low tensile strengths, high residual stresses, and complex fabrication processes.
___________________
23 P.G. Neudeck, R.S. Okojie, and L.-Y. Chen, 2002, High-temperature electronics—A role for wide bandgap semiconductors?, Proceedings of the IEEE 90(6), doi:10.1109/JPROC.2002.1021571.
Moreover, many forward-looking MEMS applications will require materials that combine high electrical conductivity with mechanical integrity. Advanced metallic alloys possess an attractive balance of properties (stiffness, strength, density, conductivity, etc.), but deposition and micromachining techniques for the formation of complex MEMS components from these alloys is still in its infancy. Thermoplastic forming of bulk metallic glass is intriguing as a fabrication method for low- to moderate-temperature MEMS devices. Electrodeposition and physical vapor deposition provide alternative routes to depositing metallic alloys, but these films are often deposited far from equilibrium and with microstructures that are much finer and often less stable than for bulk alloys. Once these issues are understood scientifically, the development of technologies for the deposition and shaping of metal MEMS alloys holds great promise and will enable realization of the IoT.
Development of tomorrow’s IoT sensors will also require materials with enhanced functional properties. Take, for example, sensors that serve as electronic “noses.” Such sensors must detect trace amounts of gases, inorganic or organic, but will probably need a different material and functionality or physical process for every category of gas to be detected. This is, and will continue to be, an area of active, forefront research. Likewise, medical sensors will need to be able to go into the human body, and either disintegrate, be naturally expelled, or be surgically extracted. Their design presents significant demands. They will need to detect extremely weak signals that combine electronic, rheological, chemical, and biological properties and must be designed with certain critical tolerances.
Other areas under active R&D are developing capabilities in the inspection and optimization of defects, parts, and processes in the manufacturing environment.
3.3.3 Next-Generation Semiconductors for Radio Frequency and Power Electronics
Next-generation information and energy systems will require a new class of electronic materials and devices that can provide higher power density, higher efficiency, and a smaller footprint than that possible with conventional semiconductors such as silicon. Electronic materials such as wide-bandgap semiconductors GaN, AlN, Ga2O3, and diamond have a higher breakdown field that can enable higher power density and gain at significantly higher frequencies, leading to disruptive advances in mm-wave and THz frequency communication and radar technologies. Research toward realization of high breakdown strength and synthesis of low-defect density layers could also enable highly efficient solid-state power electronics at voltages ranging from a few hundred volts to tens of kilovolts. Last, explorative studies on the integration of materials that exhibit new phenomena such as Mott-insulator transitions and ferroelectricity into semiconductor device platforms could enable
a range of unconventional new architectures for logic, communication, sensing, and energy conversion.
3.3.4 Interconnects and Packaging
The changes in integration and packaging, and the emergence of new devices such as field-effect-transistors, spintronic devices, and photonic devices, will also impose new restrictions on interconnects that will require materials solutions. The transition from 2D to 3D monolithic integrated circuits requires stacked layers of transistors, which poses challenges for interconnect routing and thermal budget control. For spintronics and photonics, signal conversion and density in interconnect hierarchy must be considered. Spin-based propagation heavily depends on relaxation length and time, which results in restrictive specifications for signal volatility and integrity. Optoelectronic device performance scales nonmonotonically with device length owing to design restrictions, which causes trade-offs of signal quality with density scaling. Last, integrating interconnects with new devices will require thermal budget matching, resistive-capacitive (RC) management, and electrical contact with new materials.
The increasing diversity and complexity of products, from small embedded sensors to heterogeneous “systems on a chip,” also pose growing technical challenges for packaging. Today’s heterogeneous systems integrate elements that were formerly relegated to board-level integration, such as passive components (capacitors, inductors, etc.) and active components (antennas and communication devices such as tunable filters), as well as memory and logic. While this allows more function per unit volume, it also accentuates challenges such as the management of within-package power density, thermal density, and the maintenance of signal integrity. It also increases assembly complexity and costs.
3.4 QUANTUM MATERIALS
Quantum materials were previously defined as materials whose properties cannot be explained by simple Fermi liquid theory, or those with strong electronic correlations. In the past decade, this definition has been broadened to include 2D and topological materials—materials whose electronic functionality reaches beyond simple 3D metals, semiconductors, and insulators. There exist many families of quantum materials, even dozens in the subset of unconventional superconductor, whose fundamental properties remain to be solved. The two solved families are the electron-phonon coupling of Cooper pairs within the Bardeen-Cooper-Schrieffer theory of conventional superconductivity, and the quantum and fractionalized quantum Hall effects (the discovery of both of these phenomena led to Nobel Prizes). The fundamental nature of these materials is astounding—including how
the electrons “group” into novel phases such as stripes and nematic states, and how conducting surface states can be “topologically isolated” from an insulating bulk. That there are so many unsolved quantum materials means that researchers must enable a suite of coordinated computation, growth, and measurement techniques to elucidate these natures. The technological payoffs for energy and quantum information sciences (QIS) are enormous. And for the latter, researchers stress that the material or materials that are finally used for QIS (qubits, sensors, etc.) have not yet been determined, making this a crucial MR area for the United States, especially in light of international competition. This is well described in the 2017 Department of Energy (DOE) report Opportunities for Basic Research for Next Generation Quantum Systems and in the 2018 NSF report Midscale Instrumentation for Quantum Materials.24
3.4.1 Superconductors
Superconductivity continues to be a fertile field of discovery with the ultimate grand challenge being the discovery of room-temperature superconductivity. The move of high-Tc superconductivity from being a “phenomenon” to a useful physical effect in a variety of copper oxide materials in the late 1980s and early 1990s has been transformational in extending superconductivity from a low-temperature physics phenomenon to a potentially useful applied technology.25 Superconductors with sufficiently high transition temperatures and sufficiently large current-carrying capacity are being used in the form of coated conductors in the electricity grid, for superconducting magnetic energy storage, as turbine/motor components for enhanced energy efficiency, as transformers with less than half the weight of traditional transformers,26 and as quantum sensors as well as spintronics device elements in a number of emerging technologies. Nevertheless, a predictive understanding of high-Tc superconductivity currently remains beyond our grasp as a scientific grand challenge, something that will put computational efforts to the forefront in order to solve.
Over the past decade, superconductivity has been found in unexpected regimes, from a growing number of families of iron-based superconductors to reports
___________________
24 U.S. Department of Energy, 2018, Report of the Basic Energy Sciences Roundtable on Opportunities for Basic Research for Next-Generation Quantum Systems, https://science.energy.gov/~/media/bes/pdf/reports/2018/Quantum_systems.pdf.
25 U.S. Department of Energy, 2016, Basic Research Needs for Superconductivity: Report on the Basic Energy Sciences Workshop on Superconductivity, https://science.energy.gov/~/media/bes/pdf/reports/files/Basic_Research_Needs_for_Superconductivity_rpt.pdf.
26 See M. Noe, 2016, “ESAS Summer School on High Temperature Superconductor Technology for Sustainable Energy and Transport Systems,” http://www.die.ing.unibo.it/pers/morandi/didattica/Temporary-ESAS-summer-school-Bologna-2016/Noe.pdf.
of remarkably high Tc in hydrogen-rich superconductors at the extremes of high pressure. These “surprise” discoveries serve to reinforce how little researchers actually know about the origins of superconductivity. Further, superconductivity is just one of a variety of quantum matter phenomena in which novel ground states give rise to surprisingly useful materials. The enhanced integration of theory, experiment, and synthesis is accelerating progress toward understanding superconducting phenomena. Additionally, the development of new capabilities to understand novel superconductors is impacting a variety of related fields. Nevertheless, a predictive understanding of cuprate superconductivity remains elusive, and the means by which low-energy tuning of coupled and competing interactions yields new states of matter is at best only partially understood.
U.S. researchers are making discoveries more quickly than in the past,27 and a general theme of new physics through new materials remains robust. However, international colleagues are being more effective at sustained investment at scale (see Chapter 5), especially when it comes to synthesis science,28 and this is creating a discovery imbalance (e.g., as measured by the number of new superconductors being discovered domestically versus internationally). As researchers look to the future, the opportunity is beyond “just” new materials discovery/single crystal growth and includes hierarchical structures/functional assemblies that eventually can be economically scaled to kilometer lengths. To seize this opportunity in a timely way, focus could be on coupled theoretical, computational, and experimental tools to predict novel materials and functional architectures, which is a path forward not only for superconductivity but also for all areas of materials research. Further, the role of coherence and topological protection is displacing low-energy tuning as a central focus of understanding quantum matter. Quantum matter, especially as it relates to QIS more broadly, has the opportunity to be a key research area for the next decade, and the discovery and understanding of novel superconductivity has the possibility to continue to drive this field.
3.4.2 Magnetic Materials
Classical studies of magnetism will always have a place in materials characterization. It is inherently part of the study of the properties of any newly synthesized compounds that contains atoms with unfilled d- or f-shells. It is therefore reasonable to expect progress in the discovery of new types of magnetic alignments,
___________________
27 M. Kremer, 1993, Population growth and technological change: One million B.C. to 1990, Quarterly Journal of Economics 108(3):681-716, https://doi.org/10.2307/2118405.
28 See European Commission, 2008, A More Research-Intensive and Integrated European Research Area: Science, Technology and Competitiveness Key Figures Report 2008/2009, http://ec.europa.eu/research/era/pdf/key-figures-report2008-2009_en.pdf.
of magnetic exchange (the energy involved in the magnetic exchange between neighboring atoms), of ordering temperatures (Curie or Neel) that are out of the ordinary range, and of low-dimensional effects (recent developments here include Cu-salts with spin-ladder and spin-chain structures). By combining electronic structure calculations and other numerical models with advanced materials synthesis methods, novel magnetic materials have been designed and demonstrated. Sustained efforts using these techniques in synergy will yield additional advances as other materials and structures are explored. This is the type of progress that MGI has yielded and will continue to yield. Research directions that will receive new emphasis could be the following.
Antiferromagnets
It is worth pointing out that antiferromagnets generally are more prone than ferromagnets to developing complex and rich ordered structures. Triangular lattices can result in magnetic frustration, which in turn can lead to dynamical effects (see below). Many more antiferromagnets than ferromagnets are electrically insulating, which makes it possible to study the ordering in the absence of itinerant electrons. The large variety of possible antiferromagnetic ordering schemes also increases the likelihood that unexpected phenomena will be discovered and identified in the future, with the potential to develop applications that cannot be anticipated today. The antiferromagnetic resonant frequency lies in the 100 GHz range or above, an order of magnitude higher than the ferromagnetic resonance, offering the possibility of developing much faster spintronic switches and memories. The drawback is that, because they have little or no net moment, external magnetic fields do not couple very readily to the magnetic sublattices of antiferromagnets. This complicates the experimental side of research on antiferromagnets and requires investment in instrumentation beyond magnetometers (such as spin-sensitive optical methods and neutron diffraction).
Spin-Dynamic Effects
The research emphasis on magnetism shifted some 20 years ago toward spin-dynamic effects; the past decade focused on linear spin transport. It is reasonable to extrapolate these developments into future discoveries in the field of nonlinear transport. Under strong-enough driving forces, new collective spin modes are predicted to develop, some of them labeled “magnon Bose-Einstein condensates.” While nanopillar spin-based oscillators are nonlinear devices, it is likely that the condensates can give rise to new mechanisms for auto-oscillations. Another promising possibility is that of magnonic logic and memory, where classical electronic functions will be realized in magnonic spin transport in ferromagnetic or
antiferromagnetic insulators. While magnon transport is not dissipation-less, one can hope that this approach can reduce the Joule losses associated with charge-based electronics. These fields will expand to include antiferromagnets. Because there are many more antiferromagnets, particularly among nonmetals, than ferromagnets, and because of the diversity in possible ordering structures, they promise to be a particularly fertile area for future discoveries in spin dynamics. While the field of spin dynamics is very much theory-driven, ultrafast optical probes of spin dynamics, alongside the spin-Hall and inverse spin-Hall effects, are new tools that will result in experimental progress in this field.
Antisymmetric Exchange Interactions
It is likely that the field of antisymmetric exchange interactions will expand beyond the concept of skyrmions. Because of the similarity with chiral electronic structures (such as topological insulators and Weyl semimetals), it is reasonable to expect important new physics and applications to arise from research on noncollinear spin textures in both real space and reciprocal space in general.
Multiferroics
The large response of many complex materials to electromagnetic radiation raises the possibility of ultrafast control of those materials properties through the application of short, intense photon pulses. This new field of materials research is showing promise in a number of areas including especially strongly correlated electron materials. One example of this is multiferroic materials, which show promising potential applications in which magnetic order is controlled by electric fields. However, the underlying physics and ultimate speed of magnetoelectric coupling remains largely unexplored. As shown in Figure 3.1, using ultrafast resonant X-ray diffraction revealed the spin dynamics in multiferroic TbMnO3 coherently driven by an intense few-cycle terahertz light pulse tuned to resonance with an electromagnon mode.29 The results show that atomic-scale magnetic structures can be directly manipulated with an electric field of light on a subpicosecond time scale.
3.4.3 Two-Dimensional Materials
The isolation of graphene in 2004 from graphite was a defining moment that gave “birth” to the modern field of 2D materials. There continues to be intense
___________________
29 T. Kubacka, J.A. Johnson, M.C. Hoffmann, C. Vicario, S. De Jong, P. Beaud, S. Grübel, et al., 2014, Large-amplitude spin dynamics driven by a THz pulse in resonance with an electromagnon, Science 343(6177):1333-1336.
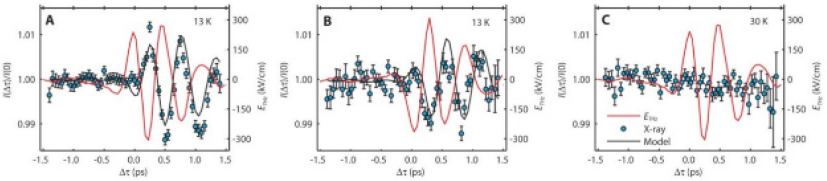
activity in the study and commercialization of graphene. However, challenges persist in achieving commercial graphene devices. Materials limitations include the inability to consistently achieve large-scale growth and processing of uniform materials, as well as the difficulty to achieve good electrical, thermal, and mechanical contact with other materials. While chemical exfoliation can be inexpensive, it is difficult to minimize defects and control layer number; on the other hand, the cleaner chemical vapor deposition growth using metal catalysts is relatively expensive. It has also been difficult to make small devices that maintain the properties of larger areas, because of issues such as edge scattering. Intrinsic properties such as lack of a bandgap and poor out-of-plane thermal conductivity limit some applications.
In 2011, a high-performance single-layer MoS2 transistor created excitement over the use of transition metal dichalcogenides in beyond complementary metal-oxide semiconductor devices, as graphene had lacked a natural bandgap. In a short time span since then, the field has gone “beyond graphene.” Opportunities abound, as well as needs from the capability of creating large-scale defect-free materials to enhancing researchers’ ability to probe the properties and defects of a variety of single-layer materials. Researchers often acquire these 2D materials via inorganic approaches such as the formation of molydenite via the direct sulfidation of elemental molybdenum. While synthetic approaches like these have allowed researchers to make great progress in understanding the fundamental physics of 2D materials and confirm theoretical predictions, there must be a shift in emphasis in order to realize many of the promised applications. This next decade must incentivize chemists, material scientists, and chemical engineers to develop robust, low-temperature synthetic approaches that create high-quality “beyond graphene” 2D materials. Without practical deposition techniques that provide high-quality 2D materials
at controlled film thicknesses and of comparable quality to their bulk inorganic films, many of these materials will remain a lab curiosity into the foreseeable future. Center(s) of excellence, such as the NSF-funded Center for Two-Dimensional and Layered Materials30 are starting to make inroads into this formidable challenge.
Push to Understand Heterostructures and Interfacial Behavior
Two-dimensional materials and layered combinations of different 2D materials (or van der Waals heterostructures) can possess unique properties that make them highly attractive for electronic and optical applications. The design and development of low-dimensional material platforms based on the controlled assembly of atomically thin structures will allow researchers to explore novel physical phenomena induced by quantum coherent electronic states. Once again, the controlled growth of high-quality 2D materials and their multilayered heterostructures remains a significant challenge. Pursuing additional development of chemical vapor deposition and atomic layer deposition processes such as molecular beam epitaxy and pulsed laser deposition to synthesize these 2D material combinations is critical.
Some 2D materials are sensitive to oxidation and must be sealed to preserve their structure and unique properties. Understanding how the interfaces of these van der Waals materials interact in heterostructures and surroundings and with impurities of varying type is important for understanding how to encapsulate them (if required), reliably make electrical contact and measure accurate bandgaps, and, finally, how to unlock their full potential. Computation has a special role in this regard, as there is a rapidly growing body of simulations about 2D materials, their fundamental properties, and anticipated heterostructure properties.
Energy Storage, Electrical Interconnects, and Mechanical Devices
Devices such as super capacitors, fuel cells, and rechargeable batteries are seeing an increased usage of 2D materials and should witness significant growth in the next decade. R&D to lower the manufacturing cost and time would accelerate their implementation.
2D or 2D-metal hybrid interconnect structures have seen some initial exploration. Advantages include high mobility and thermal conductivity. Roadblocks include the lack of large-area deposition techniques suitable for their application to high-volume manufacturing, a high out-of-plane contact resistance, and difficulties in controlling multilayer films with suitable electrical properties (mobility, carrier
___________________
30 See Penn State Materials Research Institute, “The Center for 2-Dimensional & Layered Materials,” https://www.mri.psu.edu/mri/facilities-and-centers/2dlm, accessed September 22, 2018.
density, resistivity, etc.). While novel approaches have been proposed to address these challenges, many of the concepts are still in their infancy.31
2D materials are leading candidates for next-generation nanomechanical devices. The in-plane covalent bonds and lack of dangling bonds on the van der Waals surfaces lead to high Young’s modulus and breaking strains, chemically inert surfaces, and low friction between layers. Like mechanically robust molecular analogs of plastic wrap or pieces of paper, monolayers of 2D materials can be manipulated, transferred, and stretched to form heterostructures, 3D architecture, or suspended membranes. In addition, the coupling between the mechanical and other physical properties (thermal, electronic, optical) is of great interest in exploring novel applications. Areas of fundamental mechanical interactions still need to be explored, including the mechanics of interfaces (adhesion and friction) for heterostructured and integrated devices.
3.4.4 Topological Materials
The development of materials whose electronic, optical, magnetic, and even structural properties are controlled by topology has changed our understanding of fundamental materials physics, and will guide the search of new physical phenomena for the next several decades. For example, semiconductor, semimetal, and superconductor heterostructures with signatures of topologically protected transport of electrons, Cooper pairs, and a host of new emergent quasiparticles are being discovered as the electronic properties of these materials are probed.
Utilizing topological properties may lead to a host of applications. For example, the lack of backscattering may offer new opportunities for dissipation-free nano-electronic devices, while the helical spin properties provide an opportunity for spintronics devices. Further devices, such as ultrafast inductors and fault-tolerant quantum computing elements, become possible when the topological materials are coupled to systems such as ferromagnets or superconductors. It should be noted that topological effects typically occur without requiring extreme conditions such as low temperatures or high magnetic fields. Mechanical metamaterials are also a new possibly important direction of research; these are engineered materials whose structures give them novel mechanical properties, including negative Poisson’s ratios, negative compressibilities, and phononic bandgaps. Of particular interest are systems near the point of mechanical instability, which recently have been shown to distribute force and motion in robust ways determined by a nontrivial topological
___________________
31 Semiconductor Industry Association and Semiconductor Research Corporation, 2017, Semiconductor Research Opportunities: An Industry Vision and Guide March, https://eps.ieee.org/images/files/Roadmap/SIA-SRC-Vision-Report-3.30.17.pdf.
state.32 The field of topological materials has been unique in that is has been largely theory driven, with experiments following; however, it could still benefit from more realistic modeling of spectral and transport properties.
3.5 POLYMERS, BIOMATERIALS, AND OTHER SOFT MATTER
This section addresses materials that are predominantly, although not exclusively, organic in nature. It is a huge swath of the materials domain, rich in both fundamental science questions and in important applications for commerce and society. The three elements of the title of this section are strongly overlapping, in that polymers are the largest and most economically significant class of soft materials and are also dominant in the field of biomaterials. Therefore, it is reasonable and valuable to discuss these elements in concert.
3.5.1 Polymers
Polymer materials are ubiquitous in our daily lives. The past century has seen significant growth in the large-scale production of polymers such as nylons, polyesters, polyolefins, and others. The products that were enabled by and rely on these materials range from commodity textiles to biomedical devices and implants. These materials play a significant role in meeting the grand challenges facing global society.
Polymer science and engineering research and the plastics industry are intimately connected. From biomedical technology, to electronics and communications, to structural materials, to commodity products, polymers (plastics) are everywhere. As researchers look to the future and think about significant societal needs, they need to think about the challenges and opportunities for polymer science and engineering. Application areas of importance to society where polymers are expected to have a significant role include environmental impact, energy and natural resource applications, communications and information, and health. Several promising specific lines of work can be identified in each of these application areas.
- Environmental impact: The objectives in this area should be to use both feedstocks and finished polymer products in an efficient and sustainable way. Some specific directions of particular interest or promise are as follows: (1) exploitation of neglected raw materials (such as waste products from agricultural, industrial or human waste, or other carbon- or
___________________
32 D. Zeb Rocklin, S. Zhou, K. Sun, and X. Mao, 2017, Transformable topological mechanical metamaterials, Nature Communications 8:14201.
-
silicon-containing substances such as carbon dioxide (CO2) and limestone sand) to form useful polymeric materials; (2) capitalization on the past decade’s progress in self-healing materials to enhance lifetime, durability, and recycling (currently 14 percent for plastic packaging versus 70-90 percent for iron and steel); and (3) separation or other physical processes to enable recycling of mixed plastics.
- Energy and natural resource applications: Polymers are a notable player in renewable energy processes such as wind, solar, and fuel cells. Advances in polymer technology can improve the efficiency of these processes, reduce their cost, and increase capacity. Some specific directions of particular interest or promise are as follows: (1) improved safety and efficiency of energy storage systems, including solid electrolytes, all-organic batteries, and redox polymers for flow batteries; (2) polymers for energy conversion applications, including organic photovoltaics and light-emitting diodes (LEDs), and thin film transistors, thermoelectrics, leading to flexible and wearable systems; (3) polymers for the energy-water nexus, such as membranes and antibiofouling materials; (4) smart construction materials that improve energy efficiency and transport clean water; and (5) implementation and integration of the principles of green chemistry and engineering and life cycle/sustainability thinking into the design and development of commodity and advanced polymer technologies.
- Communications and information: Communications and information technology is a key area where polymers play a critical role. In addition to providing the foundation for packaging of communications and electronics technologies and the protective coatings for fiber optics cabling, polymers also play a significant role as active materials. Some specific directions of particular interest or promise are as follows: (1) in polymer and organic semiconductors, issues that remain to be addressed include relatively low charge carrier mobility, which limits charge transport in devices; weak oxidation stability and short working lifetime owing to destabilized highest occupied molecular orbital and lowest unoccupied molecular orbital energy levels; and a fairly high bandgap that limits absorption of available sunlight; (2) design and development of semiconducting organic and polymer materials for optoelectronic applications requires consideration of structure-property-process relationships; and (3) the development and implementation of generic data repositories to facilitate all researchers’ access to raw data. These repositories can then be mined and analyzed to more rapidly advance the field.
- Health: Biomedical materials are playing a significant role in the health enterprise, and many of the advances that researchers have seen in recent years have been enabled by polymers. Polymers will continue to be critical to improving human health. Researchers need cost-effective,
innovative materials to control, treat, and diagnose infectious diseases. Further, as implant technologies continue to be developed, along with drug-delivery systems and multifunctional biosensors, researchers need a better understanding of how polymers react in dynamic and in some ways harsh environments in the body. These fundamental insights will open the door to new biomedical polymer-based technologies. Some specific directions of particular interest or promise are as follows: (1) expanding the design of polymer-based nanomaterials from tissue regeneration and drug delivery into new applications such as immuno-engineering; (2) AM of devices and scaffolds leading to better control of nano- and microstructure as well as the possibility for made-to-order, one-off, on-the-spot construction of devices and implants; and (3) development of polymer-based tissue engineering to minimize the use of animal models in drug and materials testing.
An August 2016 NSF workshop, “Frontiers in Polymer Science and Engineering” (co-sponsored by the Air Force Research Laboratory/Air Force Office of Scientific Research, Army Research Office, DOE/Basic Energy Sciences (BES), Food and Drug Administration, National Institute of Standards and Technology, and Office of Naval Research), “focused on the most promising directions for research associated with macromolecular materials,”33 and it affirmed many of these application directions. In addition to applications, the opportunities for fundamental advances in basic polymer science are enormous. Overarching opportunities include the following:
- Research that connects synthesis, structural control, property characterization, and dynamic response across many length scales. This opportunity touches instrumentation, theory, and computation with feedback into polymer synthesis. The most exciting opportunities will connect subdisciplines and length scales.
- Advanced instrumentation that is created and aggregated so that facilities and capabilities that may be out of reach of individual institutions, from synchrotron and neutron scattering down to electron microscopy, nuclear magnetic resonance, and rheology, are readily accessible.
- Ubiquitous theory and computation should break down barriers between theoretically focused and experimentally rooted investigators through investment in collaborative initiatives that exploit both sets of skills. This is the application of the MGI to polymer science.
___________________
33 National Science Foundation, 2017, Frontiers in Polymer Science and Engineering: Report of a 2016 NSF Workshop (Co-sponsored by AFRL/AFOSR, ARO, DOE/BES, FDA, NIST, ONR), University of Minnesota Printing Services, Minneapolis, MN, p. vi.
- A key opportunity is the development of accessible, scalable polymers that match or exceed the property matrix of existing materials while having a greener life cycle.
Macromolecular science starts with synthesis. As in other areas of MR, there is a focus in polymer science on opportunities in precision synthesis. Better control over sequence, composition, tacticity, branching, architecture, topology, dimensionality, functionality, and dispersity is needed. Techniques that are leading to near-biological precision in sequence control represent exciting opportunities in a basic science sense, although an equally exciting opportunity is to demonstrate what new properties can be achieved with this level of precision synthesis. Synthesis is also where considerations of other opportunities start. Green polymer chemistry can create strategies that improve recyclability, reduce environmental effects, and control the durability of polymers.
Polymer materials synthesis includes higher-order structure formation and supramolecular assembly for in situ access to complex nano-objects and polymerize preorganized monomers to create programmed architectures. Noncovalent bonding, including mechanical interlocking via catenanes and rotaxanes, is as yet underexploited in creating higher-order structures. Not only should higher-order assembly processes be explored for the distinctive structures that they can form, but also it should be recognized that self-assembly, and other higher-order assembly processes, while thermodynamically spontaneous, are not instantaneous, and therefore, study of the kinetics of these assembly processes is needed. To quote the NSF workshop report, “We must begin to think of assembly not as a process with fixed thermodynamic states defined by equilibrium but rather as a pathway with useful intermediates that yields a richer and more complex phase space. Assembly can be designed in a manner similar to a chemical reaction path, where many unit operations simultaneously take advantage of the kinetics and thermodynamics of assembly.”34
Like many areas of materials science, polymer materials science is now fueled significantly by data science. There is a need for accessible and well-managed cyberinfrastructure that facilitates and encourages the storage, organization, curation, retrieval, and effective use of experimental and computational data on polymer properties. First-principles prediction is the ultimate opportunity. Real-time acquisition, and, more importantly, interpretation, is an opportunity to accelerate integration of experiment with analysis. This opportunity is pronounced when the arena is more in the realm of polymer processing than is polymer equilibrium structure. Polymer processing frequently employs far-from-equilibrium conditions and nonlinear deformations such that the design of processing methods to control polymer properties is often accomplished by good intuition and empirical
___________________
34 Ibid., p. 8.
findings. Although equilibrium and linearity have long been useful bases, the future opportunities are in understanding dynamic states. Polymer materials have unique problems in these areas, especially in correlating processing conditions to polymer structure, most notably in semicrystalline polymers. A particular example is that the physics of failure in polymeric materials is poorly understood.
3.5.2 Biomaterials and Bio-Inspired Materials
Future directions for the broader objective of deepening our mastery of biomolecular materials science will require integration of advanced synthesis, novel characterization tools, and computation. Advances in polymer science will be critical in developing this frontier given the essential role of covalent macromolecules in cell components as well as plant and animal extracellular matrices.
There are many possible directions for the next decade ranging from autonomous behavior in soft matter to mastering the creation of synthetic materials with comparable properties and functions to those of musculoskeletal tissues. In the latter objective, it is critical to learn how to encode in molecules the formation of hierarchical structures using multiple components over a broad range of molecular weights. The opportunities in autonomous behavior could focus on rapid time scales in actuation and motion that are molecularly encoded in soft materials. Related objectives could search for materials with dynamic mechanical properties that originate in materials with capacity to reconfigure their bonding configurations or undergo the type of reversible self-assembly that operates in the cytoskeleton.
The enabling strategies for critical advances in biomolecular soft matter are many, and some of them may not be easily envisioned at the time this report was published. However, some of them are suggested based on initial work that has been recently reported. One such strategy described earlier as an emerging area just barely initiated in this past decade is the rational integration of supramolecular phases with covalent polymers. These systems were described earlier in this report as hybrid-bonding polymers—materials that could generate properties not previously observed when covalent polymers and supramolecular polymers are rationally integrated. In the past 10 years, there are examples of ordered membranes formed through self-assembly composed of such hybrid structures. In another example, simultaneous covalent and supramolecular polymerizations have yielded materials encoded for chemical regeneration. Given advances over the past decade on DNA nanotechnology, it will be important to explore whether robust and scalable chemistries with the interactive fidelity of nucleic acids can be developed in fully synthetic systems. A key objective in this area would also be a practical protein synthesis technique. While DNA is easily produced to order, proteins are not. A true “made to order” protein synthesis method would be a breakthrough in the ability to produce bio-inspired and biomolecular materials and devices. It would also make
an enormous impact on the ability to develop devices based on synthetic proteins including any based on nonbiological amino acids. Last, an important enabling strategy is to understand energy landscapes in biomolecular soft materials, a field that was just barely started at the end of the past decade.
Research in the next decade in the area of biomaterials is important not only because of its direct impact on biomedical technologies but also because basic and translational research in this area will lead us to materials innovation through bio-inspired materials. The biomedical side is critical given the growth of aging populations and society’s strong interest in high quality of life. The great opportunities in bio-inspired materials will be catalyzed by advances in biomaterials, since the latter will be designed to interact directly with living organisms and this will require emulating the materials of life. Historically, the field of biomaterials for nearly three-quarters of a century has borrowed for its needs materials developed for technology at large. Excellent examples include metals with high corrosion resistance and polymers developed for textiles and consumer goods. As researchers begin the next decade, this process is inverting, and the “new biomaterials” may in turn inspire development of materials with capabilities observed in “living matter” and produced for use outside the body. This could involve highly dynamic materials that adapt to environments by changing their properties, materials that self-repair, or materials with “robotic” character that actuate or move to perform useful tasks. Other efforts such as the National Institutes of Health (NIH)-led Tissue Chip Initiative35 is developing human tissue chips that accurately model the structure and function of human organs for improved drug screening, pushing the frontier between semiconductor technology and soft matter.
One important opportunity in inorganic biomaterials is further work on metallic materials composed of biometals that could not only resorb after implantation but also deliver metal ions for a bioactive function. Work has already been pursued on the use of magnesium, but other metals such as zinc and iron-based alloys should be explored further. This work could also have impact on discoveries relevant to biodegradable electronics to be implanted in living tissues or designed for other applications. A direction of value would be to create biomaterials or materials for other functions in which biometals are integrated with soft materials in the form of composites. Similar opportunities exist with ceramic biomaterials. In the case of biomaterials, this would offer more options to match mechanical properties of implants with those of tissues while retaining capacity for full resorption and even bioactivity. For materials at large, such combinations would lead to better options for recycling or nontoxic biosensors and wearable components. The expansion of capabilities for AM with metallic materials also greatly expands the possibilities for on-the-spot fabrication of metallic biomedical parts.
___________________
35 See National Institutes of Health, “Tissue Chip Initiatives & Projects.” https://ncats.nih.gov/tissuechip/projects, accessed July 21, 2018.
A different opportunity with metals or ceramics that would integrate functions in biomaterials or other materials is the development of strategies to create nanoscale topographies on hard materials. In this area, there are possibilities with inspiration from topographies observed in living organisms that would impact on interfacial friction and adhesion among other properties. In ceramic biomaterials, there is also the possibility to create biocompatible piezoelectric apatite-based compositions, possibly through computationally guided design, that could electrically stimulate regeneration of tissues. Again, any developments along these lines are likely to find applications in areas outside of biomaterials.
The use of AM methodologies involving sintering, melting, or soft-hard hybrid inks should continue to expand the functionality of metals and ceramics as biomaterials and other applications as well. Precision in the internal structure of implants could be used to rationally guide blood vessel and tissue ingrowth, and perhaps even antimicrobial functionality as the resolution begins to improve in AM and more is understood about bacterial colonization of implants. AM using inorganic powders could have great impact in many technologies, but in the context of biomaterials it would be particularly important in the expanding areas of orthopedics, cardiovascular medicine, dental implants, and generally on fabrication of biomedical devices.
Soft biomaterials are the materials of choice to promote regeneration, partly because they can emulate the structures and mechanical properties of soft tissues and, when combined with inorganic components, those of hard tissues such as bones and teeth. Furthermore, they can be designed through self-assembly strategies with the biomolecular structures present in cell components and their extracellular matrices. Soft biomaterials have traditionally been composed of polymers, and opportunities in this area will continue to develop with advances in polymer science that are described elsewhere in this report. However, the past decade established the many opportunities available in supramolecular soft biomaterials in which self-assembling “bioactive” molecules are used as components in order to signal cells directly and trigger biological events.
In this context, an important scientific investment for the next decade is to contemplate the development of biomolecular materials with ability to signal cells dynamically and autonomously. This implies ability to turn off signaling when no longer needed, or change signals to guide sequential steps of a biological process. This capability would be important in the use of biomaterials to manage expansion and transformations of stem cells for regenerative therapies. Equally important would be the use of biomaterials to drive dedifferentiation of a given type of cell, followed by differentiation into a different lineage, as is done in induced pluripotency stem cells. An important type of dynamic behavior researchers do not currently know how to design for is the continuous assembly and disassembly of components for temporal changes in physical properties. Recent breakthroughs have shown that incorporation of DNA chemistry in soft biomaterials (see Figure 3.2) could help

craft these behaviors using molecular displacements in double helices.36 Related mechanisms could also be developed to trigger changes in mechanical properties in biomaterials in order to mediate the surprising phenomena uncovered by the field of mechanobiology. Also, an important research direction in the field of bioactive biomaterials for the next decade is glycochemistry, since great challenges are still faced in our ability to produce synthetic glycans.
In the next decade, effective development of soft biomaterials will require advances in our ability to achieve higher levels of structural precision not currently possible. Both chemical and physical strategies are needed to control nanoscale features in supramolecular assemblies and at the other extreme hierarchical structures across the scales. In this regard, it will be important to learn more about the energy landscapes of assemblies used to create soft biomaterials in order to select the right pathways for their synthesis. Possibly the most important opportunities lie in structural control in supramolecular assemblies on scales that are much smaller
___________________
36 R. Freeman, N. Stephanopoulos, Z. Alavarez, J.A. Lewis, S. Sur, C.M. Serrano, J. Boekhoven, S. Lee, and S.I. Stupp, 2017, Instructing cells with programmable peptide DNA hybrids, Nature Communications 8:15982, doi:10.1038/ncomms15982.
than their overall dimensions—for example, the details of molecular self-sorting at the scale of nanometers in micron-long one-dimensional assemblies such as nanofibers. Another important component will be the merger of opportunities offered by advances in polymer science, described elsewhere in this report, with supramolecular materials that rely on self-assembly processes among molecules without formation of covalent bonds. An important opportunity from polymer science in this context will be to establish whether meaningful functions can arise from the long-sought objective of achieving molecular precision in synthetic polymers.
The combination of structural control and dynamic behavior in soft biomaterials is important not only in the use of biomaterials for regeneration and drug delivery that dominated the past decade but also to take advantage of new materials in important applications such as development of organoids for biological research, gene editing, microbiome control, modification of decellularized organs for transplantation, and development of organs on a chip to understand disease and create new therapies, among others. Since soft biomaterials commonly take the form of hydrogels, further advances will also require research on the organization and dynamics of water in these materials as an essential feature of their physical properties and ability to signal cells.
Expanding the potential of supramolecular biomaterials for biomedical functions will benefit from advances in our understanding and control of supramolecular dynamics. This would be important in their ability to react to biological environments, causing structural reconfigurations that change or optimize their function. Another important direction is to learn how to master precise spatial positioning of multiple biological signals within nanostructures. This area will advance as better imaging techniques are developed, and a deeper understanding of intermolecular interactions is achieved. A third needed direction is research to advance synthetic chemistry in order to integrate potent biological signals using not only peptides, which have been more common so far, but also nucleic acids and glycans.
3.5.3 Soft Matter and Granular Materials
Colloidal self-assembly will become ever more sophisticated, holding out the hope, for example, that it will eventually lead to self-replication, more efficient manufacturing processes, and evolvable materials. DNA will continue to be used to construct building blocks that will enable self-assembly of more complicated and more useful materials. It will also be used to build model systems that will enhance our understanding of natural systems. For example, nanometer-scale cross-linkers with three or more arms designed to connect to different types of linear polymers and with controlled angular stiffness for different pairs of arms could give lead to the creation of new types of polymer networks with tailored mechanical or chemical properties. Protein nanotechnology with natural and artificially designed
polypeptides is a growing area that will complement and compete with the more well-established DNA nanotechnology. Foldable and deformable emulsion and colloidal constructs will bring some of this technology to the micrometer scale. All of these developments provide opportunities for breakthrough advances.
The near future offers continued opportunities to create new colloidal particles with bespoke shape, size, and surface structure and chemistry to control local interparticle interactions and to react to and report chemical or physical changes in their host fluid or in other particles. These made-to-order particles will open new opportunities to control self-assembly, possibly leading to more efficient manufacturing or to evolvable materials. They will improve and broaden our understanding of the general phenomenon of jamming. The increased library of particle shapes and interaction offers opportunities to explore the self-assembly of possibly large particle clusters that respond only if the clusters are in a particular configuration or that exhibit the controlled allosteric response, in which forces between specified particles induce displacements between pairs of other specified, sometimes distant particles, which has already been demonstrated in random frames.37 There is much to be learned about colloidal systems (and the systems they model) that are driven into nonlinear regimes or at faster time scales. Investigation of these and related phenomena will benefit from the development of new techniques that provide simultaneous, high-speed, 3D measurements of particle positions and local stresses.
In a trend that is already well under way, research can be expected at the boundaries between different traditional fields, running the gamut from the most fundamental to the most applied. There are opportunities for further advances in the manipulation of liquid crystalline elastomers with various external agents—light, pH, heat—with applications like artificial cilia or movable parts in soft robots. Research will open the door to increased use of liquid crystals in architectural settings such as glass walls with changeable color and opacity38 and for digital optical applications like digital eyeglasses, switchable contact lenses, and virtual reality glasses, and even to the prevention of counterfeiting.39
There are many opportunities for research on nanometer-scale inclusions in liquid crystals. For example, surfactant-coated nanoparticles segregate on the surface of chiral, cholesteric liquid crystals vesicles and can form patterns following
___________________
37 J.W. Rocks, N. Pashine, I. Bischofberger, C.P. Goodrich, A.J. Liu, and S.R. Nagel, 2017, Designing allostery-inspired response in mechanical networks, Proceedings of the National Academy of Sciences U.S.A. 114(10):2520-2525.
38 Merck KGaA, 2017, “Liquid Crystals for New Optical Applications: Collaboration with the University of Leeds,” https://www.emdgroup.com/en/news/liquid-crystals-leeds-cooperation-18-05-2017.html.
39 University of Luxembourg, 2018, “From TV to Tactile Robots: The Future of Liquid Crystals,” press release, May 30, https://www.technologynetworks.com/informatics/news.
those of the underlying cholesteric,40 and gold nanorods segregate in nanometer-scale cores of disclination lines in nematics liquid crystals, giving rise to surface plasmon resonances tuned by the nanorod concentration.41 These represent a developing trend in liquid crystals to obtain better control over 3D conformations of their anisotropy, layering, and defect structure, often through various surface patternings. Ferronematics, which currently are based on alignment of ferronematic nanoplatelets, are in their infancy. Their magnetism provides opportunities for new responses to magnetic fields that could be used in applications. Better control of surface chemistry will allow templating of surface boundary conditions at shorter length scales.
Opportunities in active matter are almost unlimited. They start with the creation of new micro- and nanoscale particles preprogrammed with specific properties like the ability to move, manipulate flows of matter and energy, or transform one form of energy to another, and then continue to the design of new functional media to host the new particles such that the combined system presents new collective behavior. The long-term goals will be to further understand the statistical mechanics of these active systems and, with that understanding, to engineer them to perform useful functions like facilitating self-assembly or enabling soft microrobots that manipulate matter and the flow of energy. This is a highly interdisciplinary field that will require collaborative efforts of materials scientists, physicists, engineers, chemists, applied mathematicians, computer experts, and biologists.
Although it has been under intense study for almost two decades now, there are still many aspects of jamming that researchers do not understand and that open opportunities for future research. There is a long list of questions that can be posed but cannot yet be answered. How do microstructure (i.e., particle shape, roughness, and friction) and intermediate-scale structure (i.e., the geometric arrangement of particles) affect the dynamics of flow and force transmission within a static dry pile? This is still not understood. Will a more detailed understanding of the linear elasticity of piles inform us about catastrophic failure modes such as avalanches? How can the buildup of static electricity in flowing dry grains be controlled?
Soft matter experiments now collect staggering amounts of data—for example, from high-speed videos. Identifying physically relevant features in even one of these videos presents a daunting challenge. In addition, soft matter systems are often so complex that it is often difficult to know what parameters encode the most important physical properties. New techniques like machine learning, evolutionary
___________________
40 L. Tran, H.-N. Kim, N. Li, S. Yang, K.J. Stebe, R.D. Kamien, and M.F. Haase, 2018, Shaping nanoparticle fingerprints at the interface of cholesteric droplets, Science Advances 4(10):eaat8597.
41 E. Lee, Y. Xia, R.C. Ferrier Jr., H.‐N. Kim, M.A. Gharbi, K.J. Stebe, R.D. Kamien, R.J. Composto, and S. Yang, 2016, Fine golden rings: Tunable surface plasmon resonance from assembled nanorods in topological defects of liquid crystals, Advanced Materials 28(14):2731-2736.
algorithms, and network methods are making their appearance, but more needs to be done. Simulations and modeling play an ever-increasing role in soft matter. As in other disciplines in materials science, there are readily available codes, like large-scale atomic/molecular massively parallel simulators for running molecular dynamics simulations, but new codes will be needed to deal with new methods of data analysis. The soft matter community does not have access to large databases of experimental results and N-body simulations that can shorten the time and cost of materials development, and demand for such access in increasing.
3.6 ARCHITECTED AND METAMATERIALS
Artificially structured materials offer tailored materials properties and responses, even beyond those found in nature. They may enable key advances in areas including lightweighting, energy efficiency, optical/thermal/acoustic devices, and imaging. Challenges and opportunities exist in the development of new designs via computation, as well as new techniques to efficiently fabricate at large scales.
3.6.1 Architected Materials
The use of shape in the design of architected materials and mechanical metamaterials has only begun to scratch the surface of what could be done. Lightweighting with architected materials—that is, materials having a designed topological distribution of composite cells—offers increased energy efficiency, payload capability, and life cycle performance as well as increased quality of life via less invasive implants, high-performance prosthetics, and functional exoskeletons. Lightweighting through the design and manufacturing of architected structural materials thus holds great promise for numerous technologies including aerospace, transportation, energy generation, and industries that employ large rotating components. Developing robust methodologies for decoupling and independently optimizing properties would usher in a new wave of multifunctional architected materials—for example, structural materials or skins that provide thermal management, environmental protection, and enhanced communications, sensing, or invisibility. Creation of architected multimaterial systems would open the property space even further and could be used to impart enhanced functionality. Going beyond origami- and kirigami-inspired shape transformations, unidirectional wave guiding, electromagnetic sensing, programmable stiffness, and tailored energy absorption are all possible.
Computer-based topology optimization codes provide a very promising avenue for decoupling and independently optimizing material properties and functionality. Moreover, coupling this numerical approach with digital AM threatens to disrupt current engineering design and manufacturing practices. Full realization of this potential will require the development of topology optimization methods to model
highly complex and nonlinear phenomena, the incorporation of manufacturing constraints into the optimization codes, digital transfer of optimized designs, and cost-efficient AM of the desired architectures with requisite precision and accuracy. Challenges remain in then realizing these designs, particularly in a cost-effective way. Further, manufacturing challenges related to surface roughness, dimensional acuity, property anisotropy, and property homogeneity must be addressed and overcome. Qualification and validation of additively manufactured materials and components is receiving considerable attention, and this problem must be solved to realize both the development of exciting new applications and advancement of the fundamental science to anchor this disruption.
3.6.2 Metamaterials for Photonics, Phononics, and Plasmonics
Metamaterials are a class of structurally architected materials designed to have specific functional (e.g., magnetic, electrical, vibrational, or mechanical) responses that are often not found in nature. These materials offer tremendous opportunity for new photonic, phononic, plasmonic, and electromechanical devices that can be used, for example, for microwave technology, integrated photonic circuits, energy-efficient light sources, lithography, sensing applications, catalysis, thermal engineering, and imaging techniques beyond the diffraction limit. Early work on metamaterials involved 3D arrays of metallic circuits, which produced properties such as negative optical refraction index (useful for subwavelength imaging), cloaking, and superlensing. Future growth potential incudes making nanometer-scale structures for photonic applications, as well as nonlinear designs that control electromagnetic phase-matching conditions, and also designs to produce negative refraction index in nonelectronic materials (e.g., for manipulating elastic and acoustic response). Implementing devices will require the development of technologies such as 3D nanofabrication at large scales. Another challenge with metallic metamaterials is the intrinsic losses from electronic transitions. New opportunities exist in metamaterials made from nonmetals, such as dielectrics, as well as in designing metallic or hybrid systems that minimize the impact of losses. There are also opportunities in designing metamaterials having multifunctional properties—for example, thermal and electrical conductivity for energy efficiency.
3.7 MATERIALS FOR ENERGY, CATALYSIS, AND EXTREME ENVIRONMENTS
3.7.1 Materials for Energy
Energy expenditures correspond to approximately 10 percent of the world’s economic output; annual energy expenditures in the United States have routinely
exceeded $1 trillion/year since 2003 and have varied between 6 and 9 percent of the U.S. gross domestic product over the past 15 years. While energy represents a significant economic activity in its own right, even greater value is associated with its role as the crucial enabler for nearly all transportation, industry, and consumer activities. Therefore, providing reliable and cost-effective energy solutions is a societal and scientific grand challenge. Materials discovery and exploitation will continue to be a central element of any effective energy strategy.
Opportunities for significant advances in research on materials for energy fall into several large categories, including energy conversion, energy storage, energy efficiency, materials for energy, and resource sustainability. Some specific exciting examples of future opportunities have been described earlier in this section. Materials for solar energy conversion to electricity should continue to pay dividends from long-standing work on amorphous silicon and organic photovoltaics, to newer work on perovskites. A plentiful and large-scale solar energy supply will be possible only if an inexpensive storage mechanism is developed. Conversion of solar energy to hydrogen that can be stored and used in fuel cells merits substantial further effort, building on the type of work led by the Harvard “artificial leaf” project. Penetration of solar energy is limited at least as much by needs for energy storage materials as it is by energy conversion materials. The sluggish pace in the development of energy storage materials should be attacked on all fronts from basic electrochemistry to new battery materials to flow batteries and new battery designs.
The opposite direction of energy conversion—namely, electricity to light, in the form of LEDs, both inorganic and organic, and solid-state lighting—also presents opportunities for enormous improvements in energy efficiency. For solid-state lighting to fully realize its enormous potential, the current use of phosphors to produce white light with desirable spectral qualities must eventually give way to mixing multicolor semiconductor electroluminescence in the blue, green, and red. This requires the development of new light-emitting materials.
Energy efficiency is another important area to focus on. There are opportunities to increase energy efficiency by better thermoelectric materials, in which inorganic and organic embodiments are being explored. Although the needed performance characteristics to be effective in, for example, capture and use of waste heat are not there yet, it seems possible that hybrid materials may provide some progress. Extending the use of certain high-performance structural materials to high temperatures can enable better thermodynamic efficiency of processes in some cases. Low-power electronics is another important area for future materials development related to energy efficiency. Power consumption and the resultant heat dissipation is a major obstacle confronting advanced high-performance computing. Neuromorphic computing is a concept developed in the late 1980s, describing the use of VLSI systems containing electronic analog circuits to mimic neurobiological architectures present in the nervous system, which obviously operate with much greater
efficiency. New materials development, particularly materials for resistive switching in contrast to charge-based switching, is key to the development of neuromorphic computing. Energy efficiency in transportation may be enabled by appropriately architected metamaterials leading to lighter weight while retaining high strength.
The inter-relationships between energy and water have recently been recognized by NSF, DOE, and other agencies. For example, the report from the 2017 DOE/BES Basic Research Needs Workshop begins, “Cooling in power plants, refining petroleum, producing fuel, and extracting energy resources from the earth make up a significant fraction of water use. Conversely, water treatment, distribution, and use represent significant energy demands.”42 MR problems and needs abound in this space. Reverse osmosis membrane desalination of seawater operates near maximum possible efficiency, but there are numerous other performance characteristics of membrane separations that could be significantly enhanced such as solute selectivity and resistance to biofouling. Improvements in biofouling resistance could also yield energy efficiency in marine transportation. A 2011 study estimated that biofouling increases the frictional drag on ships so much that it costs the U.S. Navy between $180 million and $260 million per year in added fuel use.43 These are just a few of the important opportunities in MR related to energy and water, contributing further to the richness of work on materials for energy.
3.7.2 Materials for Catalysis
Theoretical predictions for improved catalytic materials have started leading the way toward the design of improved catalysts for specific reactions. Often, composition and architecture of predicted catalysts point toward the synthesis of bimetallic and multicomponent alloys, exposing the preferred crystallographic orientations on their surface. Core-shell nanoparticles with specified number of atomic layers for the shell metal is one such example (see Figure 3.3). These are promising metastable states of matter, capable of performing highly selective catalysis at low temperatures, where stability of the catalyst is not an issue. However, the synthesis of, for instance, shape-selected core-shell nanoparticles as prescribed by quantum mechanics still poses a challenge to inorganic materials synthesis. Furthermore, scalability of the synthetic protocols remains a challenge that needs to be addressed for industrial application of these highly efficient catalysts.
Another challenge for materials synthesis is to achieve the selective deposition of promoter moieties onto the active sites of the catalytic reaction to produce a
___________________
42 See U.S. Department of Energy, 2017, Basic Research Needs for Energy and Water, https://science.energy.gov/~/media/bes/pdf/reports/2017/BRN_Energy_Water_rpt.pdf.
43 C. Winner, 2012, “Barnacles and Biofilms: Could Tiny Predators Help Banish Barnacles?,” Oceanus Magazine, December 5, https://www.whoi.edu/oceanus/feature/barnacles-and-biofilms.
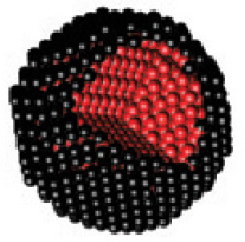
narrow distribution of site architectures, thereby optimizing catalyst performance. Moreover, the synthesis of these materials containing well-defined active sites allows the results from experimental studies to be compared with predictions from theoretical calculations.
The recent interest in 2D materials has rekindled efforts to understand and manipulate the catalytic activity of molybdenum disulfide, a common lubricant, a layered material like graphite with an inert basal plane that has already been exploited in its 3D form as a hydrodesulfurization catalyst. The ability to induce and control reactivity in its 2D form would open many doors for catalyzing new reactions, given the incredibly large surface-to-volume ratio that allows a high density of surface-active sites. These 2D materials also have excellent mechanical properties, high stability, and durability. Their high thermal conductivity could facilitate the conduction and diffusion of heat generated during exothermic reactions, while their high electric conductivity makes them good candidates for electrocatalysis or as electrocatalyst support. Furthermore, the electronic structures of the 2D materials are markedly different from their bulk forms. Given their shape and geometry, multiple ways may be found to further manipulate their electronic structure, with one example being nanoparticles coated with single-layer molybdenum disulfide.
Defects, dopants, hybrid formations, and layered heterostructures are thus among the strategies being pursued to produce not only 2D molybdenum disulfide, but also other 2D transition metal dichalcogenides, such as graphitic carbon nitride, hexagonal boron nitride, as well as graphene. They offer the potential for catalyzing important reactions, such as higher alcohol formation from synthetic gas (formed by a combination of carbon monoxide and hydrogen), oxygen reduction reactions, and hydrogen evolution reactions. Recent work has observed high activity of defect-laden hexagonal boron nitride toward hydrogenation of alkenes to alkanes (see Figure 3.4).

3.7.3 Materials for Extreme Environments
There is growing demand for high-performance materials capable of satisfactory operation under a variety of extreme operating environments.44 These demanding applications include, for example, lightweight, high-strength, and high-toughness materials. For aerospace applications, key issues are ultra-high-temperature materials for hypersonic flight and propulsion systems operating above 2000°C. Additional demanding applications include structural materials and fuel systems for advanced fission or fusion energy systems (capable of extraordinary resistance to high-dose neutron irradiation-induced property degradation while being subjected to high heat fluxes). Yet another set of demanding applications includes corrosive coolants, and high mechanical stress; and high-temperature corrosion-resistant materials to enable ultrasupercritical fossil energy power systems. Additional important niche applications include structural materials with superior resistance to ultra-high-cycle mechanical fatigue, materials for ultrahigh field magnets, semiconductors, and other electronic materials for high-temperature environments, high electric field insulators that are resistant to dielectric breakdown, cryogenic materials with high strength and high toughness, and materials for ultrahigh strain rate (shock wave) environments. In many cases, the known menu of candidate materials is sparse and highlights the critical need for the discovery/design of new materials, the strategies for their processing, and the determination of their foundational thermodynamic properties in extreme conditions. The timely discovery, design, and development of a new generation of high-performance materials would impact multiple advanced technology platforms.
___________________
44 K.T. Faber, T. Asefa, M. Backhaus‐Ricoult, R. Brow, J.Y. Chan, S. Dillon, W.G. Fahrenholtz, et al., 2017, The role of ceramic and glass science research in meeting societal challenges: Report from an NSF‐sponsored workshop, Journal of the American Ceramic Society 100(5):1777-1803.
Building upon research advances achieved in the prior 10 to 15 years, a solid mechanistic understanding is now generally available that allows science-based designs to be proposed for next-generation high-performance materials tailored for optimal performance in specific extreme environments. In particular, growing confidence in the proven value of computational thermodynamic tools to guide identification of the appropriate composition and processing specifications of new materials, paired with improved fundamental understanding of the unit processes that control property degradation in materials, offers the prospect of dramatically shortening the time period to design (see, for example, Section 4.3.1 and the discussion of MGI and ICME), fabricate, and validate the performance behavior of tailored materials. Consequently, there is a good scientific basis to be optimistic regarding the prospects for accelerating the rate of improvement in key metrics such as tensile strength (while retaining adequate ductility and toughness) or resistance to neutron displacement damage property degradation. For example, although there have been steady evolutionary enhancements in the tensile strength of alloys (while retaining adequate ductility), the current best-performing materials are still more than one order of magnitude below the ideal shear strength limit. Leveraging recent improvements in the understanding of temperature-dependent nanoscale deformation mechanisms in materials, it is now conceivable that a new family of alloys could be designed and tested in the coming decade with bulk strengths at the desired operating temperature over a factor of two higher than the current best high-strength commercial materials. Whereas computational modeling of ceramic materials for ultra-high-temperature applications is generally more challenging than in metals, methods based on DFT are now able to calculate mechanical, electronic, and thermophysical properties of some ceramics over a wide range of temperatures, and theory has recently provided a fundamental explanation for the reported maximum in melting point for rock-salt solutions of TaC-HfC. Similarly, improved understanding of the unit radiation defect recombination (“self-healing”) processes in irradiated materials offers realistic optimism that materials resistant to radiation-induced property degradation at energy-relevant elevated temperatures up to displacement damage levels more than twice the current best-performing structural materials (i.e., >500 displacements per atom) are conceivable to be successfully developed during the coming decade. Analogous improvements in scientific understanding of corrosion mechanisms can be leveraged in the coming decade to design new corrosion-resistant materials capable of performance lifetimes up to 10 times longer than current materials.
Recently developed research tools can enable important insight on the behavior of materials at extreme conditions. Laser techniques now permit characterization of melting at temperatures approaching 4000°C (e.g., HfC0.98, at 3959 ±84°C). New approaches also can reveal a material’s strength, heat capacity, thermal expansion, thermal diffusivity, and electrical conductivity at extreme temperatures.
One example is the development of superconducting power cables and how losses are measured by calorimetric methods.45 Measurement of thermodynamic properties at extreme temperatures can be performed using recently developed novel calorimetric techniques (e.g., aerodynamic levitation with laser melting), where containment of materials at these temperatures without introducing contamination or measurement artifacts had been a major practical issue. Phase transformations can be interrogated using X rays coupled with noncontact methods of heating (e.g., quadrupole lamp furnaces), although these are still limited to temperatures below about 2000°C. Oxidation studies up to 2000°C are possible for high-temperature ceramics such as refractory borides by leveraging their metallic conductivity for resistive heating. The combination of diamond anvil cells and high-luminosity synchrotron sources allows high-temperature, high-pressure in situ measurements of structural, thermal, transport, chemical, and even magnetic properties. Collectively, the emergence of these new advanced tools offers significant opportunities to dramatically improve understanding of performance limits and fundamental degradation mechanisms in materials under extreme operating conditions.
Extreme operating conditions represent a crosscutting materials opportunity both in terms of R&D to develop new materials capable of satisfactory performance under extreme conditions and harnessing extreme or nonequilibrium test conditions to obtain new insight on material properties and degradation mechanisms (e.g., phase stability of materials under extreme pressures). This forefront research offers opportunities to couple unique experimental facility research with theory and computational modeling to explore fundamental materials behavior under extreme conditions, validate model predictions and thereby simultaneously improve understanding of materials, and develop new generations of high-performance materials.
3.8 MATERIALS RESEARCH IN WATER, SUSTAINABILITY, AND CLEAN TECHNOLOGIES
Researchers face the task of developing new, integrated materials and smart technology that will satisfy the next generation’s needs, without further jeopardizing Earth. These materials must be durable, aesthetically pleasing, lightweight, modular, and inexpensive, while also being produced in an environmentally conscious manner. The breadth of the range of demands for these materials is considerable. They must encompass construction, infrastructure, and transportation sectors, in order to replace current aging buildings, fundamental facilities, and the other systems required to meet the needs of a rising global population. Sustainable
___________________
45 M. Kalsia, R.S. Dondapati, and P.R. Usurumarti, 2016, AC losses and dielectric losses in high temperature superconducting (HTS) power cables for smart grid applications: A comprehensive review, International Journal of Control Theory and Applications 9(41):309-317.
infrastructure also includes the availability and provision of sufficiently abundant clean water, a stable electrical grid with reduced dependence on fossil fuels that can then fuel more sustainable modes of transportation, and a robust system for recycle and reuse of materials.
CO2 is the highest volume contributor to greenhouse gas (GHG) production, and while it mostly comes from burning fossil fuels, materials research has a major role to play in reducing CO2 emissions.46 The most common building material, concrete, is one of the largest CO2 producers, creating up to 5 percent worldwide of human-made emissions. A 10 percent weight reduction of a fossil-fuel vehicle produces about a 6 percent improvement in fuel economy, which in turn produces about a 3 percent reduction in GHG emission averaged over the standard vehicle population of cars and trucks. Electric cars powered by renewable solar power will have a much lower CO2 footprint, compared to gas- or diesel-powered cars. Between 5 and 7 percent of the energy in a vehicle’s fuel is lost to rolling resistance between the tires and road surface. Innovative materials and devices to reduce or scavenge this dissipated energy could yield important reductions in GHG, comparable to lightweighting. MR for carbon capture47 and storage holds many research opportunities, including the following: solvent, sorbent, and membrane-based carbon capture; new carbon capture materials, such as metal organic frameworks; electrochemical capture; and sequestration with geological materials.
There are many materials problems associated with abundant clean water; a very large fraction of them relate to interfacial materials science phenomena in membranes, sorbents, catalysts, and subsurface geological formations. New materials, new characterization methods, and deployment of new interfacial chemistries are needed. The scientific issues differ between large-scale purification, which might include desalination or waste water reclamation, and remediation, which might include cleaning up contaminated groundwater or oil spills. Box 3.1 describes one such effort, the development of the Oleo Sponge by scientists at DOE’s Argonne National Laboratory. While it is difficult to improve the purification efficiency and operating expenses of current desalination processes simply by creating new membranes, as they operate near thermodynamic limits, it is possible to extend the lifetimes of membranes and reduce the capital expenses of desalination by surface treatments that reduce biofouling, such as grafting layers of zwitterionic polymers. More generally, there is a need and some promising directions for
___________________
46 Intergovernmental Panel on Climate Change, 2014, Climate Change 2014: Synthesis Report, Contribution of Working Groups I, II and III to the Fifth Assessment Report of the Intergovernmental Panel on Climate Change (Core Writing Team, R.K. Pachauri and L.A. Meyer (eds.)), IPCC, Geneva, Switzerland.
47 L. Li, W. Wong-Ng, K. Huang, and L.P. Cook, eds., 2018, Materials and Processes for CO2 Capture, Conversion and Sequestration, Wiley, February; D. Nocera, 2017, Solar fuels and solar chemicals industry, Accounts of Chemical Research 50:616-619, doi:10.1021/acs.accounts.6b00615.
“omniphobic” surfaces.48 Catalytic remediation is also a promising direction, especially for cleaning up industrial contamination of water. The interdependence of water and energy means that science to improve efficiency in providing clean water also has a GHG impact.
Solar and wind energy need to be stored because they are intermittent and diurnal. There are enormous MR opportunities in the pursuit of economically feasible storage of renewable energy. Li-ion batteries have improved in energy density only slowly in their 30-year history. A transformational jump in energy density may come from multivalent ion conductors or new battery material chemistries,
___________________
48 T.-S. Wong, S.H. Kang, S.K.Y. Tang, E.J. Smythe, B.D. Hatton, A. Grinthal, and J. Aizenberg, 2011, Bioinspired self-repairing slippery surfaces with pressure-stable omniphobicity, Nature 477(7365):443-447, doi:10.1038/nature10447.
and must also be achieved with improvements in cycle life, charging, and safety; many of the needed materials simply do not yet exist. Batteries are not the only conceivable answer for large-scale solar energy storage. The conversion of water to H2 and O2 is one of the most practical carbon-neutral schemes to store solar energy on a global scale,49 requiring new, efficient catalytic materials. The solar energy stored by water splitting may be released in the useful form of electricity by a fuel cell. The infrastructure does not currently exist to accommodate a water splitting/fuel cell energy system. The discovery of new materials to store hydrogen at high energy density will greatly accelerate the development of such an infrastructure.
Polymeric materials offer unique opportunities and challenges in the realm of sustainable, clean technologies. Clearly, they contribute powerful assets in diverse areas such as packaging for food preservations, membranes for water purification, and lightweight materials for transportation and infrastructure. But there is much more to do, including the development of new plastics derived from sustainable sources, designed to decompose harmlessly on demand, or to be recycled and re-purposed. Life cycle analysis is especially relevant in the case of recyclable materials such as many polymers.50 Such analyses take into account all aspects of polymer development, production, implementation, and destruction, creating a comprehensive assessment of the effect a polymer has on the environment. In this vein, progress is being made, but much more needs to be done in using highly naturally abundant polymers, such as cellulose, which are difficult to process in useful ways.
Over the past decade, a clear theme of sustainability has emerged in MR. This includes, for example, a reliance on naturally abundant materials in seeking energy solutions as a design constraint. Further, a focus on materials recycling and reuse spanning the entire life cycle of relevant materials has also been recognized, thereby resulting in reduced usage of scarce or critical materials. Last, there is an appreciation that, for example, materials for energy are directly coupled to water utilization and that a systems approach to energy sustainability is essential, including the need for energy- and water-efficient processing strategies for materials utilization. This requires considering materials requirements in a broader context.
Consistent with recent progress in developing materials solutions for energy needs, a number of MR directions have embraced the challenge of materials availability and supply chain considerations. In this paradigm, it is not enough to simply discover a new higher-performing material, but rather one includes as a materials design/selection criteria the availability of sufficient quantities of a given material
___________________
49 The conversion is needed not only on a global scale but also for space travel; see C. Dunnill, “Method of Making Oxygen from Water in Zero Gravity Raises Hope for Long-Distance Space Travel,” The Conversation, July 10, http://theconversation.com/method-of-making-oxygen-fromwater-in-zero-gravity-raises-hope-for-long-distance-space-travel-99554.
50 J. Cooper, M. Noon, C. Jones, E. Kahn, and P. Arbuckle, 2013, Big data in life cycle assessment, Journal of Industrial Ecology 17(6):796-799.
via a reliable supply chain as an important element of desired functionality. The recent focus on rare-earth materials as “critical materials” with limited domestic supply is an exemplar of this challenge. Mitigation strategies include not only more efficient use of rare earths in such systems but also the search for higher-performing non-rare-earth alternatives and a focus on materials recycle/recovery after utilization (see Box 3.2). Similarly, research on advanced fuel cells should continue to emphasize seeking nonplatinum catalysts to reduce cost and improve sustainability.
Materials life cycle considerations are a broader example of the recent focus on materials recycling and reuse. To enhance sustainability, a number of manufacturing processes are exploring materials recovery and recycling as a source term for needed materials input. This focus impacts manufacturing methods due both to varied forms of materials input as well as an explicit focus on materials recovery after intended use. Thus, the efficient and effective use of a material during its full system life cycle, rather than achieving a singular functional response, becomes the overall objective. Done well, this engineering approach dramatically enhances both systems performance and full life cycle costs.
Last, a central element of sustainability strategies is the recognition of the intimate coupling of energy and water utilization. From an MR perspective, this has dual implications. A focus on energy-water sustainability emphasizes efficient materials processing and manufacturing strategies that are not energy or water intensive, with recent advances in AM being a prime example. Further materials discoveries in advanced catalysts and separation science enhance the efficient use of energy and clean water, thereby advancing energy and water sustainability.
The emergence of sustainability as a key theme in MR has both necessitated a broader focus on integrated materials solutions and enhanced the utility of these advances. By including sustainability as well as reliability as “design parameters” in seeking advanced materials functionality, materials discoveries are more readily incorporated in systems solutions. This focus on sustainability accelerates the adoption of these breakthroughs, further emphasizing the impact of materials discovery for societal needs.
3.9 MATERIALS TO MOVE, STORE, PUMP, AND MANAGE HEAT
Heat management has become one of the most crucial aspects of many technologies, ranging from batteries to hypersonic aircraft. For example, continued downward scaling of integrated circuits is limited significantly by heat management from electron devices (see the discussion in Section 3.3 for further details). Heat management also limits the performance and reliability of high-frequency and
high-power amplifiers and switches.51 Furthermore, greater than 90 percent of all energy used by humanity as electrical power in heating and cooling applications or for transportation originates from thermal processes. Consequently, even small efficiency improvements in our ability to control and convert thermal energy has a significant impact on the world’s energy equation. Last, as the world population increases in warmer regions of the planet with concomitant increases in wealth, there reasonably will be an increased demand for climate control. This must occur with sustainable cooling technologies that have minimal impact on climate change.
Because small efficiency increases can have a significant impact on energy use in high-demand devices and applications, increased effort in developing materials that can store, convert, pump, and otherwise manage energy in its thermal form is required. Such materials could be developed to a sophistication that rivals electronic and structural materials. The potential for significant technological impact from fundamental studies of thermal processes is assessed in Shi et al. (2015).52
In general, heat is the energy stored in the form of random thermal fluctuations of particles, typically atoms and electrons. First-order phase transitions and their accompanying enthalpy changes likely are the most widely used form of heat storage and conversion, the most ubiquitous example being the three-centuries-old and still widely used steam power systems that enabled the Industrial Revolution.
3.9.1 Thermal Energy Storage
Solar-thermal electricity generation presently is in large-scale use.53 The technology today is more costly than photovoltaic electricity without storage, but it has one major advantage in that the heat from the sun can be stored during daytime hours, then used during high-electric-demand evening hours. The energy storage in solar-thermal today uses molten salts. The thermal efficiency of electricity production improves as operating temperatures increase. Improvements in efficiency thus require the development of more stable and corrosion-resistant materials. Research into new phase-change materials with larger changes in heat of melting54 would also have significant impact as well as raising the efficiency of the conversion.
___________________
51 S.V. Garimella, A.S. Fleischer, J.Y. Murthy, A. Keshavarzi, R. Prasher, C. Patel, S.H. Bhavnani, et al., 2008, Thermal challenges in next-generation electronic systems, IEEE Transactions on Components and Packaging Technologies 31(4):801-815.
52 L. Shi, C. Dames, J.R. Lukes, P. Reddy, J. Duda, D.G. Cahill, J. Lee, et al., 2015, Evaluating broader impacts of nanoscale thermal transport research, Nanoscale and Microscale Thermophysical Engineering 19(2):127-165.
53 See, for example, NRG Energy and BrightSource Energy, “Ivanpah Solar Power Facility,” http://www.ivanpahsolar.com/, accessed June 6, 2018.
54 I. Gur, K. Sawyer, and R. Prasher, 2012, Searching for a better thermal battery, Science 335:1454.
3.9.2 Solid-State Thermal Energy Conversion
Thermoelectrics and thermophotovoltaic effects are heat conversion engines with no moving parts; therefore, in principle, they do not wear, require no maintenance, and promise a near-infinite lifetime. They can be used as heat pumps for refrigeration and cooling or as electricity generators. Thermoelectric cooling has a very high specific power and uses no GHGs, and thermoelectric power generation has been studied for waste heat recovery. Both technologies work over very large temperature ranges because they do not rely on phase changes. The past decades have seen the efficiency of thermoelectric materials double (measured by the figure of merit, ZT). It is unlikely that further reducing the lattice thermal conductivity, the main contribution to the doubling of ZT achieved in the past 20 years,55 will result in significant future progress because the lattice thermal conductivity of modern thermoelectric materials is close to the amorphous limit. Totally new approaches are needed. Note that the best thermoelectric used in cooling applications belongs to the same class of materials as the best topological insulators.56 Promising approaches involve solids with energy dispersion relations that depart significantly from those of conventional bands, such as correlated electron systems, systems with topological or chiral features, or spin-based thermoelectric effects.57
Magnetocaloric (adiabatic demagnetization) effects rely on the heat that accompanies the ferromagnetic/paramagnetic phase change. Electrocaloric effects rely on the heat accompanying the ferroelectric-paraelectric phase change, but these are much smaller than magnetocaloric effects and may not be as promising. Both latter technologies rely on phase changes, thus operating only at a fixed temperature (unlike thermoelectric conversion), and also require heat switches (see later). Promising materials should be developed that have particularly large enthalpies of magnetization of polarization, but also have minimal hysteresis, which leads to cycle losses.
3.9.3 Active Thermal Devices, Rectifiers, and Switches
All the above technologies stand to gain from the discovery and development of new materials in which heat conduction is nonlinear (i.e., the heat flux is not proportional to the temperature difference) or is controlled externally. There is a
___________________
55 K. Biswas, J. He, I.D. Blum, C.-I. Wu, T.P. Hogan, D.N. Seidman, V.P. Dravid, and M.G. Kanatzidis, 2012, High-performance bulk thermoelectrics with all-scale hierarchical architectures, Nature 489(7416):414.
56 J.P. Heremans, R.J. Cava, and N. Samarth, 2017, Tetradymites as thermoelectrics and topological insulators, Nature Reviews Materials 2:17049.
57 K. Vandaele, S.J. Watzman, B. Flebus, A. Prakash, Y. Zheng, S.R. Boona, and J.P. Heremans, 2017, Thermal spin transport and energy conversion, Materials Today Physics 1:39-49.
technological need for efficient thermal rectifiers, amplifiers, and switches. Just like modern electronics is based on nonlinear electrical circuit elements like diodes and transistors, such active thermal devices would be particularly relevant if implemented in solid-state form.
The technological impact of active nonlinear thermal materials would reveal itself in applications in which one needs to keep materials at a constant temperature, such as batteries, or where one has to keep things cool intermittently, such as spot-cooling on an integrated circuit. In another example, solid-state thermal switches could eliminate the moving parts in magnetocaloric and electrocaloric cycles. The most intriguing possible application for active thermal circuits would be in increasing the thermodynamic efficiency of heat engines operating under time-dependent loads. Indeed, by combining a pair of heat switches with a pair of heat reservoirs (thermal energy storage devices), one can increase the temperature difference across which a heat engine operates (see Figure 3.5), but only if that heat engine operates with a cyclical heat source (e.g., the sun in a solar-thermal system).58 This in turn increases the Carnot thermal efficiency of such an engine.
A number of ideas for materials that could act as thermal switches or rectifiers are reviewed in the recent literature.59 One direction for the research in active thermal materials will involve connecting thermal properties to external forces, such as strain, electric, or magnetic field. Another direction is the exploration of phase transitions, driven either by temperature itself or by the same external forces as above. New ideas are constantly being developed, including the use of topologically protected surface states.60
3.9.4 Thermal Barrier Coatings
Advances in coating technologies have also engendered significant advances in and increased the reliability and use of multilayered wear, thermal, and environmental protection systems. Layered material systems are replacing advanced monolithic materials in a growing number of applications where the unique properties and functionality of each layer provides dramatically increased performance and life. Thermal barrier coatings for metallic Ni-base superalloys and environmental barrier coatings for CMC are two examples of layered material systems that have been developed for extreme environments, consisting of high stresses and oxidizing and corrosive gases at temperatures on the order of 1500°C. These chemically,
___________________
58 G. Wehmeyer, T. Yabuki, C. Monachon, J. Wu, and C. Dames, 2017, Thermal diodes, regulators, and switches: Physical mechanisms and potential applications, Applied Physics Reviews 4:041304.
59 Ibid.
60 T.M. McCormick, S.J. Watzman, J.P. Heremans, and N. Trivedi, 2017, “Fermi Arc Mediated Entropy Transport in Topological Semimetals,” arXiv:1703.04606.
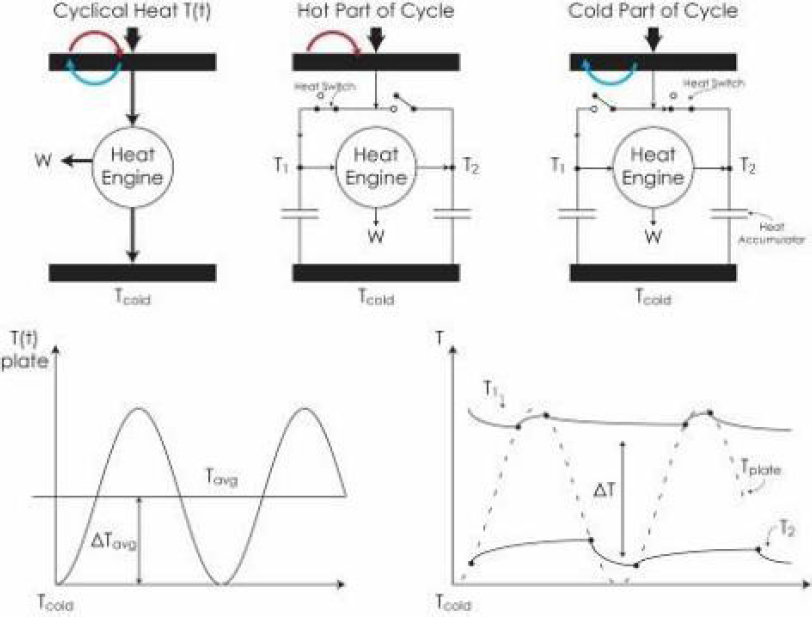
mechanically, and physically diverse layers interact and evolve throughout the lifetime of the coating, and important phenomena affecting performance and durability occur in each layer and particularly at the interfaces between disparate materials. The successful implementation of strain tolerance and low-conductivity coatings have dramatically increased coating lifetimes. As is the case for most materials designed specifically for thermal application, materials capable of operating at higher temperature and better able to withstand wear and corrosion would significantly extend the range of applications in which they can be deployed.
3.10 FINDINGS AND RECOMMENDATIONS
Key Finding: The Materials Genome Initiative, and the earlier Integrated Computational Materials Engineering approach, recognized the potential of integrating and coordinating computational methods, informatics, materials characterization, and synthesis and processing methods to accelerate the discovery and deployment of designer materials in products. The translation of these methodologies to specific industries has resulted in numerous successful applications that have reduced the development time with corresponding cost savings.
Key Recommendation: The U.S. government, with the National Science Foundation, Department of Defense, and Department of Energy coordinating, should support the quest to develop new computational and advanced dataanalytic methods, invent new experimental tools to probe the properties of materials, and design novel synthesis and processing methods. The effort should be accelerated from today’s levels through judicious agency investments and continue over the next decade in order to sustain U.S. competitiveness.
Key Finding: Research into metals, alloys, and ceramics continues to provide fundamental understanding of atomic-scale processes that govern synthesis-microstructure-property relations in many classes of materials. Armed with this understanding and state-of-the-art synthesis, characterization, and computational tools, scientists can realize novel alloys and micro/nanostructures with extraordinary properties. Traditional areas of materials research can have surprising new developments, for example, in multicomponent, high-entropy alloys and inorganic glasses.
Key Recommendation: Federal funding agencies (National Science Foundation, Department of Energy, Department of Defense) should maintain robust programs to support, and in some cases expand, fundamental research in longestablished areas such as metals, alloys, and ceramics.
Key Finding: Quantum materials science and engineering, which can include superconductors, semiconductors, magnets, and two-dimensional and topological materials, represents a vibrant area of fundamental research. New understanding and advances in materials science hold the promise of enabling transformational future applications, in computing, data storage, communications, sensing, and other emerging areas of technology. This includes new computing directions outside Moore’s law, such as quantum computing and
neuromorphic computing, critical for low-energy alternatives to traditional processors. Two of the National Science Foundation’s “10 Big Ideas”61 specifically identify support of quantum materials (see “The Quantum Leap: Leading the Next Quantum Revolution” and “Mid-scale Research Infrastructure”).
Key Recommendation: Significant investments by, and partnerships among, the National Science Foundation, Department of Energy (DOE), National Institute of Standards and Technology, Department of Defense (DoD), and Intelligence Advanced Research Projects Activity will accelerate progress in quantum materials science and engineering, so crucial to our future economy and homeland security. U.S. agencies with a stake in advanced computing, under the possible leadership of DOE’s Office of Science and National Nuclear Security Administration laboratories and DoD research laboratories (Army Research Laboratory, Naval Research Laboratory, and Air Force Research Laboratory), should undertake to support new initiatives to study the basic materials science of new computing paradigms during the next decade. To remain internationally competitive, the U.S. materials research community must continue to grow and expand in these areas.
Key Finding: Materials science and technology has an enormous impact on the quality and sustainability of Earth’s environment across the entire spectrum of materials types. This is another important opportunity for university, national laboratory, and industry cooperation.
Key Recommendation: Research in numerous directions that improve sustainable manufacturing of materials, including choices of feedstocks, energy efficiency, recyclability, and more, is urgently needed. Creative approaches for funding materials research toward sustainability goals should be developed by the National Science Foundation, the Department of Energy, and other agencies.
Finding: Sustainability and environmental impact have special import in the domain of polymer materials research owing to the dual factors of the massive accumulation of discarded polymeric materials in the environment and the unique challenges to polymer recyclability.
Recommendation: The massive accumulation of discarded polymeric materials points to several needed actions, namely, stimulating and executing
___________________
61 National Science Foundation, “NSF’s 10 Big Ideas,” https://www.nsf.gov/news/special_reports/big_ideas/, accessed September 22, 2018.
further research on environmental degradability of polymers, better methods of separating incompatible polymers out of waste streams, research toward recycling without separation, and fundamental research in green chemistry possibilities within polymer research.
Finding: Over the past decade, it has become increasingly clear that the modern materials enterprise, from mining of raw materials to processing to disposal, often affects the environment and ecosystems and consumes considerable energy.
Recommendation: All government agencies funding materials research should explore ways of including sustainability, reliability, and life cycle analysis in appropriate grants dealing with new materials, particularly those that are close to industrial usage. The Environmental Protection Agency and other agencies able to influence sustainability should immediately begin to support fundamental research, from the molecular to the systems level, at a rate that can advance materials sustainability within the next decade.
Finding: The real and potential importance of composite and hybrid materials is enormous, encompassing a diversity spanning organo-metal hydride perovskites for photovoltaics, to polymers reinforced with micro- or nanoscale inorganic particles to yield unique properties, to natural biohybrid materials with steep spatial gradients in their properties. The important zone in composite or hybrid materials is very frequently at the interfaces between materials types.
Recommendation: The National Science Foundation and Department of Energy, as well as other agencies with a stake in the composite and hybrid materials field, should support modalities such as biennial workshops and also centers (akin to Fraunhofer Institutes) to foster fundamental research on composite and hybrid materials across all relevant disciplinary boundaries. Both efforts should, at the latest, start by 2022 and be supported for a minimum of a decade.
Finding: The fields exemplified by metamaterials for photonics, phononics, and plasmonics have impressive growth potential, and could have significant impact on the next generation of tailored functional materials having properties beyond what is found in nature. New opportunities exist in the creation of multimaterial, multiscale, and multifunctional structures.
Recommendation: To advance the fields represented by metamaterials for photonics, phononics, and plasmonics, new computational techniques, as
well as a focus on addressing manufacturing challenges are needed. This need for new computational techniques and addressing manufacturing challenges is especially true when related to the quality and control of additively manufactured materials, and the development of new technologies such as threedimensional nanofabrication.
Finding: The benefits of using shape and geometry to define material response have been clearly identified for a variety of metamaterials and their attendant properties, but the design, fabrication, and use of metamaterials is still in its infancy, while contributions are seen in many fields.
Recommendation: The National Science Foundation and Department of Energy should create ecosystems that support interdisciplinary efforts to merge topology optimization and other computerbased design tools with costeffective manufacturing processes that will advance the production of multifunctional metamaterials with requisite internal shapes and architectures.
Finding: Advances in polymer science across the board, from polymer physics to new, applied, functional, organic materials, are often driven by powerful polymer synthesis capabilities.
Recommendation: Examples of directions in polymer materials synthesis that should be pursued vigorously by the community include precision synthesis (synthetic methods aimed at biological levels of control of macromolecular structure); selfassembly and noncovalent synthesis (including advancing our understanding of hydrophobic, electrostatic, hydrogenbonding, π-π and cationπ electronic interactions); and dynamic covalent bonding and selfhealing. This recommendation is aimed first at the polymer synthesis research community to continue to generate new ideas along these lines, but then action is needed at the funding agencies that support polymer synthesis research.
Finding: Soft matter deals with a wide variety of materials, including polymers, colloids, foams, emulsions, liquid crystals, and even the flowing mixture of dirt and water that is a mudslide. It has provided liquid crystal displays, microfluidics, and insights to such fundamental concepts as topological defects and maximum packing of odd-shaped objects. It is poised to provide smart materials that change shape in response to environmental cues and parts for soft robots. It is leading research into self-assembly and the exciting new field of active matter, and its impact on related fields like biomaterials and bio-inspired
materials will continue to grow. It is crucial for soft matter to be a part of the nation’s research portfolio.
Recommendation: Given the diversity of directions in soft matter research, federal agencies that fund materials research, particularly the National Science Foundation and Department of Energy, should keep abreast of advances in soft matter through focused workshops and provide support for research on subjects such as selfassembly and active matter that show great promise.
Finding: The crossroads of materials research for use in biological environments (biomaterials) and the derivation of insight into new materials science through the understanding of biology (bio-inspired materials) is very rich and continues to generate new materials science.
Recommendation: Agencies such as the National Institutes of Health, National Science Foundation, Department of Energy, and Department of Defense should work together to provide interagency support for research in biomaterials and bioinspired materials because of the fundamental interdisciplinarity of such research.
Finding: Extreme operating conditions (ultrahigh temperatures, pressures, strain rates, high-dose irradiation, or corrosive environments) pose challenges in materials discovery, synthesis, processing, and verification. Recent advances in computational modeling and innovative extreme testing methods are providing new insight into material structure and behavior, such as those for nonoxide ceramic systems that melt at temperatures near 4000°C.
Recommendation: To enhance materials understanding under extreme conditions, federal agencies (National Science Foundation, Department of Energy, National Aeronautics and Space Administration, Department of Defense) should work in concert to provide support for computational modeling and for the development and operation of shared experimental facilities for extreme conditions.
Finding: Heat management is an integral part of the design of many systems and for batteries, electronic circuits, engines, turbine blades, and materials for extreme environments. Heat management, storage, conversion to electricity, and active control of heat flow promise improvements in energy efficiency and size and cost reductions in a wide variety of technologies. Materials are needed that enable these technologies.
Recommendation: Fundamental research on the thermal properties of materials should be integrated into the material design process, and not be limited to a mere material selection process. In many cases, research on the thermal properties of a material should be considered on an equal footing with that on the electronic or mechanical properties. Predictive tools should be developed for thermal conductivity, heat capacity, and other thermal properties in the design of new materials. Research on new approaches beyond the classical band structure and crystal lattice properties should be encouraged. Steps should be taken by the National Science Foundation and Department of Energy, possibly in cooperation with other government agencies such as the National Institute of Standards and Technology and the Department of Defense, to encourage research proposals related to solidstate devices for thermal energy conversion, rectification, and switching.
Finding: Metals and semiconductors have long been among the most important of drivers of the U.S. economy, providing everything from skyscrapers to computer chips. Yet they continue to provide interesting and important opportunities for further development such as the possibility of bespoke materials through high-entropy alloys or atomic-scale precision in the manufacture of semiconductor materials.
Recommendation: Government funding agencies should pay careful attention to exciting advances and opportunities in fields such as metals and semiconductors without neglecting these and other more established but nonetheless important projects in traditional fields where incremental advances can still have a big impact.
Finding: The physical limits of scaling, or miniaturization, of silicon-based technology in computer chips are expected to be reached in the next decade. Given the multiyear timeline for bringing new materials for computing to manufacture, materials research to support new computing methodologies is essential.
Recommendation: The Department of Energy, Department of Defense, National Science Foundation, National Institute of Standards and Technology, National Institutes of Health, and other agencies with a stake in advanced computing should, for the next decade, place a high priority on materials research that advances new modalities of information technology.
Finding: Increasingly, biomaterials designed to regenerate tissues require functional properties other than mechanical, such as molecularly based bioactivity
for signal transduction or safe and rapid biodegradation, that are challenging fundamental research capacity. These define a broad new direction in materials research that will create the knowledge necessary to bring synthetic materials, and devices made from them, into more intimate functional contact with human physiology, both internally, for medical purposes, and externally, for a wide range of potentially wearable devices.
Recommendation: All agencies, particularly the National Institutes of Health, which has a very specific interest in biomaterials, should participate in supporting fundamental science of these materials at a level relevant for their mission during the next decade. The Department of Defense should expand its research funding in this area as part of its interest in humanmachine interaction and warfighter performance augmentation.
Finding: The proliferation of waste products that pollute the environment and threaten Earth’s ecosystems is an international problem that demands international cooperation.
Recommendation: All government agencies, particularly those funding materials research that includes life cycle studies, should seek to establish communication links among similar agencies in other countries by 2020 to bring the best of world research to bear on the problem of the proliferation of waste products that pollute the environment and threaten Earth’s ecosystems.