5
Topology Optimization of Soft Materials and Deformable Structures
Soft and compliant structures could unlock new applications in medicine and bioengineering, wearable structures and devices, and human-machine interfaces. While topology optimization for rigid materials and structures is quite mature, there is a lack of tools and methods for topology optimization of soft or compliant structures, as well as of structures that undergo large deformations as part of their intended function. The workshop’s third session focused on gaps and opportunities in this space. Speakers were asked to address the fundamental challenges for topology optimization of soft structures, how these challenges might be overcome, and the applications and domains that may offer transformational new opportunities.
Frank Zok, University of California, Santa Barbara, introduced the speakers: Brett Compton, University of Tennessee, Knoxville; Mark R. O’Masta, HRL Laboratories; and Xiaoyu (Rayne) Zheng, University of California, Los Angeles. Following the presentations, Ned Thomas, Rice University, moderated a brief Q&A.
MATERIAL EXTRUSION ADDITIVE MANUFACTURING OF MULTI-MATERIAL HYBRID STRUCTURES
Brett Compton, University of Tennessee, Knoxville
Compton discussed challenges and advantages of material extrusion additive manufacturing (MEAM), stressed the importance of understanding materials science relationships, and described MEAM’s effects on material properties.
MEAM Challenges and Advantages
Topology optimizations for MEAM face two challenges: (1) current manufacturing constraints and (2) a lack of understanding of the precise material- and process-specific outcomes. Despite these challenges, the flexibility MEAM offers holds great promise. MEAM enables the fabrication of integrated designs of material architecture and component geometry, at the micro-, meso-, and macroscale, using new materials and with new architectures, such as lattices and nacre-like architectures.
MEAM encompasses a wide variety of methods, including commercial fused filament fabrication and fused deposition modeling for thermoplastic materials, as well as direct ink writing. Compton said that the advantages of MEAM are that it can place materials precisely, incorporate multiple print heads for multiple materials, and align fibers as desired, enabling control of fillers to create composite materials.1 It is also amenable to a wide range of feedstocks, from epoxy resins to thermoplastic polymers to metals. Finally, it offers the critical ability to choose the print path, making it possible to manipulate fiber directionality for reinforced systems.
Understanding Materials Science Relationships
Compton stressed that MEAM methods require an understanding of the classic materials science relationships between structure, process, and property. His work has focused on deepening the understanding of how certain materials and composites react to the extrusion-based printing process, for example, how composition and print parameter choices affect strength, stiffness, and functional properties in printed materials.2 In addition to the materials themselves, the extrusion hardware design affects material properties. For example, nozzles can be designed to control fiber orientation, from straight to transverse; to create graded lattice structures, enabling a wide variety of mechanical properties from one feedstock; and to hold multiple materials.
MEAM’s Effects on Material Properties
MEAM creates products by printing layers within a shape-defining perimeter. Controlling material properties is very important, and the choices MEAM offers for density, infill direction and shape, nozzle size, deposition rate, and layer height
___________________
1 B.G. Compton and J.A. Lewis, 2014, 3D-printing of lightweight cellular composites, Advanced Materials 26(34): 5930-5935, https://doi.org/10.1002/adma.201401804.
2 B.G. Compton, N.S. Hmeidat, R.C. Pack, M.F. Heres, and J.R. Sangoro, 2018, Electrical and mechanical properties of 3D-printed graphene-reinforced epoxy, Journal of The Minerals, Metals & Materials Society 70: 292-297, https://doi.org/10.1007/s11837-017-2707-x.
are critical to printing quality composite materials, Compton said. However, he noted, these choices are not currently incorporated into design tools.
Because all of these variations strongly influence the finished product, Compton said it is essential that they be incorporated into designs. For example, rotating the nozzle a certain way controls the fiber arrangement, which in turn affects stiffness, strength, and, significantly, transport properties.3 In experiments with multiple materials using classic SIMP-based topology optimizations, Compton has learned that adjusting these variables affects several properties of the printed product, such as toughness, damage tolerance, and load-deflection behavior. Composite feedstocks show even greater complexities, and there are also significant differences when changing nozzle size or flow rate.
Compton has also experimented with multi-material architectures, specifically a low-density, compliant core material encased in a stiffer shell material, similar to a porcupine quill or sunflower stalk; and with graded and multi-material honeycombs, to which MEAM is especially well suited. For honeycombs, changing the gradient scheme, wall length, orientation, and other architectural features changes stiffness and collapse loads, making it possible to tailor stiffness and damping behavior for specific structural needs. With these new architectures more readily accessible with additive manufacturing, it becomes more important to have tools and approaches to quantify heterogeneous deformation in such structures, Compton noted.
Q&A
In response to a question about out-of-plane buckling of elastomeric honeycombs, Compton noted that it was important to find an aspect ratio where the honeycombs would not buckle during compression testing. In general, he said, these materials are very compliant with low in-plane stiffness, and out-of-plane buckling does not present a major issue. Hardik Kabaria, Carbon, Inc., suggested changing the struts’ cross-sections to control buckling, and Compton agreed that was a possibility.
William Benard, Army Research Laboratory, asked about Compton’s data needs. Acknowledging that his work is far from creating production parts, Compton said his focus is on improving understanding between process and property. To that end, he sees a need for new x-ray tools to enable better data collection and characterization.
Jonathan Berger, Nama Development LLC, asked about limitations to fiber orientation. Compton replied that fiber orientation is related to both the nozzle
___________________
3 J.R. Raney, B.G. Compton, J. Mueller, T.J. Ober, K. Shea, and J.A. Lewis, 2018, Rotational 3D printing of damage-tolerant composites with programmable mechanics, Proceedings of the National Academy of Sciences U.S.A. 115(6): 1198-1203, https://doi.org/10.1073/pnas.1715157115.
rotation rate and the diameter of the nozzle, which presents challenges at the small scale but may be feasible at the large scale.
STRUCTURE AND MATERIAL OPTIMIZATION FOR ADDITIVE MANUFACTURING
Mark R. O’Masta, HRL Laboratories
O’Masta discussed structural material optimization for additive manufacturing as a way to translate innovative technology developed in academia into usable industry parts, particularly for automotive and aerospace applications, which rely on traditional manufacturing streams. O’Masta also detailed processes for optimizing structures with soft materials and new metals materials his team has developed for optimizing parts.
Making Optimizations Useful
Optimizations will only be useful to industry if they account for design constraints, including secondary properties such as weight and loading conditions; incorporate environmental stress; offer strong cost benefits; and enable quality control, O’Masta said. In addition, these components have a wide range of requirements, from how a product will be manufactured, to individual product features, to coexisting with surrounding components and processes, to the possibility of repair or recycling.
O’Masta showed several examples of deforming materials for impact resistance, at both high and low velocities. For example, a better-designed helmet could reduce head trauma. Optimizing existing helmet topologies, however, is difficult because such nonlinear problems are very computationally intensive. Instead, O’Masta’s team added a layer of architected porous material to the helmet to spread the impact energy over a larger area, lowering the peak force of an object against a human skull. By constraining the porous material to comprise a repeating unit cell described by only a handful of parameters, O’Masta’s team considerably reduced the design space, making the architecture optimization process computationally feasible. The team discovered that using self-propagating waveguides to create 3D lattice structures was quick and economical and—over the course of several simulations, experiments, and iterations—they created a helmet that outperforms even state-of-the-art foam padding.4
___________________
4 E.C. Clough, T.A. Plaisted, Z.C. Eckel, K. Cante, J.M. Hundley, and T.A. Schaedler, 2019, Elastomeric microlattice impact attenuators, Matter 1(6): 1519-1531, https://doi.org/10.1016/j.matt.2019.10.004.
Developing New Metals Materials
Without access to the right materials, optimized designs will underperform. Therefore, O’Masta’s team also develops new metal alloys to enable additive manufacturing of optimized parts. Existing high-strength alloys crack or tear during the 3D-printing process; to make those alloys printable, the team has added inoculants that create a very fine equiaxed microstructure that is resistant to cracking.5 Their inoculant-added 7000 series aluminum alloy has strong commercial potential, and it recently was granted the material designation 7A77, a first by the Aluminum Association for a 3D-printable material.6 The inoculant process is alloy agnostic, so theoretically similar performance improvements will be achieved with other alloys systems, O’Masta said.
HRL Laboratories is also seeking to create metals with properties and microstructures that can be tailored to specifications. In addition, O’Masta’s team is creating a feature within the optimization itself to identify the most promising materials. It may also be possible to use heterogeneous nucleation sites for dispersoid strengthening to increase yield strength and reduce wear, he said.
Looking ahead, O’Masta expressed his hope that that the future of design will bring opportunities to optimize structure and materials for manufacturability at both scale and cost. Once that has been achieved, the next challenge will be to model and optimize the entire process.
Q&A
Katherine T. Faber, California Institute of Technology, asked for details on dispersoid dimensions. O’Masta replied that there are restrictions for print layer thickness, which varies depending on the process being used, and he added that for polymeric printing a dual cure approach was promising.
Zok asked if self-propagating waveguides could be used with polymers. While acknowledging that this is not his area of expertise, O’Masta noted that his team has had success with the urethane chemistries in helmets. Thomas noted that helmets are a very small design space, and O’Masta agreed but also noted that while that may create limitations, it does not preclude structural optimizations to create improvements.
Manoj Kolel-Veetil, Naval Research Laboratory, suggested that helmets might need different structures for different areas—trusses on the side and lattices at the
___________________
5 J.H. Martin, B.D. Yahata, J.M. Hundley, J.A. Mayer, T.A. Schaedler, and T.M. Pollock, 2017, 3D printing of high-strength aluminium alloys, Nature 549: 365-369, https://doi.org/10.1038/nature23894.
6 Aluminum Association, 2019, “Aluminum Association Introduces First-Ever Material Designation System for 3D Printing,” press release, April 15, https://www.aluminum.org/news/aluminumassociation-introduces-first-ever-material-designation-system-3d-printing.
front, for example—to support the brain during translational and rotational loads. O’Masta agreed, suggesting that this should be incorporated in design optimization to protect the head from all angles.
ACHIEVING MORE WITH LESS: ARCHITECTING METAMATERIALS WITH ADDITIVE MANUFACTURING
Xiaoyu (Rayne) Zayne, University of California, Los Angeles
Zheng described present and future materials manufacturing processes, stressing that additive manufacturing must be tailored to a material’s topology, constituents, and feature sizes in order to achieve the ultimate goal: utilization of architected metamaterials for multifunctional devices.
Materials, Present and Future
Design and manufacturing of materials today is an additive, iterative process in which a raw material is continually tested, measured, and adjusted for certain properties. The starting material is often a limiting factor that must be designed around, depending on the desired component properties.
The future of materials design and manufacturing, as Zheng sees it, is to start with targeting properties and behaviors, such as dielectric permittivity, conductivity, stress/strain curves, or spatial and time resolved behavior. Architecting metamaterials, he said, can enable the creation of materials with those targeted properties and behaviors to be replicated by additive manufacturing.
Architected Metamaterials and Their Structural Responses
Three factors are crucial to the structural responses of architected metamaterials: (1) architecture; (2) size effect, which includes topology, unit cell size, and size scale; and (3) material cues (i.e., all of the disparate properties inside a material). These three factors must be balanced when creating metamaterials for additive manufacturing, Zheng said.
Architecture can be tailored several different ways. For example, microstereolithography can be used to adjust topology, direction, and size, creating ultralight, ultrastiff metamaterials.7 Zheng’s team has also achieved super elasticity in multiscale metallic metamaterials, even brittle ones, creating the ability to selectively
___________________
7 X. Zheng, H. Lee, T.H. Weisgraber, M. Shusteff, J. DeOtte, E.B. Duoss, J.D. Kuntz, M.M. Biener, Q. Ge, J.A. Jackson, S.O. Kucheyev, N.X. Fang, and C.M. Spadaccini, 2014, Ultralight, ultrastiff mechanical metamaterials, Science 344(6190): 1373-1377, https://doi.org/10.1126/science.1252291.
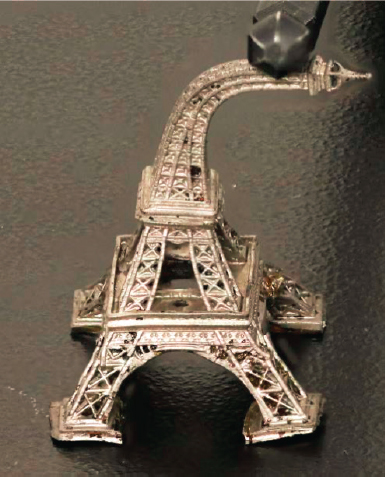
activate different buckling modes (see Figure 5.1).8 These materials can be bent or twisted to harness the power of multiscale 3D architectures and size effects, including stress/strain curves, expanding what is possible for additive manufacturing.9
The length-scales of unit cells with respect to the overall dimension of metamaterials are also important to create successfully architected metamaterials. For example, fracture toughness is essential for effective material design and utilization. While defining fracture toughness is challenging, Zheng’s team has learned that the number of unit cells that span a crack and the strut geometries are critical.10 Designed embedded cracks also affect failure modes, another size effect, but it is not yet known if those can be architected, Zheng noted.
___________________
8 X. Zheng, W. Smith, J. Jackson, B. Moran, H. Cui, D. Chen, J. Ye, N. Fang, N. Rodriguez, T. Weisgraber, and C.M. Spadaccini, 2016, Multiscale metallic metamaterials, Nature Materials 15: 1100-1106, https://doi.org/10.1038/nmat4694.
9 C.S. Ha, R.S. Lakes, and M.E. Plesha, 2019, Cubic negative stiffness lattice structure for energy absorption: Numerical and experimental studies, International Journal of Solids and Structures 178-179: 127-135, https://doi.org/10.1016/j.ijsolstr.2019.06.024.
10 H. Cui, M. O’Masta, V. Deshpande, and X. Zheng, 2018, “Fracture Toughness of Hierarchical, Low Density Architected Metamaterials,” in Proceedings of the IUTAM Symposium Architectured Materials Mechanics (T. Siegmund and F. Barthelat, eds.), Purdue University Libraries Scholarly Publishing Services, https://docs.lib.purdue.edu/iutam/presentations/abstracts/18.
Patterning material cues inside of architectures is also essential. With multimaterial stereolithography, it is possible to combine monomers with different stiffnesses. Synthesizing different monomers that carry distinct electrostatic charge polarities can be exploited to selectively deposit a variety of materials, such as metals, dielectrics, metal oxides, carbon nanotubes, and even mixtures, inside complex 3D architectures.11 This research, in collaboration with the Air Force Research Laboratory, led to the creation of waveguide and antenna arrays.
Utilizing Architected Metamaterials for Multifunctional Devices
Metamaterials can be created for many different behaviors and properties. The next challenge, Zheng said, is to utilize these features to design multifunctional devices. Zheng’s team and the Office of Naval Research are collaborating on a method to design arbitrary target stress/strain curves for properties such as maximum strength, stiffness, and density, and they are using artificial intelligence, machine learning, and additive manufacturing to achieve a material that replicates a designed mechanical behavior.
Zheng created a schematic for a machine learning–based design methodology (including feature scaling, behavior mapping, and topology via classification and regression) that can replicate the entire structured behavior of a metamaterial. This methodology was used to test multiple stress/strain curves for shoe insole designs. This inverse design of mechanical behaviors required a combination of simulation and experimentation in order to create training data that machine learning algorithms could use to capture the complete material structure behavior, including manufacturing defects, for all classification types.
These lattice materials must be multifunctional, meaning that they can be used as a device for energy transduction and communications, and so Zheng is pursuing additive manufacturing of piezoelectrics as a means of combining electric and mechanical properties. Piezoelectrics are defined by their crystalline architecture. Zheng has developed a method to synthesize piezoelectric nanocomposite colloids that can be 3D printed with adjustable monomer stiffness to achieve different levels of flexibility, which influences impact resistance.12 It is also possible to design voltage-strain conversions via 3D piezoelectric micro-architectures. In addition, Zheng and his team have found that it is possible for piezoelectrics to
___________________
11 R. Hensleigh, H. Cui, Z. Xu, J. Massman, D. Yao, J. Berrigan, and X. Zheng, 2020, Charge-programmed three-dimensional printing for multi-material electronic devices, Nature Electronics 3: 216-224, https://doi.org/10.1038/s41928-020-0391-2.
12 H. Cui, R. Hensleigh, D. Yao, D. Maurya, P. Kumar, M.G. Kang, S. Priya, and X. Zheng, 2019, Three-dimensional printing of piezoelectric materials with designed anisotropy and directional response, Nature Materials 18(3): 234-241, https://doi.org/10.1038/s41563-018-0268-1.
have different actuation modes and voltages, with precise laser patterns, from a distance of 4 meters or even underwater, for sound wave detection.
Wrapping up his presentation, Zheng reiterated that architecting metamaterials for additive manufacturing will require a thorough understanding of their architecture, size effects, and material cues in order to predict their structural properties and create multifunctional devices. Understanding this relationship may also make it possible to reverse engineer metamaterials, based on the desired structural responses, in order to design good candidates for use in additive manufacturing.
Q&A
Referring to Zheng’s work as a breakthrough, Benard asked if Zheng is pursuing ways to code signals to achieve different effects from different devices. Zheng replied that they are, although he noted that a large number of unit cells are needed. To overcome this constraint, he suggested some elements could be preprinted.
Claus Pedersen, Dassault Systèmes Simulia Corp, asked how many simulations Zheng’s designs required. Zheng answered that it depends on the classification and the testing. Pedersen argued that topology optimization would work for that problem, and in fact it is already being practiced today. Zheng said that experimentally based data acquisition could take into account all possible failures and manufacturing defect, and machine learning could eliminate the needs for nonlinear, large-scale simulations, suggesting a possible collaboration to explore this further.
When asked about crack sensitivity, Zheng responded that four-unit cells are mostly the threshold for crack sensitivity. Regarding node structure, Zheng noted that their hierarchical analyses show that nodes become hollow interconnections that can rotate and release energy.
Graeme Milton, University of Utah, asked how stress/strain curves are accounted for. Zheng explained that all the problems they seek to solve are simplified and then tested to discern average behaviors, taking into account defects or loading variations. Zheng also agreed that instability was possible in stretch-dominated architecture.
Angus Kingon, Brown University, pointed out that starting with empirical data for stress/strain curves does not help illuminate the distance from the boundary limits. Zheng agreed, but added that the specific structural architecture is the key to understanding the range of stress/strain curves and one day reach the boundary.
PANEL DISCUSSION ON EMERGING MATERIALS AND EFFORTS
Thomas introduced the three speakers who had been invited to address emerging materials and efforts in this field: Kabaria, Carbon, Inc.; Christopher M. Spadaccini, Lawrence Livermore National Laboratory (LLNL); and Ole Sigmund,
DTU Technical University of Denmark. Following their remarks, Zok moderated an open discussion.
Kabaria noted that while materials and processes are important to additive manufacturing, design tools are critical to manufacturing actual working parts, accelerating product innovation, and making additive manufacturing more widespread. To improve their design tools, Carbon developed a design parameter optimization process that takes customer inputs—material properties, desired mechanical responses, and design constraints—and creates an additive manufacturing-ready design. They rely on a closed loop optimization, from searching the lattice library, to designing the mechanical response, to multiple simulations and redesigns. Kabaria noted that this process is semi-automated so that design engineers can use it without a full understanding of the intricacies of optimizations or the properties of lattice structures.
Carbon runs thousands of these lattice design parameter optimizations, with particular attention to performance characteristics including post buckling behavior. Typically, the design with simulated data determined to be the best is then printed, although Carbon also offers customers options with varying secondary requirements. This process, Kabaria noted, will eventually become fully automated.
Carbon also employs open-source tools, Amazon Web Services, a robust lattice library, and in-house customized tools to create marketable consumer products, such as shoe midsoles, customized football helmets, and bicycle seats. Kabaria also briefly touched on how surface lattices create smoother transitions than strut-based lattices, noting that Carbon is working on a surgical device that utilizes this design software.
Spadaccini described several new materials design possibilities, including origami/folding structures, which can change shape without affecting properties;13 hollow-tube, liquid-filled materials that can change properties but not shape;14 materials with dynamically tunable stiffness, whose properties, such as stiffness, depend on the presence of a magnetic field; printable ceramics extruded via direct ink writing; micro- and nanoparticle-based feedstocks; and ceramic-metal composites for increased hardness with ductile qualities.
Spadaccini also mentioned several research areas that he sees as critical to advancing this work. First, the post-processing, including sintering, is crucial to material performance. Second, it is critical to design feedstocks in tandem with their printing process. Modeling to understand and optimize the printing process is also important.
___________________
13 K. Bertoldi, M.C. Boyce, S. Deschanel, S.M. Prange, and T. Mullin, 2008, Mechanics of deformation-triggered pattern transformations and superelastic behavior in periodic elastomeric structures, Journal of the Mechanics and Physics of Solids 56(8): 2642-2668, https://doi.org/10.1016/j.jmps.2008.03.006.
14 J.A. Jackson, M.C. Messner, N.A. Dudukovic, W.L. Smith, L. Bekker, B. Moran, A.M. Golobic, A.J. Pascall, E.B. Duoss, K.J. Loh, and C.M. Spadaccini, 2018, Field responsive mechanical metamaterials, Science Advances 4(12), https://doi.org/10.1126/sciadv.aau6419.
In situ probes and models, for example, have helped capture the detailed physics of the fabrication process, which can be used to understand and mitigate pore formation and defects in laser powder bed metal additive manufacturing.15 Finally, new characterization and metrology tools are needed in order to capture characteristics of the actual geometry that are difficult to measure with existing techniques (e.g., internal cavities). Those measurements are extremely difficult and costly to make but could lead to the creation of beneficial design constraints that result in better optimization, higher performance components, and potentially manufacturing advantages, Spadaccini suggested.
Sigmund began by clarifying two points. First, he said, topology optimization for finite deformations is possible, although the modeling may be challenging due to instabilities in low-density regions. One solution is to remove low-density elements during topology optimization, reintroducing them later, but that only works up to a certain degree of deformation. Second, negative Poisson’s ratio materials can be designed to have a prescribed deformation behavior, resulting in features closer to the desired behavior.16 These structures can then be parameterized and optimized to ensure simple manufacturing.17
Next, Sigmund proposed several discussion points. First, he argued that topology optimization today is so easy that uneducated users may produce subpar results, yet these users do not have the mechanical background to fully understand why these results are subpar. Possibly incorporating artificial intelligence and definitely human quality control points and further education throughout the process may help to improve results, he suggested.
In addition, he suggested that real-time topology optimization capabilities should be expanded, as they enable users to quickly visualize results, adjust parameters, and develop a more intuitive understanding of the process. Also, creating new additive manufacturing design rules would standardize results and eliminate unnecessary experimentation. Finally, he said it may be possible to design for energy absorption, rethink current safety factors in light of new capabilities, and challenge designers to improve support structures.
Zok opened the general discussion by sharing several observations. First, he noted that the polymers community is ahead of the metals community in terms of
___________________
15 S.A. Khairallah, A.T. Anderson, A. Rubenchik, and W.E. King, 2016, Laser powder-bed fusion additive manufacturing: Physics of complex melt flow and formation mechanisms of pores, spatter, and denudation zones, Acta Materiala 108: 36-45, https://doi.org/10.1016/j.actamat.2016.02.014.
16 F. Wang, O. Sigmund, and J.S. Jensen, 2014, Design of materials with prescribed nonlinear properties, Journal of the Mechanics and Physics of Solids 69: 156-174, https://doi.org/10.1016/j.jmps.2014.05.003.
17 F. Wang, B.S. Lazarov, O. Sigmund, and J.S. Jensen, 2014, Interpolation scheme for fictitious domain techniques and topology optimization of finite strain elastic problems, Computer Methods in Applied Mechanics and Engineering 276: 453-472, https://doi.org/10.1016/j.cma.2014.03.021.
materials printability. They have more workable materials and are designing products with very few materials, thus improving recyclability, he noted. In the metals community, by contrast, there is some promise in using inoculants, but the focus is still on traditional materials. He suggested that alloys and other composite materials should be prioritized and applied to more complex 3D structures, such as those with discontinuous fibers. In addition, Zok said there is great promise in multi-materials printing, especially for devices and high-temperature materials such as ceramics and ceramic composites.
Participants discussed the possibilities of using ceramics and other composites; designing for qualities such as elasticity, fracture toughness, defect sensitivity, and sound dynamics; and overcoming modeling difficulties.
Using Ceramics and Other Composites
Responding to Zok’s observations, Spadaccini suggested that while ceramics at the nanoscale are below the defect threshold and have promising properties, it is an open question if that outcome scales up. At LLNL, researchers have successfully scaled up the 2-photon nanoscale printing process to create larger overall components but still with nanoscale features and may soon be able to create centimeter-sized pieces with nanoscale features.
Faber suggested that scientists develop polymers with a high ceramic yield to remove existing limitations on truss sizes. With better polymers, it should be possible to create larger, non-trussed structural materials, she said. Zok proposed that the small market for such materials discourages exploration; Jim Lancaster, National Academies, noted that while bicycle seats, helmets, dentures, and connectors are each multibillion-dollar markets, ceramics may not have a similarly favorable market size and cost-benefit ratio.
Faber acknowledged that niche markets—those interested in high-temperature and lightweight performance, like aerospace—are the more likely possibilities in this space. Ryan Watkins, NASA Jet Propulsion Laboratory (JPL), agreed that ceramics were promising for magnetometers, but noted that his team was struggling with limited availability. Zok suggested that continuous-fiber ceramic composites, which are well suited for making airfoils, could be a very large market, but said that today’s additive manufacturing processes cannot achieve the multidirectionality needed to create reinforced structures.
O’Masta highlighted several key limitations of using ceramics, including their brittleness, porosity, and low damage tolerance. He said his team has been pursuing solutions to overcome these limitations in additive manufacturing for high-temperature applications. He also noted that fibrous reinforcements, which are highly desirable for toughening, have been shown to introduce defects, which must be mitigated for successful incorporation in 3D-printing platforms.
Compton suggested that direct ink writing additive manufacturing is a good match for ceramic composites’ behavior, with little linear shrinkage. Another participant pointed out that other, less brittle inorganics are also worth studying and suggested that one large market opportunity could be solid-state electrolytes to create flexible batteries.
Thomas asked if liquid-solid composites were possible, and Sigmund answered that tools already exist to optimize fluid and energy dissipation. Spadaccini added that mixing solids and liquids creates energy storage applications with different surface areas, which could have many applications. Kabaria agreed, noting that fluid capsules, which have a similar viscoelasticity as polymers, have a strain rate sensitivity that can be optimized for impact.
Designing for Elasticity, Fracture Toughness, Defect Sensitivity, and Sound Dynamics
Haydn Wadley, University of Virginia, marveled at the fracture and strain resistance of elastomeric lattices, but pointed out that stronger, less-ductile systems fracture more easily. Is it possible to use topology optimization to design multimaterial systems with built-in elasticity and fracture resistance? Kabaria answered that experiments by Zheng and Julia Greer, California Institute of Technology, show that some material structures can go through large strains relatively undamaged. Such a result would be especially promising if it could be accomplished with multi-materials, perhaps using an elastomer structure as a backbone. Spadaccini suggested such a structure could be possible with an infill process.
William Paul King, University of Illinois, Urbana-Champaign, asked if it was possible to tune the modulus for material distribution in multi-material structures to affect fracture toughness. Sigmund, who has worked on that very problem, replied that it is often a process of optimizing different material properties and working with the inevitable tradeoffs. For fracture toughness specifically, multi-materials should certainly be explored more. It also depends on how many material properties one is attempting to design for, he said. The more properties needed, the more likely multi-materials are the answer.
Zok pointed out that the inherent and varying defect sensitivity of certain topologies is also important. In addition, the role of free surfaces in initiating failure cannot be overlooked. The topology of lattice surfaces must be carefully designed to prevent failure, he noted.
Asked if sound dynamics, such as vibrations, could have sonar applications or address fatigue issues in materials systems such as helicopters, Sigmund replied that he has worked on sound damping, combining it with stiffness to create a structure with controlled resonances, waveguide-designed acoustics, and elastic waves.
Overcoming Modeling Difficulties
Watkins pointed out that most advanced materials and structures are very difficult to model, and therefore optimize, because of their inherent complexity. This, he said, makes hard problems even harder. He suggested that researchers could reduce the number of design variables or shift to studying reduced-order models in order to solve this intractable problem.
Kabaria agreed that solving nonlinear mechanics is very difficult. Reduced-order modeling may work, and Carbon is experimenting with several ideas from the literature. Commercial software may also have solved some of these simplified problems, but if the underlying physics are not properly understood, the optimization can fail. Until a model exists that truly works, it may be better to solve nonlinear mechanics to avoid incorrect and suboptimal solutions that would be a waste to manufacture, he said.
Pedersen replied that in his view, reduced modeling optimizations are often not robust for optimization iterations and perform even worse when nonlinearities are included. A simple, linear system is much easier, but does not translate to whole systems and not always to realistic detailed modeling.
Berger asked Kabaria if Carbon could eventually have enough modeling data such that a printed product would not require testing. Kabaria replied that the company is exploring computer-vision methods to capture failures. It is a difficult problem, he acknowledged, but solving it would reduce manufacturing and product costs.
Spadaccini shared that one LLNL goal is to instrument a machine with quality controls that can create “born qualified” products that require no testing. Kabaria agreed this was a worthy goal, and could be especially useful for additive manufacturing, although he noted that customization adds complications.