2
Flooding Processes and Environments on Alluvial Fans
FORMATION AND NATURE OF ALLUVIAL FANS
Alluvial fans develop where streams or debris flows emerge from steep reaches in which they are confined to relatively straight and narrow channels and flow into zones where sediment transport capacity decreases because of increases in channel width, reductions in channel gradient, or other influences. The channels on fans range from decimeters to several meters deep. These conditions develop at mountain fronts, in intermountain basins, and at valley junctions where there are major breaks in gradient or channel confinement, allowing both deposition of sediment and the lateral movement of channels to spread the sediment into a fan-shaped landform (Figure 2-1). Fan-formation is particularly favored where sediment loads are high, for example, in arid and semiarid mountain environments, wet and mechanically weak mountains, and environments that are near glaciers or active volcanoes. Deposition is particularly rapid where there is a reduction in the transport capacity of a heavily loaded stream.
Alluvial fans occur in a wide range of environments, including the western and eastern mountains of the United States, western Canada, and various mountain, arid, and volcanic regions around the world. In North America, most fans that have been subject to development are in the western mountainous regions. Fans occur in the Appalachian Mountains, but flooding on them has not yet been analyzed by FEMA because development pressure is not intense. However, minor local damage has occurred on some of these fans (Jacobson, 1993) and will no doubt increase as development pressure increases.
In the simplest cases of widely spaced stream or valley sources, fan geometry may be a sector of a simple cone emanating from a single, well-defined apex. In this simple case, a stream follows more-or-less a radial path down the cone, and the contours on the map of such a simple fan are convex downslope (Figure 2-1). Overall radial profiles are usually concave or virtually straight, and cross-fan profiles are convex. Where the sedimentary accumulations from several source areas encroach on one another, or where the deposition is forced by gradual widening or slope reduction along a valley, the simple conical fan-shape may not be easy to identify (Figure 2-2). Coalescence may lead to a general accumulation of overlapping fans along a mountain front, called a bajada (Figure 2-3). At their downstream margins, fans merge with the smoother depositional topography of valley floors, river terraces, and lake and coastal deposits, and the

FIGURE 2-1 Sketch of a simple fan with single source and no incision. Contours are convex downslope and closer together near the apex. The dashed lines represent channels that have not recently been invaded by water or debris flows. The solid, sinuous lines emanating from the apex indicate channels that have conveyed flows recently.
channels may be small, shallow, and diffuse. Fans and bajadas are different from pediments, some of which are cone-shaped, in that a fan-forms through deposition, whereas a pediment is a bedrock surface that is usually covered by a thin veneer of alluvium and colluvium.
Sediment may be transported to and across the fan by streamflow or debris flows. The latter are slurries with such high sediment-water ratios and concentrations of fine sediment that water cannot drain from them quickly enough to allow the sediment to settle out as traction load on the channel bed. Instead, the slurries travel at speeds of several to more than 10 meters per second (m/s) as dense viscous mixtures involving particle sizes from clay to boulders several meters in diameter.
Because the frequency, triggering mechanisms, size, and sedimentation processes of debris flows are so different from those of water floods, and the morphology and other clues about the nature of the flooding hazard on the respective types of fans are so radically different, it is necessary to distinguish between streamflow fans (Bull, 1977) and debris flow fans (Whipple and Dunne, 1992). Also, many fans are composites of stream and debris flow sediment. This chapter

FIGURE 2-3 Stylized view of a bajada (B) showing alluvial fans (A) merging with an alluvial plain (AP). The bajada is formed by coalescing alluvial fans originating at gullies cut in a dissected piedmont (P) and by debouching on the fan piedmont. Such eroded fan piedmont remnants commonly form the slopes above bajadas in the arid southwestern United States.
SOURCE: Adapted with permission from Peterson (1981).
emphasizes the differences by using the terms streamflow fan, debris flow fan, and composite fan, unless referring to a generic accumulation of any origin as a fan or alluvial fan. This distinction is not widely made in the literature, where all fans are usually called alluvial fans, but it is important because recognition of the nature of flooding and sedimentation processes on a fan and an understanding of the difference in triggering mechanisms and therefore probabilities of debris flows and floods are crucial to the accurate interpretation and prediction of flood risk.
The continuum of fan types reflects the range of sediment transport and depositional processes that generate and modify the landform. Sediment supply and transport mechanisms on fans include debris flow, channelized water flow and sheetflood (extensive, shallow overbank flooding of water or mud). There is no sharp line differentiating channelized flow, and sheetflood. Some fans exhibit all three transport mechanisms, with the frequency and importance of each changing down the fan.
There is also a continuum in the intensity of the sedimentation processes and therefore in the activity of the fan building and fan-modifying processes. Some fans are accumulating and changing rapidly under current climatic conditions; others are developing only slowly because changes in climate over recent millennia to centuries have caused their channels to deepen and stabilize. Still other fans have been subject to an intensification of flood and sedimentation hazard as a result of land use or engineering structures in the source area or on the fan itself.
Field evidence is an important source of information on the nature and intensity of the sedimentation processes that built the fan and is therefore critical for refining estimates of the nature, frequency, physical controls, and engineering significance of the flood risk on any fan. The morphology of the fan surface and the character of the deposits visible in the sides of channels indicate the relative contribution of water flows and debris flows to the flooding hazard on various parts of the fan. It can also be established whether changes in the governing geographical factors have changed the nature and distribution of the flood hazard during the history of the fan. It is important to realize that although fans vary in geometry and flooding characteristics because of the various combinations of their controlling factors, it is not always necessary to regress to a default assumption that there is no way to reduce uncertainty in the prediction of flooding processes on them. The copious field evidence available provides a means of reducing uncertainty about flood behavior, if it is properly interpreted.
Streamflow Fans
On fans that are actively forming from water-borne sediment alone, channels are usually braided, or multithreaded, from the apex. Deposition occurs on the channel bed in the form of bars on the margins or in the centers of channels. Rapid erosion of channel bed and banks is possible because of the loose, unconsolidated nature of the sediments. Thus, rapid erosion or deposition along a channel reach can alter the flow conveyance capacity during a single flood.
Bank erosion and lateral bar formation can force the channel to shift, while both bed aggradation and mid-channel bar formation can force water overbank and into new paths, so that channels divide and streams episodically abandon one or both channels. Channels may shift dominantly as a result of the accumulation of lateral bars, in which case they do not build up their bed or banks above the level of the surrounding surface. In addition to this gradual channel migration, sudden changes in flow path (avulsions) can occur due to overbank flooding. Even quite large and well-defined channels can be abandoned if a flood breaches one of the channel banks and water flows overbank in depressions between old bar deposits on the fan surface, often eroding a deep channel headward up to the source channel, which is then diverted. Particularly large, kilometer-scale changes in the positions of flow paths and active sedimentation zones can occur without the channel occupying or shifting across intermediate positions if the channelized and the overbank flow cause sediment to be deposited within and close to the channel, raising the bed and the channel margins above the surrounding fan surface (Figure 2-4). Breaching of the elevated banks in a large flood can allow the flow to travel toward the lower areas between channels or along the fan margins. Small shifts near the fanhead can cause dramatic changes in channel position farther down the fan.
As one proceeds down the fan, the channels separate more frequently than they join, so that on average the channels diverge and diminish in width, depth, and discharge along a general flow thread during any one flood event. Despite the decrease in discharge, the reduction in width, depth, and gradient can force water overbank in many floods, and thin, unchannelized, relatively uniform expanses of water can cover large areas (sheetflood). Sufficiently far down some fans, most of the runoff occurs as a sheetflood, either generated locally on the fan or forced overbank by the diminishing conveyance capacity of the channels. The sheetflood itself is irregular with zones of concentrated flow giving way downslope to divergences and shallowing at which small
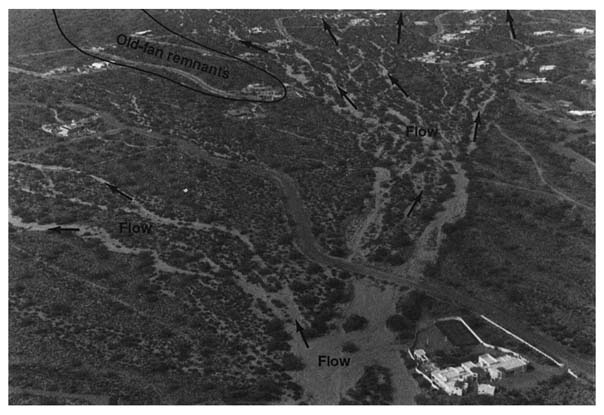
FIGURE 2-4 Oblique aerial photograph of an alluvial fan of the western slopes of the McDowell Mountains in central Arizona showing recent separation and braiding of channels downfan. The topographic apex is at the lower edge of the scene. Floodflow can inundate much of the area in the scene except for a few ridges of old fan remnants. Courtesy of H. W. Hjalmarson.
fans of sediment are deposited. At the toe of most fans, sheetflood that has a relatively low sediment concentration because of deposition on vegetated surfaces can once again gain an erosive capacity if it is concentrated into a number of swales and small channels before entering trunk streams that drain the entire mountain front.
On streamflow fans where the sediment balance has turned negative, either at present or for some period in the recent past, the channels are deeper because sedimentation on their floors and margins is replaced by incision. The flow and sediment conveyance capacity increase because form roughness is less in the absence of aggressive bar growth. Thus, many of these channels are incised below the surrounding fan surface, and avulsion occurs less frequently or not at all in the current climatic and hydrologic regime. The separation of the flow into diverging, smaller channels is reversed, and one or a few trunk streams convey the floodflow to the toe of the fan. Because these major conduits are incised they are not so frequently diverted by mid-channel bar deposits and they do not shift across the fan as quickly as those on actively accumulating fans. Instead, they tend to gather local runoff generated on the fan surface because the rills and small channels produced by such runoff repeatedly erode toward the stable trunk channel. The channel network is slightly convergent downfan, and mapped contours show upfan re-entrants that reflect
the incision. Some channel banks may be colonized by trees and bushes, adding another stabilizing influence. Because their surfaces are no longer accumulating sediment, such fans or parts of fans are said to be inactive.
Incised streamflow fans are particularly well-developed in regions where a major climatic change has altered the conditions that favored sediment accumulation (e.g., the transition from glaciation to interglacial period in parts of the Sierra Nevada and the Cascade Range of Washington state, or from wetter to drier periods in the mountains of Arizona). They are also well-developed in areas where fans have been steepened tectonically as in parts of southern California. In these cases, there is a strong isolation of deeply incised channels from the surrounding ''fossil" fan surface. Thus the problem of recognition is complicated because all degrees of isolation occur, ranging from aggressive accumulation to deep incision. Chapter 3 describes field methods for identifying and mapping degrees of activity and for dating the time of latest activity on various parts of fans.
Intermediate cases of channel stability and confinement are particularly widespread and important to recognize and evaluate. They occur, for example, in diverging channel systems (distributaries) where the sediment balance of a reach is near-steady state. Such channels may gradually become shallower downfan until their floodwaters simply disperse as sheetflood, repeatedly spreading thin layers of sediment and water and building an apron of relatively well watered and fine sediment that supports thick vegetation. In some years, there is accumulation of sediment, and in others there is net removal, so the bed may rise or fall by a few decimeters, but neither the scour nor the filling trend persists for long enough to raise or lower the channel bed significantly in relation to the fan surface.
Alternatively, there may be a persistent but very gradual trend that causes the channel to rise, lower, or shift laterally at a rate that is difficult to detect with commonly available information (e.g., sequences of maps or aerial photographs, anecdotal reports, dating of recent sediments with buried artifacts). In other cases, a reach that has stabilized may be perturbed by runoff or sediment that enters it from an unstable reach upstream. Thus analysis of the stability of a reach requires taking a broad view of the potential for change in channels upfan. Spatial context is important in any analysis of flooding and sedimentation hazards on a streamflow fan. Hjalmarson (1994) provides an illustrated account of various distributary-flow channels with a range of flow path stability and intensity of flood hazard.
Debris Flow Fans
Debris flow fans occur where strongly episodic sediment transport is triggered by collapse of an accumulation of weathered rock, soil, or sediment in a steep source region or by concentration of flow onto a steep accumulation of sediment that is then trenched rapidly in such a way that a high sediment concentration is developed with a mixture of sizes, including a significant proportion of fine sediment. The sediment-water ratio of the mixture must be so high that the flowing debris has a low permeability and water cannot drain out (upward) quickly enough to allow the water to separate from the sediment and the sediment to settle onto the bed.
The resulting poorly sorted slurry is dense and highly viscous and travels as a laminar flow except where agitated by waterfalls and cascades, by larger rocks in the bed, or by engineering structures. Observers often describe such flows as looking like wet concrete. Flows with
intermediate sediment-water ratios and characteristics between those of debris flows and turbid water flows are sometimes referred to as hyperconcentrated flows.
Debris flows consist of the full range of sediment sizes supplied from the source area, and flows generated from rocks of different types within the source basin may contain different proportions of clay. The greater the proportion of fines, the greater is the internal strength of the flow because of "cohesive" bonding caused by electrical charges shared between clays and water films. Some flows are sufficiently dense and viscous to transport boulders; others leave the largest boulders behind. As the sediment-water ratio decreases (i.e., in more dilute flows), progressively smaller boulders settle to the bed and are deposited or transported as traction load in a turbulent flow.
The flow properties of the slurries determine the fate of the debris flows when they emerge onto the fan, the nature of sediment deposition, and the resulting morphology of the deposit. These properties depend on the magnitude of the discharge and the rheological properties of the debris, which in turn are controlled by its sediment-water ratio and clay content. Discharge rate, clay content, and sediment-water ratio of each debris flow are set by the generating mechanism and the particular combination of circumstances that trigger the flow. For example, a large rainstorm or snowmelt may generate landslides that fall into stream channels containing significant discharge, and the resulting mixture may produce a dilute debris flow. Collapse of wet debris into a steep channel network that already contains a large volume of fallen debris from centuries of slow mass-wasting on adjacent hillslopes may result in scour of that accumulation into a particularly dense and viscous, boulder-charged debris flow. The volumes and peak flow rates of debris flows depend on (1) the magnitude of the water supplied from a rainstorm, snowmelt, lake outburst, or volcanic eruption, and (2) the volume of loose debris that is available to be liquefied by this water during the initial collapse, undermining and assimilation, or scour from the valley floor along the steep portion of the debris flow track. Thus, the debris flows that supply and mold any one fan have a probability distribution of discharges and rheological properties, which determine the nature and magnitude of flood risk. Fortunately, these aspects of flood risk can be read from the morphology of the fan and its source basin.
The range of rheological properties among debris flows emanating from the source valley usually accounts for differences in morphology on different parts of a single debris flow fan. Flows with the highest sediment-water ratios and therefore the greatest strength come to rest on relatively steep gradients (typically 6 to 8 degrees) on the upper parts of the fan in the form of bouldery snouts and levees. These deposits block channels scoured by water floods between debris flow episodes and divert later flows of water or debris into new channels. The result is a topographically rough surface of berms, lobes, and bouldery channel blockages on the upper parts of debris flow fans (Figure 2-5).
Somewhat more dilute and weaker flows travel through the steepest channel reaches, but deposit bouldery levees as their margins are slowed. If the peak discharge rate of a debris flow exceeds the conveyance capacity of the channel, its upper part is partially decanted overbank and it travels some distance across the fan surface until it becomes slow enough and thin enough to stop as a bouldery or gravelly sheet with a sharp edge. Stranding of boulders in levees and overbank sheets causes a progressive downfan reduction in the boulder content of flow deposits. The most dilute and weakest debris flows remain channelized as far as the lower parts of the fans, where gradients may be as low as 2 to 3 degrees. Some of these flows halt within the channel, raising its bed and lowering its depth, while others spread over the banks onto the surface of the
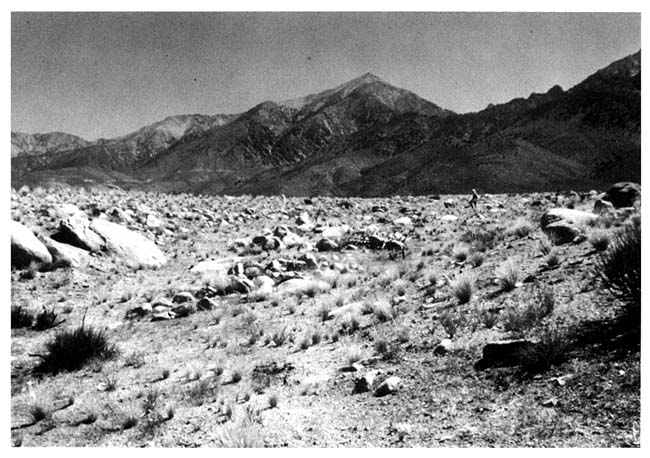
FIGURE 2-5 The upper part of this debris flow fan in Owens Valley, California, shows a classic rough, bouldery surface. Courtesy of T. Dunne.
fan as the declining gradient reduces the conveyance capacity of the channel, forcing flow overbank. The result is a smooth surface with only an occasional boulder on the lower parts of a debris flow fan. On debris flow fans, streams are often confined to nondiverging, boulder-lined channels left by the debris flows, and therefore they neither shift across the fan nor overtop the banks in most cases, except on the lower parts of the fan where shallow channels were originally formed by the dilute, low-viscosity flows described above. Of course, if the debris lining the channels is gravelly rather than bouldery, the capacity for channel shifting and eventual realignment by water floods is greater.
Many channels on debris flow fans are single-thread depressions blocked at their upper ends by bouldery accumulations, so they are never invaded by stream floods or debris flows. Like alluvial fans, debris flow fans are subject to varying amounts of deposition and parts or even much of the fan may be inactive under the present climate. For example, the debris flow fans emanating from the east side of the Sierra Nevada in the northwestern part of Owens Valley have more or less ceased to accumulate since the end of the last glaciation in the mountains, and the oldest parts of the fans date from previous glaciations. Parts of fans debouching from the unglaciated southern Sierra and from the White Mountains on the western side of Owens Valley continue to receive
debris flows in the modern climate. Descriptions of large debris flow fans in Owens Valley, California, are provided by Whipple and Dunne (1992), and smaller debris flow fans in a wetter environment are described by Kellerhals and Church (1990).
One approach to flood risk on debris flow fans concludes that even on active fans the probability of a debris flow is less than 1 percent in any one year, and therefore the "100-year flood" is not a debris flow but a runoff event. This is a generalization that fails to appreciate an important aspect of debris flow initiation, namely, that it is not an independent, random event in the same way that runoff floods are assumed to be. Debris accumulates in source localities and along stream channels over timescales from decades to centuries between failures that evacuate the debris (Benda and Dunne, 1987; Dunne, 1991; Reneau and Dietrich, 1991). Thus, a frequency count of dated debris flows in a region might indicate that the average frequency of occurrence is, say, 200 years per fan (with a probability of occurrence in any one year of 0.5 percent). However, if a geologist were to walk up any one of the source basins, he or she might find many potential failure sites and the channels below them to be occupied by thick layers of sediment that have accumulated since the previous debris flow occurred centuries earlier. In a neighboring valley, recent debris flows may have stripped such sediment from the valley and reset the clock so that the probability of debris flow is virtually zero for the foreseeable future. Thus flood risk estimates can be refined by first recognizing from field evidence that debris flows are the dominant sediment transporting agent on a particular fan and then examining the source basin to determine whether debris availability favors an enhanced risk of a debris flow in the event of a large rainstorm or snowmelt.
Composite Fans
Many fans are fed by both water floods and debris flows. Others were formed by debris flows under a different climatic regime and are now the sites of stream sedimentation and flooding only. Thus, both streamflow sediments and debris flow sediments and their associated morphologies attest to the nature of the flood risk on different parts of the same fan. The debris flow sediments are usually concentrated on the upper, steeper parts of the fans, producing a surface laced with berms, lobes, and channel plugs. The lower, streamflow part of the fan has the characteristics of an alluvial fan described above, although there may also be a contribution of dilute debris flow deposition on these distal areas. An indication of the relative contributions of debris flows and water floods can be obtained through systematic identification and mapping of the distribution of the two types of sediments on the fan surface and in vertical sections along the sides of channels.
Incised Channels on Fans
At the heads of some alluvial fans, channels are strongly incised in a fanhead trench, from which they emerge at some distance downfan to take on the character of a diverging braided channel network or a linear, boulder-leveed channel characteristic of debris flow fans, as described above. This report calls this point the hydrographic apex. Several reasons for the transition are identifiable in the field. The simplest case arises on a composite fan where episodic debris flow
deposition produces a gradient that is steeper than that required by intervening floods to transport the sediment load supplied by the source basin or produces sufficient channel bank strength to confine water flows to depths sufficient to transport the sediment supply. In this case, the water floods will scour away some of the debris flow sediment, establishing a lower-gradient channel incised within the debris flow deposits. At some distance down the fan, where the gradient of the debris flow sediment surface has diminished, the required stream gradient intersects the fan surface and a single-thread or braided channel or a swath of sheetflooding emerges from the fanhead trench at what Hooke (1967) called an intersection point, that is, a transition between flow and sediment transport process regimes.
In other cases, trenching at the fanhead or even over the entire fan may occur as a result of channel incision of older fan deposits, either because the sediment supply has diminished or because the transport capacity has increased owing to climate or vegetation changes within the source basin, or to tectonism. The roles of climatic change and tectonism in trenching the heads of fans are reviewed thoroughly by Bull (1991).
FLOODING PROCESSES ON ALLUVIAL FANS
Flooding on Streamflow Fans
Since streamflow alluvial fans typically occur in arid and mountainous environments, one of the first difficulties encountered in the quantification of alluvial fan flooding processes is the magnitude-frequency relationship for flows supplied to the apex. The sparseness of hydrologic monitoring stations in such regions and the shortness of most records render most estimates of probable flood discharges highly uncertain. Fans receive high water discharges from hurricanes or typhoons on the subtropical eastern sides of continents, and from more localized rainstorms or from intense, persistent snowmelt in mountainous western North America. In southern Europe, particularly in southeastern Spain, the most destructive discharges are again generated by rainstorms. In each of these regions, the history of hydrologic analysis and prediction has been one of surprises.
Stream flooding on alluvial fans differs from most riverine flooding in that the hazard not only derives from the inundation itself, but also is intimately connected with sedimentation processes. These latter have immediate impact during the flood itself, and they have long-term geomorphic influence through the rearrangement of sediment on the fan. High flood stages in channels are accompanied by high flow velocities, and by heavy loads of floating wood, and other debris in some environments. High velocities are promoted by the relatively steep, hydraulically simple nature of the channels. The flood hazard is markedly increased, however, by the potential for channel change during the flood itself. The loose bed material may be scoured several meters deep. On some fans the loose, unconsolidated nature of the sediments allows rapid channel widening by bank collapse if the flood persists for several hours or days. On others, the deposition of bars along a channel margin causes the channel to shift against the opposite, concave bank at rates of up to tens of meters per flood. Thus, rapid scour and filling of the channel cause changes in the channel conveyance capacity between and during floods.
The largest and most widespread threat arises, however, through the process of avulsion ("tearing away") in which water escapes from a channel by scouring a new path through the bank.
This process may begin by sudden bank collapse or by gradual overflow of water from the rising flood. As the relatively dilute surface water flows overbank, it often travels down a gradient that is steeper than the channel (because of the convexity of the fan cross section and the perched nature of some channels above the general fan surface) and so is able to pick up sediment and scour a new path. The water may also take advantage of a former channel, or a series of abandoned channel segments. In the short-term, this process may be easily predicted if there are obvious low sections of bank or narrow levees separating the channel from much lower parts of the fan. However, it is difficult to anticipate all such weak sections of the banks and to predict the exact flow path that the diversion is likely to follow across the irregular fan surface, especially since the diverted flow has the transport capacity to modify that surface. On the timescale of decades, it is virtually impossible, with either field inspection or mathematical modeling of sediment transport, to anticipate the locations of in channel deposition and bank erosion that might provoke avulsion. The problem is aggravated by the fact that a diversion on the upper part of the fan may alter flow paths on the lower part of the fan in ways that are independent of local fan morphology. Topographic changes in the channel network far upstream of a channel reach have the greatest potential for radically altering the risk of inundation, overtopping channel banks, or undermining a site downfan, but are probably the most difficult threat to anticipate and quantify.
Sheetfloods spread extensively on low relief lower parts of fan, and as they decelerate they often deposit sheets and low bars of sand or gravel. Even though velocities and depths are low, inundation by turbid water can be very destructive.
Flooding on Debris Flow Fans
Debris flows are dense (approximately 1.8 to 2.0 times the density of water), viscous (approximately 10,000 times the viscosity of water), and fast (3 to 10 m/s (9 to 30 feet/s)). They can transport boulders up to several meters in diameter, either as individuals supported in the matrix of the flow or as dams of boulders pushed along at the front. Some debris flows consist of waves of slurry behind bouldery dams (Sharp and Nobles, 1953; Suwa and Okuda, 1983). Large woody debris, engineering artifacts, and vehicles are also transported by debris flows and can because blockage, flow diversion, and extra damage to houses and other structures downfan. As they approach their final deposition point, debris flow sediments acquire a finite yield strength that prevents them from draining away like water. They remain as permanent covers on fan surfaces, and are expensive to remove from urban areas or channels. (On the other hand, however, they are less likely than water flows to undermine and destroy a road, so once cleared the road is generally still useable.) In extreme cases, such as after particularly large debris flows on fans near active volcanoes, deposits may be so thick and extensive that they permanently bury settlements. The deposits also block drainage in valley floors and at tributary junctions.
Some aspects of the prediction of debris flow-frequency and magnitude at the fan apex are more difficult than is the case for water floods, but other characteristics of debris flow occurrence simplify the problem. Within the United States, there are no monitoring stations with records long enough to provide a representative sample of debris flow occurrence on which a probability analysis might be based. A procedure commonly used by flood control agencies involves using records of runoff for prediction of a water flood peak with a 1 percent probability of occurrence,
and then increasing the volume of that predicted flood peak to account for the observed sediment-water ratio in a recent debris flow (which usually triggered the concern by surprising the agency in the first place) (Brunner, 1992). Although such a procedure might give a reasonable answer for those hyperconcentrated flows generated by runoff processes, it is wrong to mix in a probability analysis the results of runoff processes (gauging station records of floods) with debris flows, which are the usually triggered by some form of mass failure.
A particularly misleading situation arises when the assumption of interannual independence that has been found to be a useful approximation for rain-generated and snowmelt floods is applied to debris flow occurrence. This is because the occurrence of a debris flow in one year substantially reduces the probability of future debris flows by removing the sedimentary accumulations required for their generation and growth (Benda and Dunne, 1987; Keaton, 1988; Keaton et al., 1988). Fortunately, it is often possible to identify through field observations those conditions that favor the generation and growth of debris flows. For example, deep accumulations of colluvium on bedrock indicate a relatively high probability of debris flow occurrence in comparison to that in a basin in which most of the colluvium was evacuated in a relatively recent meteorologic event, after a forest fire, or after a climatic change. Thick accumulations of sediment along channels upstream of a fan indicate that no debris flow has passed for a considerable amount of time and therefore that the conditions are evolving toward a failure that could convey large quantities of sediment from canyon floors to the fan. Such observations combined with a probability analysis of rainfall or snowmelt required to trigger a mass failure are required for estimating the debris flow risk at the apex. Estimating the probable magnitude is more time-consuming, since it requires documenting volumes of sediment in old debris flow deposits or in the valleys above the fan.
Avulsions of debris flows occur on boulder-rich fans and are particularly difficult to forecast because of the uncertainty about the magnitude and rheology of the next debris flow. However, some clues to the likelihood of an avulsion occurring can be obtained from field inspection of the morphology and sedimentology of the fan itself. In particular, useful indications of the avulsion potential might be provided by (1) the volumes of sediment susceptible to liquefaction in the source area (Keaton, 1988; Keaton et al., 1988) and therefore the likelihood of a peak discharge great enough to overtax the conveyance capacity of the channel for such a debris flow; (2) previous blockage of the main channel by bouldery berms; (3) relatively low channel banks near the apex or in the vicinity of any blockage in the main channel; and (4) the topography of the fan surface at these locations. Calculations of the channel conveyance capacity for debris flows with a range of rheology can be made for various channel cross sections down the fan to judge the potential for overbank flow and spreading (Whipple, 1992; Whipple and Dunne, 1992). At the distal margins of debris flow fans, low-strength flows often spread widely in a manner similar to sheetflooding on streamflow alluvial fans (Figure 2-6).
A particularly hazardous situation arises on debris flow fans around active volcanoes because of the huge volumes of sediment that can be liquefied and the persistence of the liquefaction. For example, the October 1994 lahar (volcanic debris flow) generated by a typhoon from the slopes of Mt. Pinatubo in the Philippines deposited approximately 50 million cubic meters (1.8 billion cubic feet) on an urbanized and cultivated alluvial fan. The North Fork Toutle River lahar generated by the Mt. St. Helens eruption of May 18, 1990, deposited approximately 100 million cubic meters (3.5 billion cubic feet) of debris (Fairchild, 1985). Such volumes
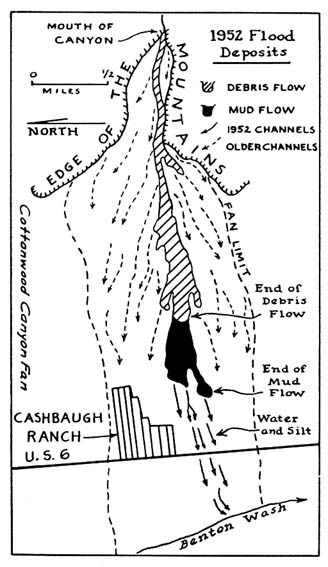
FIGURE 2-6 A 1952 debris flow flood on Cottonwood Canyon Fan, California. SOURCE: Reprinted with permission from Beaty (1963).
completely overwhelm the pre-existing channel system and topography of fans and create new pathways that are impossible to predict in detail.
Flooding on Composite Fans
Composite fans are subject to both streamflow and debris flow flooding. The relative importance of each varies between fans, between positions on each fan, between individual
meteorologic events, and between episodes as climate fluctuates or as debris accumulates slowly for centuries after a period of debris flow activity.
In general, debris flow activity is most frequent on the upper parts of a composite fan, but a large, dilute, and therefore weak debris flow may remain channelized and mobile for tens of kilometers, and therefore traverse the zone that is dominated by streamflow activity. However, through geologic mapping of distinct debris flow and alluvial deposits it is usually possible to define and map the probable nature of the various types of deposition (bouldery, lobate debris flow deposits; channelized debris flow deposits with bouldery levees; tabular sheets of debris flow deposits; bars of streamflow gravel; and sheets of gravely or sandy streamflow deposits) and thus the maximum extent of debris flows on a composite fan. After examining the debris flow generation potential of the source area, it is also possible to make an approximate calculation of the maximum conceivable transport of debris flows downfan. It is also possible through geologic mapping and dating of deposits and examination of source areas to determine whether the debris flow activity that may have built most of a fan is still active or will generate much smaller debris flows than during the period of intense fan building.
An important issue for the prediction of flood risk on fans affected by debris flow is that the rearrangement of the channel system by a debris flow can cause a long-term change in the flooding hazard during stream flooding. Furthermore, after a debris flow has deposited large volumes of sediment on the upper parts of a fan, streamflow in the same or later events will spread the sediment far downfan, causing channel instability and sedimentation that reduce the flood conveyance capacity of channels.
CHANGE OVER TIME
Fans form over thousands to millions of years, during which time environmental conditions affecting their formation change more or less continuously. For this reason, they are always evolving, although parts (reaches) of them may temporarily attain a steady state in which their channel gradients can pass the supplied sediment and no further deposition occurs in that reach. Even in that state, the channels can shift across the fan and remold its surface by erosion and deposition.
However, part or the whole of the fan may become a zone of greatly diminished accumulation or in the extreme case may become a zone of net erosion with the entire channel system entrenched. The fan surface may thus be weathering and eroding, or it may have attained a steady state after a period of incision. However, some runoff and channel patterns develop on the fan after it ceases to be a zone of net accumulation.
Changes in the sediment balance and its spatial distribution can occur because of (1) externally imposed changes (e.g., of climate or land cover influencing sediment supply and transport capacity or of tectonism influencing channel slope and therefore transport capacity); (2) long-term evolution of the fan-shape (especially its gradient) as it evolves over time in response to the sediment accumulation; and (3) shorter-term changes in form due to sediment accumulation (e.g., channel shifting accompanying bar accumulation, channel avulsion, raising of channel above the surrounding surface, deposition and channel filling by a single debris flow, runs of wet years caused by weather fluctuations, which may cause accelerated channel widening or shifting or reactivation of sediment sources producing debris flows that have been dormant for a long time).
Even if the fan is still a zone of active accumulation, the locus of that deposition may vary between fans or on the same fan during its lifetime. Distributaries develop most frequently where aggradation is intense and thus may shift the centers of active sedimentation across or along the fan. Deposition also may shift down the fan if the uplift rate in the mountain source exceeds the deposition rate at the fanhead. This can cause trenching of the source canyon and the upper fan or intense episodes of debris flow and stream transport associated with increased runoff. In the latter cases, the channel may be entrenched below the upstream part of the fan surface and all deposition and attendant channel shifting or change occurs on the lower portions of the fan. Appreciation of such changes is important for understanding flood hazards on fans because (1) some of the changes occur sufficiently quickly to affect channels on timescales relevant to engineering analyses, so that it is important to know whether a particular fan is undergoing a change at the present time, and (2) geologically recent, but no-longer active, processes may leave a morphologic signal on the fan or in the source basin that must be interpreted in order to refine estimates of recent changes in flood hazard.
Fans grow in a variety of ways and the thickness generally increases at the slope transition formed at the fan toe as it is progressively buried (Hooke and Dorn, 1992). Deposits near the apex commonly are remobilized and redeposited farther down the fan on and below the fan toe. French (1995) gives a method of estimating the depth of sediment deposition at these slope transitions on fans. The most permanent deposition typically begins at the toe and propagates both up the fan and below the toe where the slope typically diminishes.
The evolution of the fan surface causes a difficult problem for the interpretation of field evidence concerning alluvial fan flooding and for the prediction of future flood risk. For example, if a part of a fan surface has not been disturbed by flooding or erosion for 15,000 years, its surface will have become weathered and covered by a soil-profile and vegetation (described in Chapter 3). The surface of such a fan will be very different from the surface of a nearby channelized and actively evolving area. An important geomorphologic and hydrologic question for flood risk analysis is whether the older surface has evolved out of the flood zone or whether it simply has not been flooded for 15,000 years because random channel migration across the fan took the locus of flooding and sedimentation far from the site for that length of time.
If, for example, the active sedimentation zone is now migrating into the older surface through lateral bank erosion, or lies at an elevation only a few meters below that surface (i.e., within the range of flood stage), or if upstream of the site there is an opportunity for avulsions that could lead channels or sheetfloods across the older surface, then the older surface lies within the zone subject to alluvial fan flooding.
If, by contrast, channels have become entrenched during the past 15,000 years for one of the reasons given above, the elevation difference between the recently active sedimentation zone and the older surface may be greater than any flood or debris flow stage conceivable in the current regime of climate, hydrology, or land use in the source area. In this case, "overbank" flooding is not possible. Maximum conceivable flood heights, albeit approximate because of the assumptions that must be made about potential changes of bed elevation, are predictable through methods described by Burkham (1988) for floods and Whipple (1992) for debris flows. If field inspection reveals that the margin between the older and the recently active surfaces is armored with bouldery or cohesive sediment, and if the ages of trees along this margin as well as the aerial photographic record (now approximately 50 years old) indicate little or no migration of that boundary, then one's confidence is increased that lateral bank erosion does not threaten the site.
Such evidence is found, for example, along many channelized alluvial fans that have been entrenched and rendered less active by changes of climate and hydrology. Former debris flow activity, or large meltwater or monsoonal Pleistocene floods, have often built fans of coarse sediment that were trenched by smaller floods soon after the climate change and now have channels that can contain all the stream discharges conceivable under current environmental conditions and are lined with sediment too resistant to be eroded rapidly. More equivocal conditions occur along channels that have undergone less extreme changes, but photographic and dendrochronological evidence indicates that channels on some, even fine-grained, fans do not aggressively undermine their root-reinforced banks, presumably because bank scour is not required by the combination of flood lows and sediment balance in the reach. Finally, even if the older surface is judged to be free from the risk of overbank inundation and undermining, the channel system upfan needs to be systematically surveyed for the potential of an avulsion that would lead flows across the older surface. This would require a combination of geomorphologic survey (for low banks, elevated channels, zones of sediment accumulation, bank erosion and channel shifting, and topographic lows on the fan surface) and calculations of the heights of conceivable floods and debris flows. On many alluvial fans, however, there is a relatively clear separation between older, higher, stable parts of fans, and channels or narrow inset floors trenched into the fan deposits.
The kinds of field evidence that can be used for making such determinations are reviewed in Chapter 3, and valuable sources of information are listed in Appendix B. It is unfortunate that the problem of interpreting the significance of different ages of fan surface for alluvial fan flooding risk is often not reduced by a simple indicator or numerical index. However, with a systematic approach such as the example given above (identify the risk of overbank inundation, lateral bank erosion, and inundation by avulsion from upstream), most field situations can be classified as a basis for both regulation and choice of a method for estimating flood risk, as required by FEMA. Complicated situations, however, will tax any approach.
REPORTS OF FLOODING ON ALLUVIAL FANS
Documented accounts of flooding confirm that active fans function primarily as a terminus for water and debris, while relict fans and streams function to convey water and sediment. Appendix A summarizes accounts of flooding at 29 individual or groups of alluvial fans to illustrate a wide variety of flood conditions on streamflow, debris flow, and composite fans. Most of the alluvial fans are in the arid southwestern United States, and a few are in humid areas throughout the world. The accounts are for observed floods and consist of direct and indirect measurements and observations of flood characteristics and geomorphologic interpretation of flood remnants. Only a few maps of the extent of flooding are available, and direct measurements of flow depths and velocities on fans are rare. Opportunities for systematic collection of flood data on alluvial fans are also rare.
These accounts demonstrate the complex nature of flooding on alluvial fans, ranging from flooding in stable channels to massive deposition of debris in urbanized areas. Accounts by different observers of a particular flood on a particular fan can depict different characteristics. This is because (1) observations from a single vantage point of the widespread and variable nature of fan flooding are limited and not desirable and (2) local conditions change during a flood
because of the rearrangement of the channel system by remobilization of deposited debris, as described in the previous section. Also, the flood characteristics on adjacent or nearby fans resulting from the same storm commonly are different because of local differences in rainstorm intensity, sediment availability in the source areas, and the respective differences in recent flow path history.
Although flooding accounts depict varied properties in time and space, they show certain distinctive properties when considered collectively. High floodflow velocities were reported for about half of the sites listed in Appendix A. Supercritical flow velocities for water floods are also suggested at other sites by the steep fan slopes and low hydraulic roughness associated with fine-textured surface sediments and simple channel geometries. Flash floods (short-lived floods with a short time to peak) are reported at eight sites, including accounts of translatory waves at the Chicago Creek, British Columbia; Horseshoe Park, Colorado; and Montrose, California, alluvial fans. At the Cottonwood Canyon, Magnesia Spring Canyon, and Montrose alluvial fans in California the reported combination of high flow velocities, flashy flow, unstable channels, and movement of flow paths produced an especially serious hazard that resulted in loss of life at two of the fans.
Sheetflooding and distributary-flow also are typical of alluvial fan flooding. Unstable channel boundaries are common, while stable channel beds or banks are less frequent, as suggested by accounts at only 5 of the sites studied. Flow path movement was reported for 8 sites, no movement for 13 sites, and either no mention of movement or absence of movement at the remaining 8 sites. A possible reason for the lack of flow path movement and disruption of the surface of some of the active fans is the short duration of the floods in concert with a limited supply of unconsolidated medium to coarse material from the drainage basin. Typical distributary channels appear to scour and fill; however, stable flow paths do occur during individual floods.
The Carefree and Wild Burro alluvial fans in Arizona are examples of less active or inactive fans with some channel incision and a limited supply of medium-to-coarse sediment from the drainage basin. Most of the sediment delivered to the fans is carried by streamflow and is coarse sand and fine gravel. The basins are not very steep. For example, the basin of the Carefree fan is a pediment. On these fans the channels are slightly trenched below the surrounding fan surface and are lined with desert trees and shrubs. During recent major floods, there was no movement of the many distributary channels on either of these fans.
Flood hazards on the least active fan surfaces, such as the Carefree (see Chapter 4) and Wild Burro alluvial fans, can be deceptively small because of the small size of distributary channels and the rather long distances from mountainous drainage basins. For example, shallow floodflow unexpectedly entered several small defined channels during a major flood on the Saddle Mountain, Arizona, alluvial fan (USACE, 1993). A subsequent field investigation revealed no new channel formation on the fan. Rather, small distributary channels that in places appeared to be simple topographic lows conveyed overflow from the larger distributary channels or carried runoff from high-intensity rainfall directly on the fan surface. Many less active alluvial fans in the arid southwestern United States have developed soils with small, slightly incised stable flow paths that convey shallow high-velocity floodflow during major floods.
Flood control works such as levees and debris dams at several of the sites were partially or totally ineffective during major floods. For example, at Day and Deer creeks (Figure 2-2), Henderson Canyon (see Chapter 4), and Magnesia Spring Canyon alluvial fans in California, the flood control structures were overwhelmed, and floodwater followed original flow paths and fan
topography at and below sites of structural failure. This suggests that (1) major flood control works are necessary to mitigate flood hazards on active alluvial fans, (2) predevelopment fan topography influences the location of major flooding even after fans are urbanized and minor flood control structures are in place, and (3) flood control works must be designed to address specific types of hazards and special design consideration should be given for areas where water can still reach after flood control structures are installed.
The small Glendora alluvial fan is highly urbanized, and the paths of floodwater for a flood in January 1969 were significantly controlled by the network of streets that cross the fan parallel and at right angles to the general direction of fan slope (Figure 2-7). The alluvial fans of Cottonwood Creek and Stuart and Crane Gulches are also highly urbanized, and the paths of floodwater are significantly controlled by the conveyance capacity and location of many streets. A FIRM showing alluvial fan flooding hazards based on the assumption of uniform risk, thereby ignoring the channels created by the streets, would be inappropriate for these urban areas.
The Horseshoe Park and Wadi Mikeimin alluvial fans each were formed during a single flood. The Horseshoe Park alluvial fan was formed by a catastrophic flood from a dam failure. The fan-formed at a topographic break at the mouth of the Roaring River. Wadi Mikeimin also formed at a break in floodflow confinement at the mouth of a river. The Roaring and Mikeimin River fans were significantly modified by erosion following the fan building floods.
Abnormally large volumes of sediment were produced by storm runoff from recently burned basins of the Montrose, Cottonwood Creek, and Wasatch front alluvial fans. The close proximity of these fan basins to urbanizing areas may result in a greater incidence of wildfires with associated debris flows and alluviation on urbanized fan surfaces.
Reported flood characteristics of the sample of fans include high-velocity water flows, debris flows, translatory waves, sheetflood, distributary-flow, unstable and stable channel boundaries, movement of flow paths, stable flow paths, and alluviation on the unchanneled fan surface. The composite of these accounts of flooding shows a wide variability of processes and flood hazard in time and space, which places a premium on field inspection and interpretation of concrete evidence from each alluvial fan before a determination is made of flood risk.
REFERENCES
Beaty, C. B. 1963. Origin of alluvial fans, White Mountains, California and Nevada. Annals of the Association of American Geographers 53:516–535.
Benda, L., and T. Dunne. 1987. Sediment Routing by Debris Flow. Publication. 165. Oxford, England: International Association of Hydrological Sciences.
Brunner, G. W. 1992. Numerical simulation of mudflows from hypothetical failures of the Castle Lake debris blockage near Mount St. Helens, Washington, Appendix A: Derivation of the equation to calculated the ultimate bulking factor. In Program for Steep Streams Workshop, Seattle, Washington, October 27–29, 1992. Portland, Ore.: U.S. Army Corps of Engineers.
Bull, W. B. 1977. The alluvial fan environment. Progress in Physical Geography 1(2):222–270.
Bull, W. B. 1991. Geomorphic Responses to Climate Change. New York: Oxford University Press.
Burkham, D. E. 1988. Methods for delineating flood-prone areas in the Great Basin of Nevada and adjacent states. U.S. Geological Survey Water-Supply Paper 2316. Reston, Va.: U.S. Geological Survey.
Dunne, T. 1991. Stochastic aspects of the relations between climate, hydrology, and landform evolution. Transactions, Japanese Geomorphological Union 12:1–24.
Fairchild, L. H. 1985. Lahars at Mount St. Helens. Ph.D. dissertation, University of Washington, Seattle.
French, R. H. 1995. Estimating the depth and length of sediment deposition at slope transitions on alluvial fans during flood events. Journal of Soil and Water Conservation 50(5):521–522.
Geissner, F. W., and M. Price. 1971. Flood of January 1969 Near Azusa and Glendora, California. U.S. Geological Survey Hydrologic Atlas HA 424. Reston, Va.: U.S. Geological Survey.
Hjalmarson, H. W. 1994. Potential flood hazards and hydraulic characteristics of distributary-flow areas in Maricopa County, Arizona. U.S. Geological Survey Water Resources Investigations Report 93-4169. Reston, Va.: U.S. Geological Survey.
Hooke, R. L. 1967. Processes on arid-region alluvial fans. Journal of Geology 75:438–460.
Hooke, R. L., and R. I. Dorn. 1992. Segmentation of alluvial fans in Death Valley, California, new insights from surface exposure dating. Earth Surface Processes and Landforms 17:557–574
Jacobson, R. B. 1993. Geomorphic studies of the storm and flood of November 3–5, 1985 in the Upper Potomac and Cheat River basins in West Virginia and Virginia. U.S. Geological Survey Bulletin 1981. Reston, Va.: U.S. Geological Survey.
Keaton, J. R. 1988. A probabilistic model for hazards-related sedimentation processes on alluvial fans in Davis County. Ph.D. dissertation. Texas A&M University, College Station.
Keaton, J. R., L. R. Anderson, and C. C. Mathewson. 1988. Assessing debris flow hazards on alluvial fans in Davis County, Utah. Final report for U.S. Geological Survey Landslide Hazard Reduction. Agreement No. 14-08-0001-A0507. Reston, Va.: U.S. Geological Survey.
Kellerhals, R., and M. Church. 1990. Hazard management of fans, with examples from British Columbia. Pp. 335–354 in Alluvial Fans: A Field Approach, A. H. Rachocki and M. Church, eds. New York: John Wiley & Sons.
Peterson, F. F. 1981. Landforms of the Basin and Range Province: Defined for Soil Survey. Technical Bulletin 28. Reno: Nevada Agricultural Experiment Station, University of Nevada.
Reneau, S. L., and W. E. Dietrich 1991. Erosion rates in the Southern Oregon Coast Range: evidence for an equilibrium between hillslope erosion and sediment yield. Earth Surface Processes and Landforms 16:307–322.
Sharp, R. P., and Nobles, L. H. 1953. Mudflow of 1941 at Wrightwood, Southern California. Geological Society of America Bulletin 64:547–560.
Singer, J. A., and M. Price. 1971. Flood of January 1969 Near Cucamonga, California. U.S. Geological Survey Hydrologic Atlas HA-425. Reston, Va.: U.S. Geological Survey.
Suwa, H., and S. Okuda. 1983. Deposition of debris flows on a fan surface, Mt. Yakedake, Japan. Zeitschrift fur Geomorphologie Supplement Band 46:79–101.
U.S. Army Corps of Engineers (USACE). 1993. Assessment of Structural Flood Control Measures on Alluvial Fans. Davis, Calif.: Hydrologic Engineering Center.
Whipple, K. X. 1992. Predicting debris flow runout and deposition on fans: the importance of the flow hydrograph, erosion, debris flow and environment in mountain regions. Pp. 337–345 in Proceedings of the International Association of Hydrologic Sciences Symposium, D. Walling, T. H. Davies, and B. Hasholt, eds. IAHS Publication 209. Oxford, England: IAHS Press.
Whipple, K. X., and T. Dunne. 1992. The influence of debris flow rheology on fan morphology, Owens Valley, California. Geological Society of America Bulletin 104:887–900.