5
Effect of Metabolic Modifiers on Nutrient Requirements of Growing Swine
The productive efficiency of swine raised for pork production is determined, to a large extent, by the proportion of nutrients used for fat versus muscle accretion. Nutrient partitioning is influenced by genotype, gender, hormones, and stage of growth as well as by feeding management. Recent advances in biology have made it possible for animal scientists to dramatically alter growth and development patterns by modifying the animal's metabolism. Porcine somatotropin (pST) and β-adrenergic agonists (β-agonists) are metabolic modifiers that have allowed scientists to investigate and modify how farm animals use absorbed nutrients for growth. Unprecedented responses in protein and lipid accretion have been reported (Campbell and Taverner, 1988; Evock et al., 1988; Boyd and Krick, 1989).
The availability of metabolic modifiers presents an important nutritional challenge because each metabolic modifier differs in its mode of action, and these differences can result in considerable variation in the rates of lean tissue deposition. This suggests that a systematic method for determining nutrient requirements must evolve to accommodate a widening range of biological and nutritional situations. Therefore, any experimental or empirical attempts to estimate a particular nutrient requirement should contribute quantitative information and knowledge of biological principles such that mathematical relationships could then be used in dynamic, computer models (factorial approach).
In this chapter somatotropin (ST) and β-agonists are discussed in the context that their use may change intermediary metabolism of treated pigs such that nutritional requirements might be altered. A systematic approach is possible with pST because the data are extensive and accommodate a dynamic, computer simulation approach. Little quantitative information is available about the effects of β-agonists on nutrient requirements, but there are some potentially important differences when β-agonists are compared with somatotropin. Care is taken to illustrate how the experimental approach in estimating nutrient requirements can simultaneously accommodate the objectives of estimation and definition of mathematical relationships. Emphasis will be placed on dietary protein (amino acids) and energy because the available information almost exclusively addresses one or both of these nutritional entities.
NUTRITIONAL IMPLICATIONS OF METABOLIC MODIFIERS
The growth response to metabolic modifiers is best illustrated with pST because both treated and untreated pigs have been well characterized. The effects of pST administration on growing swine have been documented for two phases of growth—growing and finishing—in which intrinsic differences exist for protein and lipid accretion (Boyd et al., 1991; see Table 5-1). Although pST is effective in diverting nutrients away from lipid accretion and stimulating protein accretion during the early growth phase (approximately 20 to 50 kg body weight), the greatest relative response has been observed during the later growth stage or finishing phase (approximately 50 to 100 kg body weight). The biological basis for this is unclear but the potential impact on nutritional requirements is most evident for the finishing phase of growth. First, the effect of pST on depressing lipid accretion (grams per day) is much greater than the increase in protein accretion (grams per day) (see Table 5-1) which, overall, results in a lower caloric gain and, concomitantly, a decline in energy intake. This implies that the nutrient-to-energy ratio must increase to ensure a constant intake of nutrients. Second, an increase in the rate of protein deposition requires increased daily amino acid intake, and an increase in the proportion of total body protein requires an increase in total amino acid intake. The extent to which this is true, however, depends on whether pST alters a pig's ability to digest and absorb amino acids and/or its metabolic efficiency in using absorbed amino acids for
TABLE 5-1 Responses of Swine Administered Porcine Somatotropin (pST) during Two Phases of Growth
|
Growing Phase (20–50 kg BW) |
Finishing Phase (50–100 kg BW) |
||||
Variable |
Control |
pST |
% Change |
Control |
pST |
% Change |
Protein accretion, g/daya |
120 |
150 |
25 |
135 |
235 |
74 |
Lipid accretion, g/daya |
207 |
122 |
-41 |
340 |
60 |
-82 |
Ash accretion, g/daya |
21 |
25 |
19 |
16 |
26 |
62 |
Muscle yield, kg |
— |
— |
— |
31.4 |
42.8 |
36 |
Fat yield, kg |
— |
— |
— |
23.2 |
6.0 |
-74 |
Growth rate, g/day |
900 |
990 |
10 |
1,140 |
1,330 |
17 |
Growth efficiency, F:G |
2.3 |
2.0 |
-13 |
3.0 |
2.0 |
-33 |
DE intake, Mcal/day |
7.3 |
6.7 |
-8 |
13.3 |
10.2 |
-23 |
NOTE: Animals used were females and castrate males (8 to 10 per treatment) fed diets adequate in protein (Krick et al., 1990, 1993). The pST dose used (150 µg pST/kg BW/day) maximized protein deposition. DE, digestible energy; F:G, feed to gain ratio; —, not available. a Whole-body deposition rates determined by comparative slaughter. Source: Boyd et al. (1991). |
protein accretion (called biological value or partial efficiency). In addition, the mineral content of diets must be considered because dramatic changes have been observed for ash accretion rate (Caperna et al., 1989; Bark, 1990). The potential impact, however, on the requirement for vitamins is not predictable from available data.
The need for a more dynamic approach for estimating amino acid requirements and perhaps other nutrients is suggested by data presented in Table 5-1 and Figure 2-2. This information shows that marked differences in nutrient deposition do occur between the early growth and the finishing phase. Further, the response to pST will vary markedly depending on the dose used (see Figure 2-2). Nutrient requirements are generally derived experimentally by relating a physiological response to increments of nutrient input under specific conditions. However, animals are often inadequately characterized beyond age, weight, gender, and genotype. For example, protein accretion rate is seldom specified even though this clearly determines the requirement for a particular amino acid. The factorial approach is a more dynamic way to estimate the amino acid requirement because factorial estimates are not limited to conditions under which they were derived. Thus, factorial estimates are more amenable to changes that occur in genetic base, gender, and technological advancements such as metabolic modifiers. However, an understanding of biological principles and experimentally derived mathematical relationships is required if factorial estimates are to be trusted. A more systematic biological and mathematical view of requirement estimation must be undertaken so that conditions for which the requirement are relevant are better defined and accommodating of such a dynamic status.
Data in Table 5-1 indicate that the rate of protein accretion is considerably below the inherent, genetic capacity and suggests that endogenous secretion of pST in nontreated pigs is not sufficient to support the rate of protein deposition that can be attained. The potential for protein deposition is best illustrated by the elite responders (top 10 percent), which deposited 250 to 270 g/day of protein when treated with pST. This rate of protein accretion, with castrate and female pigs, exceeds previous estimates for intact males by approximately 30 percent (Campbell, 1987; Campbell et al., 1990a), negating the advantage typically acknowledged for the intact male. Thus, the estimated biological potential for protein accretion in finishing phase pigs is at least 270 g/day. The net effect of this alteration was an unprecedented efficiency of growth (feed: gain approximately 1.8) coincident with an equally impressive rate of gain for intact male pigs of an elite genotype that were highly selected for lean tissue deposition (>1,500 g/day; Campbell et al., 1990a). The potential for efficiency of growth in this example rivals commercial broiler chickens.
NUTRITIONAL CONSTRAINTS TO LEAN GROWTH
Although the capacity for protein accretion is determined by genotype, gender, and stage of growth, it is well known that extrinsic or environmental factors such as inadequate intake of either protein (amino acids) or energy may limit the potential for lean tissue deposition. The interrelationship between protein and energy intake and protein deposition has been studied in several species, but data are most extensive for growing swine (Black et al., 1986; Campbell, 1987; Dunkin, 1987). One must assume that metabolic modifiers likewise operate within this framework, until data on biological mechanisms suggest otherwise. Failure to address these nutritional considerations may limit the biological response to metabolic modifiers.
The interrelationship between protein accretion and protein and energy intake is illustrated in Figure 5-1A. In the protein-dependent phase, protein deposition increases in a linear manner with each increment of dietary protein intake. Whether the animal is able to reach its potential for protein deposition depends on protein adequacy and also the availability of sufficient dietary energy. For example, if pigs are fed increasing amounts of protein, in conjunction with a fixed amount of energy (E1), protein deposition increases to an apparent maximum (M2). Additional increments of protein will not result in a further increase in protein accretion because of an energy constraint. However, when this energy restriction is removed (E2), protein deposition increases to a new maximum (M1) allowable by protein intake. M1 protein accretion would represent the genetically determined maximum, if energy and protein intake are both adequate. Thus, the protein (amino acid) requirement is a function of the level of the protein deposition allowable by energy consumed. Unfortunately, M 1 and M2 are seldom experimentally defined even though they are important factors in accounting for differences in the protein (amino acid) requirement at a particular energy intake.
An energy limitation is less likely to occur with pigs weighing more than 50 kg because energy intake generally exceeds the expressed capacity for protein accretion (3.5 to 4.0 times the maintenance energy requirement) with ad libitum feeding. In contrast, energy intake may constrain protein deposition for pigs weighing less than 50 kg and perhaps for genetically elite intact males weighing more than 50 kg. The apparent disparity between pigs in early and late stages of growth may be the result of the fact that an inverse relationship exists between nitrogen deposition per unit metabolic weight and body weight (Carr et al., 1977).

FIGURE 5-1
Interrelationship between protein deposition and protein (amino acid) intake in swine. A: Protein deposition under restricted energy intake (E1) results in an apparent maximal protein deposition (M2); but when energy intake is not limiting (E2), a higher maximal protein deposition (M1) can be achieved. B: Effect of protein biological value (BV)—the solid line represents the intake of protein with an ''ideal" amino acid pattern for protein deposition; the dashed line represents the response to a protein with an inferior biological value.
The effect of protein quality on the dietary protein requirement for a given level of protein deposition is illustrated in Figure 5-1B. The slope of the linear component is determined by the digestibility of dietary protein, the extent to which the amino acid pattern accommodates the tissue requirement (i.e., "ideal" amino acid pattern; Agricultural Research Council, 1981; Taverner, 1987; Wang and Fuller, 1987) and the extent to which absorbed amino acids are partitioned to protein deposition (i.e., biological value). This coefficient is a measure of net protein utility, which in turn determines the quantity of dietary protein required to support a given rate of protein accretion. Any metabolic modifier that enhances protein deposition without altering the efficiency of protein digestion and/or partitioning of amino acids to protein deposition could do so only if there were a concurrent increase in dietary protein intake.
The relationship between energy intake and protein deposition must be precisely defined for both nontreated pigs and pigs treated with metabolic modifiers in order to differentiate between the animal's tissue requirement for protein (M1, M2) and the capacity of different dietary regimens to satisfy this tissue requirement (e.g., restricted versus ad libitum feeding). For example, a program of energy restriction attempts to accommodate maximum lean tissue accretion while minimizing fat deposition. This principle is illustrated in Figure 5-2, which relates the pattern of muscle and fat deposition to energy intake for pigs in the 50 to 100 kg body weight range (Whittemore, 1986). Expression of amino acid requirements in relation to a defined optimum for energy intake [e.g., grams of lysine per Mcal of digestible energy (DE) intake] is appropriate when energy is the ultimate determinant of protein accretion. Concomitantly, this ratio accommodates the dynamics of feed intake conceivable

FIGURE 5-2
Hypothetical model originally presented by Whittemore (1986) showing the relationship between dietary energy intake and deposition of muscle and fat tissues. Point A represents restricted energy intake to the level just required to maximize protein deposition. Point B represents ad libitum feed intake.
with restricted feeding and the consequent effects on tissue protein deposition. Conversely, with ad libitum energy intake, the tissue requirement for amino acids is independent of energy intake so that the relevant expression of dietary needs is grams per day of a particular amino acid.
EFFECT OF METABOLIC MODIFIERS ON NUTRIENT REQUIREMENTS
Consideration of how nutrient requirements are altered by metabolic modifiers and other new technologies requires a systematic approach to quantitatively define how each of the components of nutrient use is affected. This has been fully described in a recent review by Boyd et al. (1991). The essential components are (1) digestibility, (2) rate and composition of gain (i.e., nutrient deposition), (3) efficiency with which absorbed nutrients are used for tissue deposition, (4) the quantity of nutrients required for maintenance, and (5) level of feed intake. The impact of metabolic modifiers on amino acid requirements and allowable energy intake is discussed within this framework and in the context of ST because these data are most extensive. Particular emphasis is placed on experiments that reveal both biological principles and mathematical relationships.
Any strategy that increases ST concentration in the blood would be expected to affect the pattern of nutrient deposition. Given the current technologies and our understanding of ST biology, there are at least four conceivable approaches to manipulating the ST axis, which have been discussed in recent reviews (Boyd and Wray-Cahen, 1989; Campion and Novakofski, 1990). Also, the argument for a more dynamic approach for estimating nutrient requirements is well illustrated with exogenous administration of pST where the magnitude of response has proved to be a function of dose (see Figure 2-2), gender (Figure 5-3), and genotype (Campbell et al., 1990a; Krick et al., 1992). Thus, information from the pST data base has established the precedent to adopt a more dynamic method for estimating nutrient requirements. This has implications with respect to experimental design considerations and the type of information collected.
Intake
A consistent effect of pST treatment is reduced energy intake (see Figure 2-2 and Table 5-1; see also Table 5-2). Definition of the energy-allowable intake is important under conditions of ad libitum feeding because the nutrient requirement must ultimately be related to expected voluntary intake to ensure that the daily nutrient requirement is met. The extent to which energy consumption is altered, however,

FIGURE 5-3
Differential response of female and intact male pigs to pST treatment and dietary energy intake (Campbell et al., 1991). Pigs (between 60 and 90 kg) were treated daily with pST (90 µg/kg body weight) or excipient. Six levels of energy intake were used that ranged from 5.08 Mcal DE/day to ad libitum. Protein intake was 12 percent above that determined as adequate in a previous study involving intact males of the same genotype (3.7 g lysine/Mcal DE) and level of pST. Carcass protein deposition rates were determined by comparative slaughter.
TABLE 5-2 Factorial Estimation of the Dietary Protein and Lysine Requirements for Control and Porcine Somatotropin (pST)-Treated Pigs (50-100 kg) Exhibiting Different Protein Accretion Rates
Variable |
Control |
pST |
||||
Protein deposition, g/day |
120 |
120 |
145 |
170 |
190 |
215 |
Change, % |
0 |
0 |
20 |
40 |
60 |
80 |
Maintenance protein, g/day |
13 |
13 |
13 |
13 |
13 |
13 |
Tissue protein requirement, g/day |
133 |
133 |
158 |
183 |
203 |
228 |
Dietary protein digestibilitya |
0.86 |
0.86 |
0.86 |
0.86 |
0.86 |
0.86 |
Biological valueb |
0.62 |
0.45 |
0.62 |
0.62 |
0.62 |
0.62 |
Net protein utilization for growth and maintenance |
0.53 |
0.39 |
0.53 |
0.53 |
0.53 |
0.53 |
Dietary "ideal" protein, g/dayc |
248 |
341 |
298 |
345 |
383 |
430 |
Dietary lysine, g/dayd |
16.6 |
22.8 |
22.0 |
25.5 |
28.3 |
31.8 |
NOTE: Data are based on results shown in Figure 5-6. a Dietary protein digestibility is based on estimates provided in Nutrient Requirements of Swine, Ninth Edition (National Research Council, 1988c). b Biological value: 0.62 is the efficiency of absorbed protein use for protein deposition and is based on determined values from Figure 5-6 for digestible ideal protein. This value is consistent with Krick et al. (1990) for pST treatment on absorbed lysine utilization. The 0.45 coefficient is closer to the estimates derived by Krick et al. (1990; see Boyd et al., 1991), Wiesemuller (1987), and as computed from Moughan (1991) in untreated pigs fed conventional diets. c This is the minimum level of an "ideal" protein having a perfect amino acid pattern. d Based on lysine composition in protein. Refer to text. |
depends on the energy density of gain allowable by the dose of a particular metabolic modifier. For example, at a dose that maximizes protein accretion, pST reduced caloric gain in growing swine from 3.30 to 1.75 Mcal/day during the 50 to 100 kg phase (see Figure 2-2). Equally striking are the dynamics of pST dose in relation to both intake and protein accretion (Figure 2-2). Thus, voluntary intake must be documented in relation to dose of the particular metabolic modifier when administered under ad libitum feeding conditions; subtle differences exist for pigs on energy-restricted regimens.
Also, the energy intake versus pST dose relationship may vary with phase of growth and, to a lesser extent, by genotype and gender. For example, pST reduces feed intake of ad libitum fed pigs less (-8 percent) when administered during the early growth phase (20 to 50 kg body weight) than when administered during the finishing phase (-23 percent; Table 5-1). The biological basis for this is not clear but appears to be a reflection of the relative changes in protein and fat accretion induced by pST. The impact on intake may also differ with metabolic modifier. Table 5-3 shows that the β-agonist ractopamine causes intake to decrease only slightly in comparison to pST.
Digestion
The tissue requirement for amino acid deposition is a function of both the need for deposition in tissues and for maintenance. The dietary requirement, however, is a function of the extent to which dietary protein is digested and amino acids absorbed as well as the efficiency with which absorbed amino acids are used for protein deposition. In principle, the effects of ST on energy and protein utilization in farm animals appear to be principally associated with the use of absorbed nutrients (Boyd and Bauman, 1989). It is conceivable, however, that digestion is altered indirectly. For example, a reduction in feed intake could result in a slower rate of passage, which in turn may lead to increased digestibility. This probably accounts for the small improvements in nitrogen and energy digestion observed with pST treatment (Wray-Cahen at al., 1991). The relative advantage observed for digestibility of nitrogen in pigs administered pST compared to control counterparts (87 versus 84 percent for controls), with each group fed ad libitum, was predictable from the reduction in intake (Haydon et al., 1983; see also Verstegen et al., 1990). Thus, little or no difference is expected for pigs already fed via a restricted intake regimen.
Maintenance
pST has been shown to alter the maintenance requirement for both energy and amino acids. The higher maintenance energy requirement for pST-treated pigs (Campbell et al., 1988; Verstegen et al., 1989) appears to be an inevitable consequence of increased protein mass (Dickerson, 1985; Campbell and Taverner, 1988). Therefore, when the maintenance cost is constant per unit of lean tissue, the pST-treated pig will have a greater maintenance requirement because it has a greater proportion of lean tissue at any given body weight. However, an increase of 10 percent of the
increased maintenance energy observed in the pST-treated pig could not be accounted for by increased protein accretion (Verstegen et al., 1989; Noblet and Dubois, 1990; Noblet et al., 1992). This difference appears to be related to changes in metabolic activity of organs. For example, Noblet observed that oxygen consumption of some organs was increased (e.g., 50 percent for liver; J. Noblet, Centre de Recherches de Rennes, INRA, St. Gilles, L'Hermitage, France, personal communication, 1990), which is significant because organ weights have been shown to be increased by 25 to 40 percent (Evock et al., 1988). The relevance of these changes to nutritional requirements of restrictively fed pigs is that an adjustment in intake would be required; however, they are of little practical importance to the formulation of diets fed ad libitum.
Efficiency of Absorbed Nutrient Use
As discussed, the effects of ST on energy and protein utilization appear to be postabsorptive. Consequently, the question of whether dietary protein requirements increase in direct proportion to the level deposited depends on whether a metabolic modifier alters the efficiency with which absorbed nutrients are deposited. Furthermore, this facet appears to be an area of opportunity in that a significant inefficiency appears to exist in amino acid metabolism. For example, the efficiency of absorbed amino acid use for protein deposition is known to be inherently low and variable in conventional diets (e.g., partial efficiency of 0.40 to 0.60; Rerat, 1972; Wiesemuller, 1987; Moughan, 1991). Values on the order of 0.80 to 0.94 have been achieved in growing pigs given semipurified diets and fed to optimum rates of growth (Moughan and Smith, 1984; Wang and Fuller, 1989; Chung and Baker, 1992). Although there is no satisfactory explanation for this inefficiency, involvement of amino acids as substrates for processes other than protein deposition accounts for only a small proportion of this inefficiency. To what extent amino acids may be diverted from oxidation in general is unknown, but the impact on dietary requirements is enormous. There is evidence, however, that ST improves the efficiency of amino acid partitioning to protein by as much as 20 to 40 percent in young growing swine (Campbell et al., 1990b; Boyd et al., 1991), cattle (Houseknecht et al., 1992), and lambs (Beermann et al., 1991). A change of this magnitude would have a pronounced effect on the dietary requirement for amino acids.
Theoretical considerations appropriate for the efficiency of absorbed amino acid use are illustrated in Figure 5-4. If the partial or metabolic efficiency of amino acid use for protein deposition is not altered by a metabolic modifier, then the requirement for a particular amino acid would vary in direct proportion to the protein accretion rate (Figure 5-4A). Alternatively, if a metabolic modifier increased the efficiency of amino acid use, then increased protein accretion would be accommodated with proportionally less amino acid input than the conventional animal (Figure 5-4B). In fact, an improvement in the partial efficiency of 20 to 40 percent could result in little or no change in the amino acid requirement for metabolically modified animals despite marked changes in the rate of protein accretion.
ESTIMATES OF THE LYSINE AND ENERGY REQUIREMENTS FOR ST-TREATED GROWING SWINE
Amino Acids
Several definitive studies have been conducted to determine the impact of pST on the dietary requirement for protein (amino acids) during the early and later phases of growth (20 to 60 and >60 kg). In general, the effects of pST on protein accretion cannot be fully exploited without an increase in protein (amino acid) intake, but a striking difference

FIGURE 5-4
Theoretical protein (amino acid) dose-response curves for metabolic modifiers. (A) A scenario wherein the efficiency of amino acid deposition is not altered; (B) a scenario wherein efficiency of amino acid deposition is improved. Scenario A would require an increase in protein (amino acid) intake to effect an increase in protein deposition beyond that achieved by a pig not supplemented with a metabolic modifier (solid line). Scenario B implies a reduction in protein (amino acid) intake required (dashed line) to achieve a comparable response to a pig not supplemented with a metabolic modifier.
exists in the extent to which the requirement must be increased for the two phases of growth. As previously mentioned, these studies also suggest that pST increases the partial efficiency of amino acid utilization for protein accretion. Particular emphasis will be placed on studies in which protein (or amino acids) dose-response curves were established for both control and pST-treated pigs because such data simultaneously demonstrate the impact of pST on the requirement for protein and the efficiency with which dietary protein is used for protein accretion.
GROWING PHASE (20 TO 60 kg)
Three studies have been conducted to establish the relationship between protein intake and protein accretion in pigs administered pST during the 20 to 60 kg phase of growth. In each study, protein accretion was determined by the comparative slaughter technique in control and pST-treated pigs and regressed on dietary protein intake. These data enable determination of the tissue requirement for and efficiency of amino acid deposition as protein so that improvements in protein deposition can be quantitatively related to required dietary inputs.
Campbell et al. (1990b) used intact male pigs injected daily with either a buffer control or pST (90 µg/kg body weight/day) between 30 and 60 kg body weight. Increments of protein (8 to 23 percent), calculated to provide an ideal pattern of amino acids (Agricultural Research Council, 1981; Taverner, 1987) were added to a diet that was restrictively fed at 88 percent of ad libitum energy intake. The level of dietary protein that accommodated maximum response resulted in a net deposition of 174 g protein/day for pST-treated boars (25 percent higher than controls) as compared with 139 g/day for controls. The most impressive observation was that the rate of protein accretion per unit of dietary protein intake was increased with pST such that the maximal response to pST required only a slight increase in total dietary protein intake (2.4 percent; 17.0 versus 17.4 percent dietary crude protein) to fully exploit the effects of pST on protein accretion and, thus, efficiency of live-weight gain. This equates to approximately 22.0 and 22.6 g lysine/day for control and pST-treated pigs, respectively. In principle, the dietary requirement of lysine may be estimated because amino acids were supplied in an approximately "ideal" pattern. Thus, when protein accretion was regressed on protein intake, pST increased the efficiency of protein use for protein accretion by approximately 25 percent. The absolute estimates for both dietary protein and lysine are anticipated to be slightly overestimated since it has been shown that the Agricultural Research Council's pattern of amino acids is low in sulfur amino acids, threonine, and tryptophan (Wang and Fuller, 1989; Chung and Baker, 1992). This would not affect the conclusion regarding relative differences in the requirement between treatments, however.
Similar observations (Figure 5-5) were also reported by Krick et al. (1993) in a study in which castrate and female pigs were administered either control buffer or pST (150 µg/kg body weight/day) between 20 and 55 kg body weight. Increments of protein (6.4 to 23.5 percent), approximating the "ideal" pattern of amino acids established by Wang and Fuller (1989), were added to a diet and fed ad libitum. Dose-response curves for protein accretion and efficiency of gain suggest a minimum dietary lysine requirement of approximately 24 g/day (148 g/day protein accretion) for the pST group compared to 23 g/day (119 g/day protein accretion) for controls. These data likewise suggest that pST increases the efficiency of amino acid use for protein accretion because a marked increase in protein deposition occurred (+24 percent) with little change in the estimated requirement for dietary lysine (2.6 percent). The apparent increase in the efficiency of amino acid use is further supported by the data of Caperna et al. (1991).
Thus, Figure 5-4B is a portrayal of the appropriate scenario for the effects of pST on amino acid use during this growing phase. Based on the data presented by Campbell et al. (1990b) and Krick et al. (1993), the biological value of absorbed amino acids appears to increase by approximately 25 percent with pST treatment. The net effect is that the dietary protein (amino acids) requirement for pigs treated with pST during this phase of growth would be equivalent to that recommended for untreated pigs if pST caused a 20
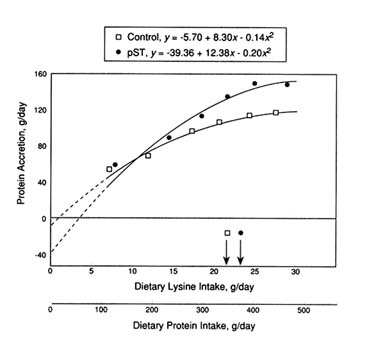
FIGURE 5-5
Dose-response curve for control and pST-treated castrate male and female pigs weighing 20 to 60 kg and receiving increments of dietary protein having an approximately ideal amino acid balance (Krick et al., 1993). The pST selected elicited a maximum response in protein accretion.
percent increase in protein accretion. These results have important practical implications and provide new insight into amino acid use, which warrants further research.
FINISHING PHASE (60 TO 100 kg)
The relationship between protein intake and response to pST during the finishing phase has been examined, but results are less clear than for younger pigs. Clearly the benefits of pST cannot be realized without an increase in dietary protein (amino acids). However, it has not yet been conclusively established whether the protein requirement per unit of protein accretion is unchanged with pST treatment or if the partial efficiency is improved as observed for growing pigs (<60 kg). Three of the studies were designed to quantitatively address this question but results were not in total agreement.
In the study by Campbell et al. (1991), intact male pigs between 60 and 90 kg were injected daily with either a control buffer or pST (90 µg/kg body weight) and were fed diets ranging from 7.3 to 23.8 percent crude protein of an "ideal" amino acid pattern (0.46 to 1.56 percent lysine) (Figure 5-6). Energy intake was restricted (average, 8.53 Mcal DE/day). Whole-body protein deposition increased from 119 to 215 g/day (88 percent) as a result of pST administration. The daily protein intake required to support maximal protein deposition in control and pST-treated pigs was 246 and 357 g/day, respectively. Corresponding lysine intakes were 18.8 g/day (equal to 0.68 percent on an as-fed basis) and 27.1 g/day (equal to 1.20 percent) for control and pST-treated pigs, respectively. For control pigs, the estimate for lysine (18.8 g/day) is slightly lower than anticipated, given the response of the same genotype during the 30 to 60 kg phase of growth, but this may reflect an energy constraint imposed on protein accretion. These data suggest that the plateau for maximum protein accretion was increased with pST treatment but that the biological value was unchanged (0.62). Based on these results, the dietary protein (amino acids) requirement for pST-treated pigs during the finishing phase of growth would increase in direct proportion to the increase in protein accretion rate (Figure 5-6).
Different results were obtained in the study by Krick and co-workers (1990; see also review by Boyd et al., 1991). Protein-response curves were likewise established for both control and pST-treated pigs (150 µg/kg body weight) with a rapidly growing strain of castrate males and females during the finishing phase. Dietary lysine concentrations ranged from 0.35 to 1.56 percent (9.9 to 22.5 percent crude protein) with lysine computed to be first limiting amino acid (Wang and Fuller, 1987; National Research Council, 1988c). Energy intake was not restricted. Whole-body protein deposition increased from 136 g/day for controls to 236 g/day (74 percent) for pST-treated pigs. The daily requirement for lysine was estimated to be 32 and 35 g/day for control and
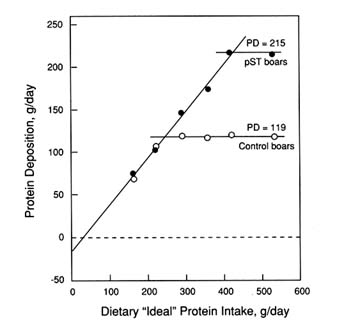
FIGURE 5-6
Dose-response curve for control and pST-treated intact male pigs (treated from 60 to 90 kg) and receiving increments of a dietary protein having an approximately ideal amino acid balance (Campbell et al., 1991). The pST dose used was 90 µg/kg body weight/day. Carcass protein deposition (PD) rate was determined by comparative slaughter.
pST groups, respectively (a 17 percent increase). The partial efficiency of lysine use was increased from a low 0.40 in control pigs to 0.60 in pigs receiving pST. This improvement in the partial efficiency of lysine use is in agreement with their previous study with pigs weighing 20 to 55 kg. A similar improvement has also been reported recently in pigs administered pST during this phase of growth (+30 percent; Noblet et al., 1992) and in growing cattle (Houseknecht et al., 1992) and lambs (Beermann et al., 1991). The apparently high estimate for required dietary lysine in control pigs is due to high levels of protein deposition and the relatively low efficiency of absorbed lysine utilization. The latter may have been partially the result of a less than ideal lysine: protein ratio, which varied from 3.5 percent to 5.0 percent over the linear response range (Krick, 1993).
The studies of Campbell et al. (1991) and Krick et al. (1990) with finishing pigs are in agreement on two critical facets: (1) that the effects of pST cannot be fully appreciated without an increase in lysine and other dietary amino acids and (2) that the efficiency of absorbed lysine use when conventional diets are fed to pST-treated pigs is approximately 0.60. Consequently, the requirements for dietary lysine and ideal protein could be estimated in relation to anticipated improvements in protein deposition. Use of pST at or near the dose optimum for protein deposition (according to the above studies) allows for estimation over a
wide range of potential pST doses. The two studies cited differ, however, with respect to the ability of pST to improve the partial efficiency of lysine utilization by finishing pigs. It is possible that both experiments are correct and that the ability of pST to improve the efficiency of absorbed lysine utilization depends on the partial efficiency achieved through nutritional means. For example, it is possible that pST could not metabolically increase the partial efficiency achieved by Campbell and co-workers (0.62 for absorbed protein use) because it may approximate a "physiological" maximum for the dietary conditions. Alternatively, the low efficiency of lysine use (0.40) observed by Krick and co-workers (1990; see also Boyd et al., 1991) may have allowed for an ST-mediated improvement. This dilemma impacts solely on our ability to predict requirements for nontreated control pigs, since the partial efficiency value is more uncertain than previously believed (0.65; Whittemore, 1986; Boyd et al., 1991). It is not clear whether the relatively higher efficiency of lysine (and protein) use by nontreated pigs in Campbell and co-workers' study as compared to Krick and co-workers and even Wiesemuller (1987; 0.45 to 0.50) is the consequence of differences in amino acid balance or differing endocrine status conferred by the intact male.
Results of less quantitative experiments strengthen the conclusion that the response to pST would be constrained without additional protein intake during the finishing phase (Newcomb et al., 1988; Goodband et al., 1990). Each conducted experiments with female and castrate male pigs in which successive increments of either dietary protein or lysine (diets exceeded anticipated requirement for other amino acids) were added to diets of pST-treated pigs. However, neither group established a dose-response curve for control pigs and assumed the NRC (1988c) minimum recommendation as appropriate for their respective genotypes. Another important difference from the studies discussed previously is that the pST dose used (4 and 3 mg/day, respectively) was below that observed to maximize protein accretion, since the intent was to determine the dietary lysine and/or protein requirement for pigs treated with pST at levels anticipated for commercial use. This is obviously important when making comparisons between different data sets. Goodband and co-workers (1990) suggested that the requirement for dietary lysine to support maximal growth (for a 4 mg/day pST dose) was doubled (1.20 percent dietary lysine or 30 g lysine/day) for pST-treated pigs. This represents a 58 percent increase in the daily requirement but a 35 percent increase for the growth period assumed to be 60 to 90 kg because fewer days were required to reach target weight. Newcomb and co-workers (1988) concluded that the rate and efficiency of gain in pST-treated pigs was maximized at approximately 20 percent protein (or 1.10 percent dietary lysine equivalent).
Comparison between the latter estimates and that of Campbell et al. (1991) and Krick et al. (1990) is not possible because protein accretion data are required to compare estimates at equivalent rates of protein deposition. However, an approximation of the dietary lysine requirement for commercially anticipated levels of pST can be derived from the data presented in Figure 2-2. A dose of 4 mg pST/day would elicit approximately 60 percent of the potential improvement in protein accretion (i.e., 44 versus 74 percent observed at dose maxima; estimated from the pST dose titration curve by Krick et al., 1992). Relating this level of response to the protein accretion portion of Figure 2-2 results in an estimated protein accretion of 196 g/day. This in turn equates to approximately 30 g dietary lysine/day or 1.12 percent dietary lysine based on an actual ad libitum intake of 2.67 kg/day (B. Krick, Department of Animal Science, Cornell University, personal communication, 1990).
Dietary Energy
The question of whether the response to a metabolic modifier depends on a proportional increase in protein input must include consideration of the relationship between energy intake and protein deposition. Knowledge of this relationship is important to determine (1) whether further improvements in protein accretion are constrained by energy intake and (2) whether, and to what extent, energy restriction beyond that induced by the metabolic modifier is appropriate in attempting to match energy intake with lean tissue yield.
GROWING PHASE (20 TO 60 kg)
The relationship between energy intake and protein accretion in growing castrate male pigs administered with pST is summarized in Figures 5-7 and 5-8 (cited from Campbell et al., 1988). Protein deposition increased in a linear manner with each increment of energy intake for both control and pST-treated pigs (Figure 5-7). Porcine ST affected both an increase in line slope and position. Thus, pST is efficacious over a wide range of energy intakes below ad libitum consumption and partitions energy toward protein deposition. Furthermore, there was no evidence of having achieved the plateau for protein deposition for either control or pST-treated pigs, which suggests that energy intake was a common constraint during this phase of growth (25 to 55 kg). The ability of pST to partition energy away from lipid deposition in adipose tissue at each level of energy input may have accounted for the ability to stimulate protein accretion at each level of energy intake (Figure 5-8).
FINISHING PHASE (60 TO 100 kg)
A summary of energy intake-protein deposition response curves for control and pST-treated intact male and female pigs during the finishing phase appear in Figures 5-3 and 5-8. For control groups, protein deposition increased with each
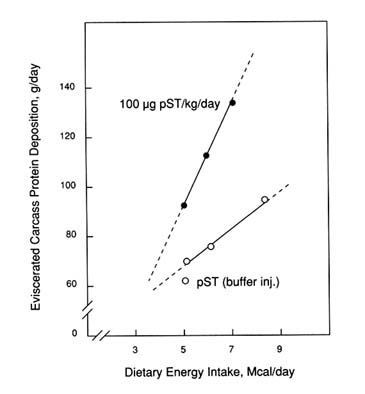
FIGURE 5-7
Carcass protein deposition response of castrate male pigs treated from 25 to 55 kg and treated with pST (100 µg pST/kg body weight/day) or buffer when fed three levels of dietary energy (Campbell et al., 1988). Protein deposition rate was determined in the eviscerated carcass.
increment of energy intake and achieved a plateau. This is consistent with other studies on pigs during the finishing phase, which demonstrates that protein deposition is not constrained by energy intake regardless of gender or genotype (Whittemore, 1986; Campbell, 1987; Dunkin, 1987).
With respect to Figure 5-3, maximum protein deposition was greater for male pigs (180 g/day) versus female pigs (112 g/day) and was achieved at approximately 80 percent of ad libitum energy intake (9.56 Mcal/day), whereas maximal protein deposition for gilts was achieved at 62 percent ad libitum energy intake (6.8 Mcal/day; Campbell et al., 1991). Thus, different restrictive feeding regimens would be required to accommodate maximal lean tissue deposition in male, compared to female pigs.
Using information obtained by the comparative slaughter technique, energy retention plots can be constructed to estimate maintenance energy expenditure. The energy retention plots for control and pST-treated female pigs are shown in Figure 5-8. Data are shown for female pigs because the magnitude of the pST response is greater (45 percent increase) than observed for male pigs. Similar calculations for male pigs indicated that pST administration increased maintenance energy expenditure by 30 percent. At energy intakes up to 7 to 9 Mcal DE/day, pST eliminated the effect of gender on protein accretion. This finding is consistent with the results of Campbell et al. (1989a). In the latter study, females were reported to be more responsive to pST with respect to stimulation of growth performance and protein accretion. A biological explanation for this is unclear other than that the intact male elicits near maximal protein accretion and growth in general is more closely synchronized with genetic capacity. Female pigs treated with pST exhibited a plateau for protein deposition at 7 to 9 Mcal DE/day, or 86 percent of ad libitum energy intake, even though protein accretion was 80 percent greater than control females (202 versus 112 g/day). Protein accretion of intact males responded linearly to increased dietary energy intake up to ad libitum (9.56 Mcal DE/day). Consequently, intact males exhibited slightly greater protein accretion capacity (240 versus 210 g/day) beyond that exhibited by females consuming 7 to 9 Mcal DE/day.
It is noteworthy that pigs administered pST and weighing approximately 75 kg exhibit a similar efficiency of growth (1.95 and 1.98 for males and females, respectively) as generally observed for pigs weighing 15 to 20 kg. The regression coefficient for the linear component of the protein deposition-energy intake relationship of pST-treated males and females was also similar to that reported for younger pigs by Campbell and Taverner (1988). Accordingly, the dietary amino acid requirements of pST-treated pigs in the finishing phase might logically be expected to approximate those of pigs weighing 15 to 20 kg. The NRC (1988c) recommends 0.95 percent lysine (2.78 g/Mcal DE) and the Agricultural Research Council (1981) recommends 1.25 percent lysine
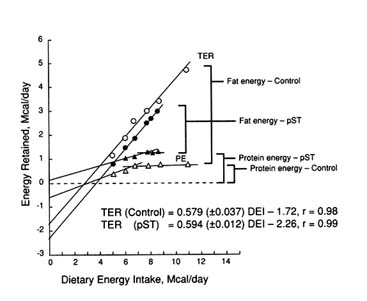
FIGURE 5-8
Effect of digestible energy intake (DEI) on total energy retained (TER) as protein energy (PE) and maintenance energy required (at zero DEI) for control and pST-treated pigs. Dose of pST administered was 100 mg pST/kg body weight/day.
(3.6 g/Mcal DE) for pigs weighing 10 to 20 kg. Levels within this range would appear adequate to support near maximal protein accretion (70 to 75 percent) and growth performance of pST-treated females and castrate males.
Dynamic Estimates of the Lysine Requirement for ST-Treated Swine
Quantitative information as presented in Figures 5-5 and 5-6 may be used to compute the tissue and dietary protein and lysine requirements for various levels of protein accretion. To develop a more dynamic approach we need to know
-
the digestibility of dietary protein (amino acids),
-
how the metabolic modifier alters the rate of protein accretion (plateau of Figures 5-5 and 5-6) and the requirement for maintenance (intercept of Figures 5-5 and 5-6),
-
the pattern of amino acid deposition (e.g., lysine), and
-
the partial efficiency with which absorbed amino acids (protein) are used for deposition and maintenance (slope of line of Figures 5-5 and 5-6).
To derive dynamic estimates for the dietary lysine requirement (DLR, expressed as g/day) the information is interrelated as follows:
DLR= (RLD + OLL) / (BV x DIG),
where, RLD is rate of lysine deposition (protein deposition in g/day × percent tissue lysine), OLL is obligatory lysine loss (tissue requirement for maintenance lysine), BV is biological value or the partial efficiency of dietary lysine use for protein accretion, and DIG is digestibility of dietary lysine. The need for a more complete description of experimental conditions with information appropriate for a more dynamic approach is apparent and should be incorporated into the design of future experiments. Ultimately, estimates for nutrient requirements must be related to the expected energy intake to ensure that the requirements for amino acids are met.
A summary of the impact of different protein accretion rates and biological values for amino acid use is portrayed in Table 5-2. This serves as an example of the use of factorial estimation and illustrates how appropriate data from growth studies may be used to address dynamic situations. The impact of metabolic regulation on the dynamics of the "ideal" protein and lysine requirement was calculated using 120 g/day as the baseline rate of protein accretion (Figure 5-6). Whole-body protein accretion rates of 100 and 135 g/day are typical of reported values for castrates and females (Wiesemuller, 1987; Boyd and Bauman, 1989) (Figure 5-5). However, contemporary strains are known to deposit less protein (85 g/day; Wiesemuller, 1987). Proportional increases in protein deposition to 180 and 210 g/day are likely with pST treatment of pigs
TABLE 5-3 Expected Field Responses to Porcine Somatotropin (pST) and the β-Agonist Ractopamine
Criteria |
Somatotropina |
Ractopamineb |
Growth rate |
+12 |
+9 |
Feed: gain |
-25 |
-12 |
Diet intake |
-16 |
-4 |
Back fat (tenth rib) |
-35 |
-14b |
Loin area |
+28 |
+15b |
Dissected tissue mass |
|
|
Skeletal muscle |
+25 |
+12b |
Adipose |
-30 |
-14b |
NOTE: Values are relative response, percent of control. a Computed from Krick et al. (1992). The pST dose used was 50 µg/kg body weight/day. Tissue mass data were derived from Thiel et al. (1993) using the same pST dose in the same experiment. b Adapted from Veenhuizen and Anderson (1990). Growth performance data are summarized for 12 trials; dissection data represent 24 animals and were expressed as percent of carcass. |
that have a 120 to 135 g/day base. One must bear in mind that a 40 to 60 percent stimulation of protein deposition may occur with a commercial dose of pST, but the actual level achieved will be a function of dose and base accretion rate for the genotype. These in turn will ultimately determine the dietary lysine requirement. The alternative biological value of 45 percent for untreated control pigs in Table 5-2 reflects the results of Krick et al. (1990; see also Krick, 1993) and Wiesemuller (1987) in contrast to Campbell et al. (1991); hence, the impact on the dietary lysine requirement is the same based on Campbell et al. and Krick et al., but the relative increase predicted in relation to untreated pigs differs.
The proportion of lysine in pig tissue was 6.7 percent based on analysis of protein for 20 and 60 kg pigs (Krick et al., 1993). This agrees relatively well with previous reports (Campbell et al., 1988; Batterham et al., 1990; Chung and Baker, 1992) but is lower than observed for pST-treated counterparts (7.4 percent; Krick et al., 1993). This apparent increase in lysine content coincided with a reciprocal decrease in glycine. The pattern of amino acids in pST-treated pigs appeared to be otherwise similar. The estimate for maintenance or obligatory protein (or lysine; Figure 5-5) loss was derived at zero lysine (or protein) intake. Dietary lysine is anticipated to remain the first limiting amino acid for ingredients normally used, but preliminary estimates for other selected essential amino acids could not be derived using a validated "ideal" amino acid pattern for untreated pigs (Wang and Fuller, 1989; Chung and Baker, 1992), since the pattern for pST-treated pigs appears to be altered.
The dynamics of gender, genotype, type and dose of metabolic modifier, and feeding strategy make it unrealistic to suggest a single dietary requirement for amino acids for all growing pigs. Consequently, conventional nutrient requirement
tables can only be used as a guide. Only computer-simulated models can integrate requisite animal, environmental, and dietary factors to arrive at biological and economic optimums for dietary nutrients. A number of such models have been developed for growing pigs (Black et al., 1986; Whittemore, 1986; Moughan, 1991).
It is also important to appreciate that nutrient recommendations need to be amenable to "whole-model" production economics. Ultimately, the most appropriate requirement in practice is that which accommodates an economic optimum. It is arrived at by relating predicted responses from nutrient input and associated costs to predicted return. This approach is based on factorial methodology and best accommodates the needs of animal producers.
ESTIMATES OF AMINO ACID AND ENERGY REQUIREMENTS FOR SWINE ADMINISTERED β-ADRENERGIC AGONISTS
β-adrenergic agonists (β-agonists) cause reciprocal shifts in protein and lipid accretion, but more quantitative information is needed to determine whether nutritional requirements are changed. Several important differences exist that are examples of factors that must be considered in evaluating the impact of new technologies on nutrient requirements. First, while it is convenient to discuss β-agonists in general terms, one must appreciate that each differs structurally. As a result of differences in mode of action, effects on promoting lean deposition and ancillary actions can be expected (see Chapter 2). Second, at least one compound has been shown to elicit a transient response in gain and efficiency of gain. This has not been adequately documented in studies with other β-agonists, yet it is a fundamental consideration. Third, β-agonists appear to cause a differential growth in that increases in protein accretion are only observed in specific carcass components. This is in contrast to ST, which causes a generalized growth of proteinaceous tissues. This would have a bearing on the pattern of amino acids needed for tissue growth.
Unlike ST, β-agonists are orally active and effective at relatively low concentrations (0.5 to 20 mg/kg diet). Their general effect is to increase the rate of skeletal muscle growth, concurrent with a reduction in lipid accretion. Rate and efficiency of growth are generally improved, but differences have been variable and possibly related to differences in effectiveness between compounds, phase of growth, and nutrient provision. Results from 12 studies involving ractopamine hydrochloride fed to castrate male and female pigs have been summarized by Veenhuizen and Anderson (1990) and appear in Table 5-3.
Proper characterization of the response to metabolic modifiers also involves definition of the temporal pattern of growth (i.e., pattern of nutrient deposition). The importance of this is illustrated in Figure 5-9 with the β-agonist L-644,969. The data show a declining response in growing swine to this particular β-agonist throughout the treatment period (Wallace et al., 1987). A similar response pattern has been observed for growing cattle (Moloney et al., 1990). The difficulty that this poses when either attempting to define required nutrient input or when measuring metabolic or hormone patterns is that the tissue requirement is also in a dynamic flux.
The relative importance of dietary lysine is expected to increase in relation to other indispensable amino acids because intestinal growth is not adversely affected by treatment or by the rerouting of nutrients from adipose to muscle tissue coincident with enhanced muscle growth with β-agonist administration (Reeds and Mersmann, 1991). This appears to be a common feature for this class of metabolic modifiers and results in a greater proportion of lysine deposition relative to other amino acids. Thus, lysine would appear to be first limiting and, as such, should be the focus of initial efforts in the evaluation of amino acid requirements. The differential pattern of tissue(s) growth may also confer an advantage in amino acid use relative to ST in that the greater relative allocation of amino acids to muscle confers an efficiency that may affect the amino acid requirement. However, such differential effects on visceral and carcass components may imply a specificity for β-agonist effects during the finishing phase of growth when the relative rate of visceral tissue accretion is diminished. This may partially account for the positive response of growth in pigs fed marginal protein diets (12 percent protein; Mitchell et al., 1991).
Although the efficacy and relative responsiveness to the β-agonist ractopamine has been established for growing swine (Table 5-3), quantitative information is generally lacking
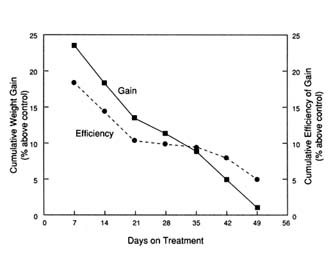
FIGURE 5-9
Temporal pattern of the growth response of pigs administered the b-adrenergic agonist L-644,969 for 7 weeks (Wallace et al., 1987).
for the various components required for factorial analysis, and, concomitantly, little information is available on how nutritional requirements will be altered. Several reports indicate that increases in dietary protein intake are required for the effects of ractopamine to be fully exhibited with respect to composition of gain and growth performance (Anderson et al., 1987; Adeola et al., 1990; Bracher-Jakob and Blum, 1990; Dunshea et al., 1991; Mitchell et al., 1991). To our knowledge, only two studies provide quantitative information on rates of protein deposition in relation to lipid deposition and protein and energy intake (Dunshea et al., 1991; Mitchell et al., 1991).
Mitchell et al. (1991) reported that the ractopamine effect was influenced by dietary protein level and constrained by dietary energy intake if administered during the finishing phase (>60 kg) of growth. However, maintenance energy expenditure was not influenced by the β-agonist. Energy restriction, in a manner and level analogous to previous studies with pST (Figure 5-3), precluded a response in body protein deposition to increments of dietary protein (Figure 5-10). This suggests an apparent inability of ractopamine to depress lipid deposition rate significantly, thereby resulting in an energy constraint of the protein deposition response. This is in contrast to observations with pST.
Dunshea et al. (1991) conducted a systematic protein titration study to determine whether the ractopamine response was caused by increased intake of dietary protein and/or increased biological value of dietary protein. The effects of this and other β-agonists appear to be associated predominantly with the use of absorbed nutrients (Reeds and Mersmann, 1991). Forty-eight female pigs were allotted to one of six levels of dietary protein (8.3 to 23.0 percent)
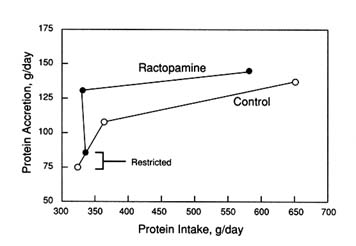
FIGURE 5-10
Protein deposition response of castrate pigs (treated from 60 to 90 kg) to diets containing 12 or 18 percent crude protein fed ad libitum or an 18 percent crude protein diet fed restrictively. Diets contained 0, 20 (12 and 18 percent ad libitum), or 30 (18 percent restricted) mg ractopamine/kg of feed (Mitchell et al., 1991).

FIGURE 5-11
Relationship between empty-body protein deposition and dietary protein intake in restrictively fed female pigs (7.1 Mcal DE/day) given diets with 0 and 20 mg ractopamine/kg of feed between 60 to 90 kg liveweight (Dunshea et al., 1991).
and two levels of ractopamine-HCl (0 and 20 mg/kg). They were restrictively fed to just below the energy requirement for maximum protein deposition (7.1 Mcal DE/day) from 60 kg until slaughter at 90 kg. Estimates for dietary protein requirements were approximately 14.0 and 16.8 percent respectively, to support carcass protein accretion rates of 91 and 112 g/day for 0 and 20 mg/kg ractopamine, respectively (Figure 5-11). Carcass protein deposition rates were not increased by ractopamine at or below 14 percent protein, and the regression slope of protein intake on protein deposition was similar for both treatments. Therefore, the efficiency of protein utilization was not altered as observed in studies with pST with an increase in protein deposition requiring a proportional increase in dietary protein (amino acids). This scenario was previously illustrated for pST (Table 5-2).
EFFECT OF METABOLIC MODIFIERS ON MINERAL AND VITAMIN REQUIREMENTS
The increase in the rate of bone ash deposition induced by exogenous pST (Table 2-2; Caperna et al., 1989; Bark, 1990), but not by the β-agonist ractopamine (Bark, 1990), and the structural abnormalities noted in ST-transgenic swine (Pursel et al., 1989) suggest the need for an increase in the daily intake of dietary calcium, phosphorus, and possibly other mineral elements by pigs treated with pST. The rate of bone deposition for pST-treated pigs appears to be a function of dose, with an approximate increase of 35 to 40 percent occurring with 3 to 4 mg pST/day (Bark, 1990). Calcium, zinc, and copper concentration of bone (mg/g dry bone or mg/g ash), however, are not influenced. Therefore,
deposition of specific bone structural elements appears approximately proportional to the increase in growth rate and the corresponding increase in bone mass associated with muscle mass. Assuming that the proportion of dietary minerals absorbed is not altered, a reasonable approach to satisfy this need would be to increase dietary calcium and available phosphorus at a constant rate in direct relation to the expected increase of protein accretion (i.e., 30 to 50 percent).
Because of the similarity in the pattern of nutrient deposition of metabolically modified pigs in the finishing phase and conventional nursery pigs (10 to 20 kg), dietary mineral and vitamin levels based on the estimated requirements of these younger animals may also be appropriate. The possibility for improved digestion, efficiency of use, and the extent to which increased deposition is actually required, versus promoted, are important questions to address. Clearly, this area needs additional research to systematically evaluate the effect of metabolic modifiers.
There is virtually no information relative to the impact of pST or ractopamine on vitamin requirements with the exception of one paper on vitamin D. Typical swine diet formulations contain added vitamin D and fortification levels for other vitamins that are 50 to 100 percent in excess of the minimum NRC (1988c) recommendations. Vitamin D metabolite concentrations differed in serum of pigs treated with exogenous pST (Goff et al., 1990). For pigs consuming a diet fortified with 880 IU vitamin D/kg, sufficient substrate was available to satisfy requirements, and differences observed in vitamin D metabolites reflect either rate-limiting enzymes in the vitamin D-1,25-dihydroxy-vitamin D pathway or represent a concerted adaptation proportionate to long-bone growth. The apparent absence of any other specific vitamin deficiency syndrome in research studies to date indicates there may be no need to further fortify diets with other vitamins beyond correcting for reduced intake.
SUMMARY
Recently developed technologies for modifying protein and energy metabolism provide animal scientists with an unparalleled opportunity to investigate the biochemical and metabolic controls of growth and development. At a practical level, these technologies have the potential to markedly improve the efficiency and competitive position for market share by swine producers. The dramatic effects of pST on protein accretion and thus efficiency of gain depend on markedly different nutrient-to-energy relationships than those that exist for untreated pigs fed ad libitum or restricted intake. A striking difference exists in the extent to which the requirement for amino acids must increase for different phases of growth. An increase in protein accretion of approximately 25 percent during the growing phase is achievable with only a small increase in the requirement for amino acids because of an improvement in the partial efficiency with which absorbed amino acids are used for protein deposition. At present, the effect of pST on protein (amino acid) requirements of finishing pigs is less clear. The marked increase in protein accretion (> 60 percent) cannot occur without at least a 20 percent increase in amino acid intake over nontreated controls. However, it remains to be determined whether the increased dietary requirement is directly proportional to the improvement in protein deposition induced by pST. Elucidation of this issue is important to the successful implementation of exogenous pST administration as a practical and economic means of enhancing the efficiency of meat production in pigs.
This review emphasizes the need for systematic research and gives precedence to studies designed to address the causes of low and variable efficiency of amino acid use. This is the single most important factor affecting estimates of the growing pig's requirements for dietary protein (amino acids) and the ability of a diet to meet tissue demands of pigs either treated or not with metabolic modifiers.