4
The Economic Challenge
The economic challenge that advanced reactor developers face is daunting. In the current market environment, advanced nuclear reactors will need to be cost-competitive with alternative low-carbon energy technologies. Developers will need to reduce the capital costs needed to deploy these technologies to enable their large-scale market entry and will also need to demonstrate cost and schedule control. As discussed in Chapter 3, nuclear energy is used almost exclusively to generate electricity as its end-product. Current commercial nuclear power plants (NPPs) are economically evaluated as a firm resource, comparable to coal-fired or natural gas-fired technologies. An expansion in the portfolio of market competitors, combined with pricing and regulatory reforms, have impacted the economic viability of nuclear power in some markets over the previous two decades, and will create increased barriers to entry for any advanced nuclear technologies. This chapter summarizes existing and emergent economic and financing challenges for nuclear technologies generally, and advanced nuclear reactors specifically.
Finding 4-1: The key economic challenge for advanced nuclear reactors is the need to either be cost competitive with other low-carbon energy systems in providing electricity, expand their use to applications beyond the electricity sector, or have an otherwise strong value proposition that encourages investment. Given anticipated market conditions, and the range of low carbon energy technology options, this will require reductions in capital cost.
ADDRESSING THE MARKET CHALLENGE: CAPITAL COSTS, COMPETITIVENESS, AND ENTRY BARRIERS
Despite the challenges of cost competition in electricity markets, which is discussed in Chapter 3, a 2018 study by the Electric Power Research Institute (EPRI) found that advanced nuclear could be economically competitive in some scenarios, identifying several key drivers that may influence future deployments: energy and environmental policies, cost of fuel for alternatives such as natural gas, regional factors such as gas pipelines and existing asset mixes, and capital costs for nuclear investments as evaluated in the context of the costs of other technology alternatives and market variations (EPRI 2018). A 2022 study by Pacific Northwest National Laboratory (PNNL) revalidated the importance of capital costs for nuclear and competing low-carbon technologies in determining the future trajectory of nuclear in the United States and addressed the potential impact of a carbon tax and net-zero emissions policies in leading to growth in nuclear deployment in the future grid (Kim 2022). Beyond market
forces, other studies have reflected on opportunities for improved cost control in development, innovation in labor management, and better project governance structures that may help in creating a learning environment where costs actually decline from first-of-a-kind (FOAK) to nth-of-a-kind (NOAK) builds, something not frequently seen in nuclear development (Ingersoll et al. 2020b).
The risks of investing in nuclear power are compounded by the often-significant uncertainties associated with nuclear development costs. The high capital cost and risk of cost growth could impact an investor’s willingness to pursue nuclear power when other options are available, even if a plant owner-operator sees utility in incorporating nuclear electricity generation. The uncertainty surrounding these economic risks impacts how investors view nuclear technologies and reducing these risks by lowering up-front costs and demonstrating disciplined cost control could be a key factor in overcoming concerns with a nuclear investment. While the EPRI and PNNL studies highlight the importance of capital costs, the entire cost landscape for nuclear development impacts its economic competitiveness and market viability. The PNNL study capital costs are based on the Idaho National Laboratory (INL) Nuclear Cost Basis Report (2022). The EPRI data and references are based on input from existing NPPs. The costs of advanced NPPs may be very different from the assumptions in these studies, based on potential differences in design, deployment, operation, and risk drivers. This variation in assumptions leads to significant uncertainty in future nuclear deployment pathways.
Power Plant Cost Drivers and Overcoming the “Sunk Cost” Challenge of Nuclear
Three major cost components in any power plant are capital cost, operating and maintenance costs, and fuel costs. Capital cost is composed of two parts: (1) the “overnight cost,” which refers to the cost of building the plant, including equipment, construction materials, site preparation and labor, excluding costs associated with financing and escalation (hence “overnight”); and (2) the cost of capital (interest on funds borrowed to build the plant, financed by either debt or equity). All upfront capital costs are “sunk” costs from the perspective of an investor because they are recoverable only over time from payments from energy production. The cost of capital is affected by the time required to construct the plant and the interest rate on the funds borrowed. In the United States, the cost of capital is dependent on technology, location, and developer; it is determined by how credible investors assess the project to be (i.e., is it a “safe bet” for generating return, and can it attract debt financing?), and a host of other factors including incentives by federal, state, and local authorities.
Once a plant is built, operation and maintenance (O&M) costs depend on the personnel needed and the materials used in operating the facility. The final cost component is the cost of the fuel required to generate energy. Capital costs, along with many components of operating costs, could be considered fixed costs that are incurred whether the plant is in operation or not. O&M costs have a variable component that is affected by personnel changes and materials used.1 Most fuel costs, by contrast, are variable because they are incurred only when the plant is operating.
Many low-carbon technologies, including nuclear power, are capital-intensive relative to their operating costs. Depending on nuclear plant design, capital cost can account for as much as 80 percent of the lifetime cost of energy from the plant, with the remainder of the cost typically divided between O&M (15 percent) and fuel costs (5 percent) (World Nuclear Association 2022). These percentages can vary for different plants depending on location (owing to differences in site preparation, labor, and permitting), actual plant construction time, and the interest rate on borrowed capital (which itself is a function of construction time). The cost structure for nuclear plants is in direct contrast to that of a natural gas plant, where 80 percent of the total lifetime cost is the fuel cost (EPRI 2018).
Because the dominant cost component for nuclear technology is the capital cost, it is instructive to provide a typical cost breakdown of that cost component. Following the classification approach used by Black and Veatch in 2012, a 2018 Massachusetts Institute of Technology (MIT) report found that for current light water reactor (LWR) plant construction projects (AP1000 in the United States, EPR in Europe, AP1400 in UAE), a small fraction of the overnight capital cost is owing to the nuclear island equipment (10–20 percent) and even less for the
___________________
1 Other O&M costs include water usage, discharge treatment, chemicals, and consumables (EIA 2020).
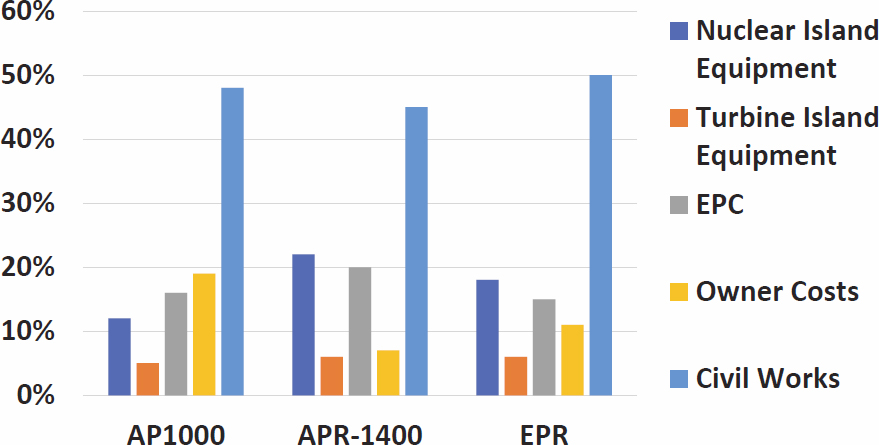
turbine-generator equipment (5–10 percent) (Buongiorno et al. 2018). As shown in Figure 4-1, most of the overnight cost was taken up by engineering, procurement, and construction (EPC) costs (10–20 percent), the cost of civil work (40–50 percent), and owner’s costs (10 percent).2 In its projections for market viability, the same MIT study independently estimated NOAK overnight capital costs for advanced reactor designs that were between $4,600 and $5,400 per kWe. It is noteworthy that the overnight cost estimates provided by the advanced reactor vendors to the committee were far lower (by ~50 percent). The capital cost mix may change if advanced reactors realize their goal of development using a manufacturing model,3 but it is still unclear if this would reduce the overall cost burden or simply shift the costs to development of a manufacturing support infrastructure. An in-depth discussion on EPC and civil works challenges is presented in Chapter 6, including a discussion of reasons for cost overruns and potential differences in cost outcome depending on the size and design of a reactor, and the manufacturing approach.
A more recent study of cost drivers for nuclear-specific development evaluated multiple nuclear projects to determine the dominant cost driver categories—that is, those that had the greatest influence on cost growth/overrun (Ingersoll et al. 2020b). A notable aspect of the analysis was a comparison of U.S. and European construction experience with that in the rest of the world (ROW). The evaluation highlighted the role of different construction practices, raw material costs, labor market costs, regulatory burdens, and financing structures.4 Highlighted here
___________________
2 Engineering procurement and construction costs are related to indirect engineering, quality assurance (QA), and supervisory costs. Cost of civil works includes costs to prepare the site, including excavations and foundations, the ultimate heat sink (e.g., cooling towers), other equipment, and the installation of plant components. Owner’s cost includes fees, permits, taxes, owner’s engineering costs, and costs for spare parts and commissioning.
3 The distribution of costs will also be specific to reactor design, and some traditional LWR cost categories may be inapplicable to new designs. See Ingersoll (2020b) for a discussion of potential cost differences. Additional cost analysis will be required as designs reach technical maturity.
4 ROW funding is often a significant factor in controlling overall costs given that financing costs during the build can escalate if there are schedule delays.
are several issues not tied to location, which should be addressed by any new nuclear developer to help control the costs of nuclear power in future developments. Specifically, successful programs have ensured that there is:
- A completed design prior to construction;
- A fully detailed development schedule that incorporates adequate quality assurance oversight;
- Adherence to a detailed build schedule;
- Sustained learning effects from repetitive builds of the same design;
- Preferential tax policies and cheaper financing.
The first four items relate to the management of development projects and are addressed along with other project management issues in Chapter 6. Notably, the passage of the Inflation Reduction Act (IRA) of 2022 recognizes nuclear as a low-carbon energy source eliminating uneven treatment of this technology compared to other low carbon technologies. Tax policy and finance mechanisms are addressed later in this chapter.
Finding 4-2: Nuclear developers face higher financing costs, and thus a greater sunk cost burden, than developers of other energy technologies. These higher costs result from (1) uncertain development and build times and (2) limited financing options and high interest rates that increase the finance burden, partly a result of a poor track record and consistent cost overruns in past construction.
UNIQUE ADVANCED REACTOR COST DRIVERS
Beyond the typical capital cost drivers, there are also unique cost drivers that advanced reactor developers will need to address, including a potentially more complex fuel cycle for some designs. Given the lack of reliable cost data on development of a fuel production facility, it is treated qualitatively rather than quantitatively in this report. The absence of a common fuel type among the competing advanced reactor designs means that multiple fuel supply chains will have to be developed for fuel fabrication at the required enrichment. Additionally, before a fuel supply vendor would choose to develop a facility, there must be sufficient demand (absent a continuing U.S. government commitment to develop and maintain a strategic capability and supply) to justify the development of new fabrication capabilities. This is further complicated by the extended fuel cycles (longer time between refueling) envisioned for several advanced reactors, which may result in intermittent demand absent an extended fleet development effort. If enough reactors of similar design are ordered over time, demand can be levelized despite the long fuel cycles for each reactor. However, it may be difficult to justify significant capital investment for supply chains that do not have regular demand. This may be particularly true for fuel production facilities that support multiple reactor types and that do not allow the standardization of processes or production cycles. Longer core lifetimes envisioned by the vendors can potentially offset a portion of the high cost of developing a new fuel form, but there is limited data to establish that higher fuel burnup will offset the added cost drivers. Additional analysis is needed to clearly demonstrate that the costs of developing a new fuel fabrication facility (or backfitting an existing facility) and the costs of higher assay fuels will be sufficiently mitigated by the longer core lifetimes postulated and other strategic planning efforts, such as staggered refueling schedules.
Multiple reactor designs also anticipate the use of high-assay low-enrichment uranium (HALEU). The supply chain for this more highly enriched fuel has additional costs associated with controls for special nuclear material of moderate strategic significance (NRC Category II). Until 2021, the absence of a reliable domestic HALEU supply chain meant that Russia was sometimes referred to as a potential supplier of necessity for HALEU. The 2022 Russian invasion of Ukraine has likely made this a non-viable pathway for the foreseeable future. Even when the war ends and if relations with Russia thaw, the energy security and geopolitical implications associated with this pathway will remain.
The implementation of a once-through fuel cycle would simplify cost assumptions for FOAK development and reduce regulatory risk, because costs for wet and dry storage are relatively well established. However, this eliminates one of the stated advantages of some advanced nuclear reactors—to reduce existing or future stockpiles of nuclear waste. If interim fuel storage is required for advanced reactor spent fuel, new storage casks for more
unique fuel forms and new facilities for processing novel fuel forms may be needed, both of which incur costs. However, a passively safe cask design would result in minimal operating costs and risk. Yet, successful widespread deployment of small reactors at a level that would contribute to greenhouse gas mitigation would require hundreds—potentially thousands—of reactors deployed at both current and new nuclear sites. Without developing centralized storage sites, a repository, or a fuel reprocessing capability, the waste management challenge might become even more urgent in a world dominated by small, advanced nuclear reactors. While there has been limited business or political interest in developing consolidated storage sites in the United States, two license applications have been submitted for interim storage facilities in Texas and New Mexico (NRC 2021a,b). These sites face political opposition, and waste disposal issues for advanced reactors face continued uncertainty. A recent National Academies’ report discusses this issue in great depth (NASEM 2022). Additional discussion on regulatory and societal challenges associated with storage facilities is available in Chapters 7 and 8.
The fuel cycle is not the only area where new designs may face supply chain challenges. For other critical specialized components (pumps, valves, heat pipes, etc.), design differences limit economies of scale that could reduce capital costs. As a result, government financial support may be required to ensure that supply chains are developed and maintained if a diverse advanced reactor ecosystem develops. Government support will likely be necessary at the early stage to help catalyze development of particular components, because private capital may be hesitant to invest before a vendor has clearly demonstrated a market for its design. There will, of course, be risk in this government support in that until there is growth in demand, there will be limited development of a supporting supply chain, and until there is a more robust supply chain with learning, costs will not come down to support cost reductions from FOAK to NOAK—a classic “chicken and egg” conundrum. Regardless, to demonstrate cost reductions, vendors will need stable supply chain support—which makes government funding the likely bridge to address a “supply chain valley of death.” Down-selecting to a more limited number of designs would reduce the supply-chain cost burden and focus government spending—this approach is discussed later in the chapter.
Finding 4-3: Commercialization of multiple designs would logically necessitate the development of a variety of supply chains. For example, there are multiple fuel types proposed for the competing advanced reactor designs. Extended operating cycles with fewer refueling outages may affect demand stability for nuclear fuel. Thus, fuel fabrication may exacerbate the cost challenges for nuclear power.
OTHER DRIVERS OF PERCEIVED UTILITY IN ENERGY MARKETS
Many other factors could influence the choice between generating options in a competitive market. The fundamental question is the “willingness to pay” for nuclear generation. In most electricity markets today, the primary comparative attribute used by energy service providers is levelized cost and levelized avoided cost when considering alternative technologies. However, as the energy transition unfolds and the supply, demand, regulatory, pricing, and technical pictures change, the various other attributes that advanced nuclear could provide may be valued sufficiently that a customer would be willing to pay a premium for this technology.
In some cases, an electrical generation company expanding its resource portfolio might determine that there is utility in having a balance of generation resources to ensure greater flexibility and reliability. This would differ substantially across locations and regulatory environments, rendering the estimation of a “new nuclear market” spatially dependent and uncertain (discussed further in Chapter 3). For example, where there is more limited renewable energy (RE) resource or greater restrictions on natural gas supply, there may be a more credible market opportunity for nuclear developers. While this scenario could tip the balance toward nuclear, the most likely outcome is decidedly unclear. But resource availability and cost are not the only factors that influence investment.
In addition to the pure economic factors, other decision criteria should be considered when selecting among energy generation technologies, including some that are not easily monetized. Within the field of behavioral economics, there has been significant consideration of the difference between a pure economic preference assessment, which is generally tied to evaluations and comparison of economic value, and a broader suite of considerations that affect choices, in which equity, safety, risk, public acceptability, and other societal impacts all play a role (EIA 2014).
OTHER ECONOMIC CONSIDERATIONS
Overall grid costs and cost avoidance are additional considerations in determining the viability of nuclear as a low-carbon resource. A 2022 study by Vibrant Clean Energy (sponsored by the Nuclear Energy Institute) indicated that constrained deployment of nuclear in decarbonization scenarios led to as much as $346 billion in additional development costs to reach comparable levels of grid decarbonization by 2050. While this study makes untested assumptions about nuclear NOAK costs, there is still a valid concern tied to overall system cost. If low-carbon sources such as nuclear are discounted before they are fully evaluated, higher overall electricity system costs are possible as much lower capacity factor solutions are deployed to meet growing electricity demand (Clack 2022). As noted in Chapter 3, an options approach that maintains flexibility in technology choice for as long as possible may be better in ensuring that this technology transition does not lead to costly dead ends.
To maintain flexibility, there may be value in the current approach by DOE to support smaller scale nuclear alternatives. Prior studies of nuclear construction costs have indicated that diseconomies of scale exist with nuclear power development and deployment based on the association between increases in plant size and increases in cost on a $/mWe basis (Talabi and Fischbeck 2013). Economic flexibility is often greater when the scale of the economic risk is lower for any individual technology of choice. Smaller scale yields opportunities for factory manufacture and assembly that could substantially mitigate many of the identified cost drivers in traditional construction, as well as enabling staged deployment and more controlled expansion, which may lower investment and deployment execution risk. Smaller scale may also better enable penetration into microgrid and alternative industrial process heat markets and provide a path to larger order books that may yield economy of scale through deployment, as well as a beneficial learning curve.
Finding 4-4: Diseconomies of scale exist in certain nuclear power development and deployment scenarios. The diseconomies of scale may be owing to increased complexity and addition of systems associated with increased plant size, as well as the need for robust supply chains to support additional complexity and size.
Recommendation 4-1: The Department of Energy should continue to support developers in their efforts to design smaller plants and microreactors. This may be an early and low-risk path for nuclear deployments in certain selected applications where reduced scale may enable better control of cost and schedule overruns, potentially creating demand-pull, a larger number of orders, and greater potential for rapid learning.
In summary, the key economic challenge for advanced nuclear reactors is reduction in the up-front capital costs (i.e., sunk costs) needed to develop and deploy these technologies so that they are cost competitive with other low-carbon energy systems. Achieving cost-competitiveness would enable advanced reactors to enter the market and attract enough private investment to be deployed at scale, whether the primary goal is to provide electricity or to support industrial processes. As with most energy technologies, federal government support will be required in many phases of the research, development, and demonstration process. The next section addresses frameworks for private–public cooperation that will almost certainly be necessary to clear technical and commercialization barriers to entry for advanced nuclear. This economic challenge also translates into a financial challenge for advanced reactors: there will be limits to acquiring the necessary funds for widespread deployment of advanced reactors. The financing challenge and financing avenues are addressed after a discussion of government’s role in demonstration.
STRUCTURE AND PARAMETERS FOR PUBLIC–PRIVATE PARTNERSHIPS TO PURSUE DEMONSTRATIONS
Today, private industry is moving forward with several advanced nuclear technologies. Building on past work that began with substantial federal funding, these advanced nuclear reactor developers are aiming to produce electricity as well as other energy products beyond electricity that can potentially address a broader set of energy services. The readiness of different nuclear concepts varies, with some developers aiming for demonstration in this decade, while others target demonstrations early in the next decade.
As described in Chapter 2, each new design has different levels of technology and system readiness. Federal and state governments have thus expanded their efforts to support technology development, and many private developers have taken a pragmatic approach that recognizes their diverse development needs and have shown a willingness to incorporate aspects of private-public partnership, especially in completion of higher-risk R&D. Such a framework will be necessary to result in successful demonstration of first-of-a-kind advanced reactors.
An American Nuclear Society Task Force proposed a set of development phases for private–public partnerships that would involve teams of industry, national laboratories, and universities (ANS Task Force 2021). The Task Force report outlined the necessary phases of a technical approach that would support the ongoing advancement of nuclear energy concepts from the required applied research, the development of advanced concepts, and then deployment of these reactor concepts. This approach would (1) maintain national R&D infrastructure capabilities for these teams; (2) enable teams to innovate new reactor concepts; (3) develop promising concepts by industry for demonstration that meet technical milestones; (4) demonstrate a few select concepts; and (5) commercialize successful demonstrations for deployment.5 Such activities could be developed at a faster pace to get maximum value from public as well as private investments in this decade.
To facilitate such a development structure, development teams can employ DOE’s recently established National Reactor Innovation Center (NRIC) and the Gateway for Acceleration of Innovation in Nuclear (GAIN) programs (see Box 4-1). These programs were specifically established to connect private industry teams to federal nuclear R&D experimental facilities and analysis capabilities. These infrastructure programs support activities that would receive federal financing, such as the newly created Advanced Reactor Demonstration Program (ARDP), which is a private–public partnership.
Given this overall structure, certain key elements could be part of a private–public partnership, as suggested by Petti et al. (2017) and EPRI (2017). These reports suggest similar cost sharing concepts and financing approaches that begin with government support of R&D facilities, co-funding of R&D activities that lead to a reactor concept via private–public partnerships, and eventually industry-funded commercialization with government financial incentives. Similar approaches have been used by NASA and Space-X (Box 4-2). Figure 4-2 provides a conceptual picture of these elements, and Petti et al. (2017) note that some of these development stages may not be needed for more mature reactor concepts.
Not all advanced reactor concepts have followed this development pathway. NuScale leveraged the substantial collective experience with LWR technology to move directly to a combined performance demonstration and commercial offering. Given that a plant site may house several modules, the first nuclear power module is planned to demonstrate acceptable performance, followed by installation of the other modules as part of the commercial plant. For the high-temperature gas-cooled reactor and the sodium fast reactor, a few prior engineering demonstrations have served as proofs-of-concept. The current design concepts are relatively mature, so developers (X-Energy and TerraPower) are aiming to adopt a similar model that combines performance and commercial demonstration. In contrast, for the molten salt concepts that are not as mature (e.g., Kairos), an engineering demonstration is part of the development pathway for the vendor to reach commercial demonstration and potential deployment.
DOE is playing a key role across the advanced reactor development landscape by funding multiple designs for demonstration, risk reduction, and further research and development. This federal government support will be crucial in mitigating technical challenges that lead to risk in development and could affect the sunk cost burden. For example, these government programs involve private-public partnerships that:
- Assist the developer of mature reactor concepts in completing the detailed design and help engage the regulator to review the design and its ability to meet safety criteria.
- Reduce the risk of maturing reactor concepts in demonstrating the satisfactory performance of innovative safety systems.
- Provide R&D funding for less mature concepts to develop needed materials and fuels.
___________________
5 Note that some of these elements may not be needed for mature advanced reactor concepts. Also, the ANS description of the phases has been modified by separating the demonstration phase from the commercialization phase. Demonstration would likely involve direct financial involvement by the government in partnership with a vendor and owner-operator. Commercialization would likely involve private investment with the government providing more indirect financial incentives.
The following section summarizes these advanced reactor program efforts and Appendix D describes in detail the breadth of these DOE programs.
ADVANCED REACTOR DEMONSTRATION PROGRAM: PATH FORWARD
The Advanced Reactor Demonstration Program (ARDP)6 formally started in fiscal year (FY) 2020 and supports advanced reactor development through a collection of legacy R&D programs within DOE and through this congressionally authorized demonstration program. ARDP provides funding for two reactor demonstrations at
___________________
6 The ARDP program was funded in FY 2021 appropriations at $80 million for each demonstration project, $40 million for risk reduction efforts and $15 million for regulatory development. The FY 2022 President’s Budget increased funding for the two selected demonstration projects to $109 million and $136 million, respectively, and increased risk reduction funding to $50 million. The federal demonstration funding is a 50 percent match for the private investments by the two industrial teams for the Natrium and X-Energy reactor concepts.
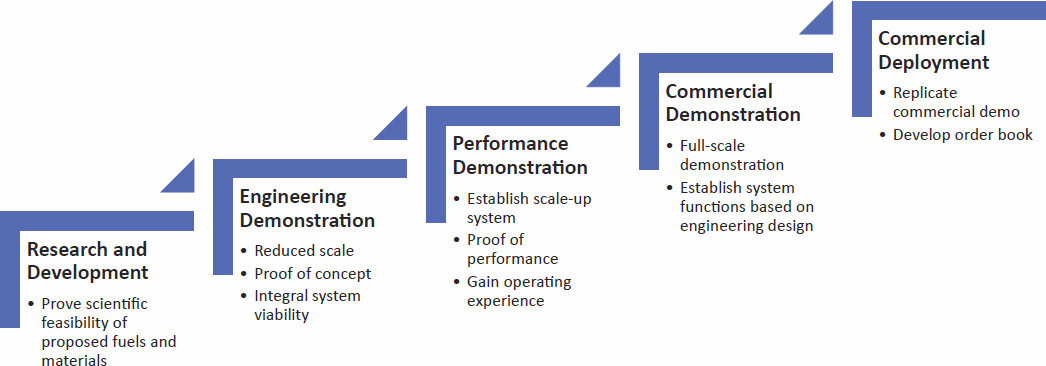
higher technology readiness levels (TRLs)7 and risk reduction for reactors at lower TRLs. As of December 2021, DOE established the Office of Clean Energy Demonstrations (OCED), which now manages the ARDP’s two reactor demonstration projects.
The overall DOE program has elements in addition to ARDP. The combined portfolio includes
- University-led research with industry and national laboratory teams;
- Industry-led research through targeted Funding Opportunity Announcements;
- Laboratory-led advanced SMR program for early-stage, crosscutting engineering technologies;
- Laboratory-led advanced reactor technology program for long-term innovative technologies; and
- ARPA-E independent programs in support of nuclear energy.
The details of the current DOE programs are discussed in Appendix D.
It is important for DOE to ensure that its investments will constitute a sustainable, innovative holistic program, not just a set of loosely coordinated individual programs. Since the initiation of ARDP as a separate program in 2020, there appears to be a need to assess and organize these multiple programs to minimize potential duplication of program goals (unless warranted for specific research objectives) and to align overall research and development as well as demonstration efforts. However, some of the necessary organizational support structures have come to exist through NRIC and GAIN, which are described in Box 4-1.
The overall structure of ARDP partnerships needs to have a comprehensive set of development phases for these private–public partnerships. A coherent progression of technology development, kept on target by assessments at key milestones with established metrics, could be organized to produce reactor concepts ready for deployment and ensure innovations in efficiency and design.
For the ARDP demonstrations to be successfully completed by the end of this decade,8 the program needs to receive full funding for these first two demonstration projects. The Bipartisan Infrastructure Bill, passed in Congress in November 2021, funded the ARDP projects only through FY 2025 (Nuclear Newswire 2021; see Appendix D). As part of the ARDP, an additional eight reactor concepts are supported by DOE for risk reduction and early-stage design. Realistically, not all these reactor concepts will see their way to eventual demonstration. Rather, DOE should plan for a down-selection to a few concepts that can proceed. The purpose of including agreed-upon milestones is to explicitly establish the criteria for the down selection.
Plant owner-operators, industry developers, and DOE as well as its laboratories need to develop a coordinated plan to support necessary development efforts for those selected designs. While initial efforts reflect a desire to broadly support multiple promising concepts, the ARDP ultimately needs a well-vetted strategic technology development plan, with performance milestones that are closely coupled with the long-range funding strategy. This plan would factor in issues such as ongoing design progress and adherence to cost and schedule and would dictate assessment against a consciously chosen set of competitive criteria to allow recurring review and support for those concepts that continue to meet cost targets, regulatory milestones, and technology development goals. As technology development progresses, it may be necessary to consider a staggered approach to demonstrations to ensure affordability for the overall program (e.g., two demonstrations through the planned 2027 timetable and two more chosen from risk-reduction potential candidates). It is in the long-term interest of DOE to ensure multiple demonstrations are completed given the uncertainty in long-term operability or affordability of any single design. Thus, it is critical that DOE remain supportive of continuing the ARDP effort, including technology risk reduction for lower TRL designs, even if one of the more mature concepts shows promise in initial demonstration. That said, it is also important that DOE adhere to a milestone approach that includes “off-ramps” for technologies that do not meet specified goals across cost, schedule, and performance.
As discussed in more detail in Appendix C, past DOE support for commercialization programs such as the NP2010 Program successfully led to commercial opportunities for more advanced light water reactor designs. Despite the success of that program in generating utility interest in deploying these new designs, one of the resulting
___________________
7 First developed by NASA in the 1980s, the Technology Readiness Level (TRL) is a type of measurement system used to assess the maturity level of a particular technology. The scale ranges from 1 (basic principles observed) through 9 (total technological system used successfully in project operations) (NASA 2021).
8 Current ARDP plans indicate a completion of these projects by 2027. This seems optimistic; the early 2030s is more realistic.
development efforts—the V.C. Summer project in South Carolina—was ultimately cancelled after billions of dollars were invested, and the second project at Vogtle in Georgia has suffered extensive schedule delays and cost overruns (see Chapter 6 for a discussion).9 While some assessment of these development programs has been completed, examining these efforts in the context of execution of the ARDP may be beneficial in order to ensure the lessons learned from the Vogtle and V.C. Summer projects are factored in. Specifically, the reexamination needs to assess issues associated with project management and civil engineering, which to date do not seem to be a prominent aspect of the ARDP, but which may have a greater bearing on ultimate commercial viability than demonstration of the fundamental nuclear technologies. The issue of project management and the importance of lessons learned is discussed in more detail in Chapter 6.
Given DOE’s efforts to demonstrate new reactors and the uncertainty that exists, vendors should aim for costs that are close to $4,000–$5,000/kWe overnight (see Chapter 3). This is also supported by past studies that suggest the need to reach similar NOAK overnight costs (EPRI 2018; Buongiorno et al. 2018). While the committee did not receive detailed cost estimates from vendors, most acknowledged a need to meet likely market thresholds of <$5,000/kWe. Some indicated that they anticipated reaching less than ~$3,000/kWe over time for a NOAK plant, suggesting that with a sufficiently large order book, learning would drive costs lower (Lovering et al. 2020). These claims were not backed by a clear assessment of how large that order book would need to be, any evidence of supply chain readiness, estimates of cost of unique fuel designs (including HALEU), or cost factors anticipated in developing a manufacturing infrastructure. Importantly, most vendors pointed to a manufacturing approach as a necessary precursor to achieving their cost reduction goals. The committee notes that the average cost to reach readiness level for demonstration for most nuclear technologies has exceeded $1 billion for each design; however, this could be consistent with a slower, structured approach to a broad future technology portfolio (Ford 2019). Prototype and demonstration plant costs have varied based on scale, but the anticipated government cost share for the ARDP effort is $3.2 billion, which will be matched by the industry participants. Given the lower plant output for the Natrium (345 mWe) and X-Energy Xe-100 (four power modules, each at 80 mWe) designs, the implied demonstration cost is likely >$10,000/kWe for these initial plants, which provides an indication of the cost reductions required to reach market competitive prices.10
Finding 4-5: Past studies suggest that for advanced reactors to achieve a strong market demand signal, it is likely that they will need to reach overnight capital costs in the range of ~$4,000–$5,000/kWe. While final costs for planned Advanced Reactor Demonstration Program (ARDP) plants are still quite uncertain, the level of government funding and vendor matching contributions (for the first-of-a-kind [FOAK] demonstrations) implies a cost level of approximately 2–2.5 times the $4,000–$5,000/kWe cost threshold. Significant and rapid learning and cost reductions will be necessary when moving from FOAK to nth-of-a-kind (NOAK) cost levels.
OTHER DOE EFFORTS TO SUPPORT TECHNICAL DEVELOPMENT
DOE has made other efforts to further the research and development of new reactor designs. While this is covered in more detail in Appendix D, some topics merit a mention here given their potential impact in overall viability of the DOE portfolio.
In the area of support for small modular reactor development, DOE continues to provide funding and material support for NuScale Power. In 2013, the company was selected as the winner of the DOE competitively bid, $226 million, 5-year, financial assistance award to develop this SMR LWR technology. In 2015, DOE awarded a $16.6 million to NuScale Power for the preparation of a combined Construction and Operating License Application (COLA) with its first customer, Utah Associated Municipal Power Systems (UAMPS) for the Carbon Free Power Project (CFPP). UAMPS plans to site a NuScale reference plant in Idaho that is expected to be operational by the end of this decade.
___________________
9 Both AP1000 projects were fixed-price contracts.
10 Natrium, a 345 MWe reactor, has total funding of $4 billion (e.g., $11,600/kWe) while X-Energy, a 320 MWe design, has funding of $2.464 billion (e.g., ~$7,700/kWe; combined DOE and company match). Source: GAO-22-105394 of September 2022 “DOE Should Institutionalize Oversight Plans for Demonstrations of New Reactor Types.”
DOE also has ongoing efforts to provide national R&D testbeds in support of advanced reactor R&D. These testbeds involve cutting-edge experimental capabilities, computational capabilities, and databases, and staffing those activities with qualified people. Current experimental test beds include the Advanced Test Reactor (ATR), the Transient Reactor Test Facility (TREAT), and the High Flux Isotope Reactor (HFIR), and require appropriate federal and industry support to maintain their capabilities. The other program that has generated significant funding and development is the Versatile Test Reactor. However, ongoing Congressional budget deliberations have placed the VTR at risk, highlighting the challenge that DOE faces in attempting to support such a breadth of programs within the DOE-NE budget.
Last, two major programs in nuclear energy are currently funded through a sub-office within DOE—the Advanced Research Projects Agency–Energy (ARPA-E). The Modeling-Enhanced Innovations Trailblazing Nuclear Energy Reinvigoration (MEITNER) program was created to develop innovative technologies that can enable advanced reactor designs to achieve lower cost and safer operation. These enabling technologies could establish the basis for a modern, domestic supply chain supporting nuclear technology. The MEITNER program began in summer of 2018 and made 10 awards totaling $24 million. The Generating Electricity Managed by Intelligent Nuclear Assets (GEMINA) program seeks to develop digital twin technology for advanced nuclear reactors and transform operations and maintenance (O&M) systems for advanced nuclear plants (explained further in Chapter 6). The GEMINA program began in summer of 2019 and made 10 awards totaling $27 million. Given the increasing funding being allocated to advanced reactor research by ARPA-E, it will be vital that the goals of this office remain well aligned with the future vision of the broader DOE-NE funded efforts.
While there are many efforts under way to support technical development, the current DOE portfolio is not structured to continuously move ideas from basic discovery to deployment and, as recently detailed by the Government Accountability Office, has not incorporated independent reviews of ARDP project performance nor demonstrated the ability to make funding decisions based on accomplishment of critical development milestones.11
Finding 4-6: The Department of Energy (DOE), Office of Nuclear Energy, and Office of Clean Energy Demonstration portfolios support national infrastructure, databases, and human resources; basic discovery research; concept development and improvement; and support for demonstrations. As a means of reducing risk against an uncertain future, the portfolio entertains a diversity of approaches and designs. At the same time, strong evaluation criteria are warranted for deciding on programmatic elements subject to careful consideration of realistic budget constraints. The current DOE portfolio is not structured to continuously move ideas from basic discovery to deployment and has not incorporated independent reviews of Advanced Reactor Demonstration Program (ARDP) project performance nor demonstrated the ability to make funding decisions based on accomplishment of critical development milestones. There is a risk that future funding instability and lack of rigorous oversight may result in a failure to achieve the program goal of demonstrating multiple nuclear technologies that can be commercialized.
Recommendation 4-2: The nuclear industry and the Department of Energy’s Office of Nuclear Energy should fully develop a structured, ongoing program to ensure the best performing technologies move rapidly to and through demonstration as measured by technical (testing, reliability), financial (cost, schedule), regulatory, and social acceptance milestones. Concepts that do not meet their milestones in the ordinary course should no longer receive support and newer concepts should be allowed to enter the program in their place.
Recommendation 4-3: Congress and the Department of Energy (DOE) should maintain the Advanced Reactor Demonstration Program (ARDP) concept. DOE should develop a coordinated plan among owner/operators, industry vendors, and the DOE laboratories that supports needed development efforts. The ARDP plan needs to include long-range funding linked to staged milestones; ongoing design, cost, and schedule reviews; and siting and community acceptance reviews. This plan will help DOE downselect among concepts for continued support toward demonstration. A modification in the demonstration
___________________
11 GAO-22-105394 of September 2022, “DOE Should Institutionalize Oversight Plans for Demonstrations of New Reactor Types.”
schedule that takes a phased (versus concurrent) approach to reactor demonstration may be required. For example, funding would be continued for the first two demonstrations under the ARDP. A second round of demonstrations of designs expected to mature from the current ARDP Risk Reduction for Future Demonstrations award recipients could be funded for demonstration under an “ARDP 2.0” starting thereafter and going into the future.
ADVANCED REACTOR COMMERCIALIZATION PROGRAM
The preceding section described government efforts to support technical development of advanced reactor technologies through R&D support programs. Even if programs such as ARDP are successful in demonstrating the possible viability of advanced reactors, the overall aim of the effort would not be achieved unless the barriers to wide scale deployment are overcome.
It is appropriate in this context to consider the financial challenge in terms of the overall commitment to aggressive climate goals. Decarbonizing the global energy system is estimated to require world capital investments on physical assets of about $275 trillion through 2050 (Krishnan et al. 2022). Electric infrastructure alone is estimated to require investment on the order $800 billion per year for much the period from 2030 to 2050 (IEA 2021b). Achieving net zero in the United States is estimated to require at least at least $2.5 trillion in additional capital investment (relative to business as usual) in energy supply, industry, buildings, and vehicles over the next decade (Larson 2021). While these estimates are very uncertain, they reflect the likelihood that an immense amount of capital will be required. In the United States and similar economies, a large share of the financing will have to be marshalled by private investors through traditional equity and debt markets. Funding on the scale that is needed for widespread commercialization is simply not available in the venture capital and private equity markets.
To attract adequate funding from the standard public markets, an investment must present sufficiently low risk that large-scale financing can compete with other “ordinary” investments. As a result, an investor’s perceptions of risk will greatly influence the evolution of the energy system. A technology option might appear to generate a lower-cost electricity portfolio to an analyst who is building a large-scale optimization model, but prudent investors will consider the credibility of a technology solution, the magnitude of the risks, and the incentives that are offered to support it. Understanding how capital is likely to flow to support different options—including advanced nuclear power—requires listening to investors and their assessments of credibility.
The credibility of a technology option is a function of a hard-nosed assessment of costs and markets, the historical deployment record, technological readiness, and the magnitude and impacts of uncertainties. In this respect, advanced technologies are, by definition, riskier bets than established ones. In assessing the credibility of an emerging technology option, investors often consider the historical record. How successful have the developers been in commercializing (and selling) past technologies? How much of their own equity have they invested in the new technology, and are these investments bearing fruit? Are there risks that could constrain deployment even if the technology operates within its expected performance envelope, and is the developer serious in its effort to mitigate those risks? And who will bear completion risk, particularly for FOAK units?
In this respect, investments in capital-intensive energy infrastructure, such as nuclear power, are at a disadvantage. Across countries and sectors, there is a poor record of cost and schedule control with most such megaprojects (Flyvbjerg 2014; Garemo 2015; Olaniran et al. 2015; Callegari et al. 2018; Vartabedian 2021). Concerns are likely to be given special prominence in connection with nuclear projects given the experience with recent construction projects. in the United States and Europe. The demands on an organization—both hard, in the form of resources and manpower, and soft, in the form of managerial skills and a high reliability mind-set—are high. This matter is covered further in Chapter 6.
To compete on the technical merits with other electricity generation options, advanced reactors must demonstrate potential for delivery of products that are smaller, less capital-intensive, less risky, and more socio-politically attractive, and offering various generation options. Advanced reactors must also demonstrate more favorable costs, greater buy-in from competent and deep-pocketed energy infrastructure developers, and lower system integration and socio-technical risks than large reactors have been able to accomplish. The fact that large LWRs beyond the Vogtle reactors are not being considered in the United States today demonstrates a clear failure by the conventional industry to compete on those attributes. This is in contrast to other parts of the world where large-scale LWRs are being delivered on time and at costs that are competitive with other electricity generation options.
If the technical merits are insufficient to steer funds toward large-scale deployment, the proponent often turns to politics and policy for aid. But credibility matters in this sphere also. The policies on offer to support an emerging technology must be credible to an investor. Credibility is a multifaceted concept: credible incentives offer upfront rather than delayed support and they offer unconditional rather than conditional support.
Renewable energy technologies have succeeded over many years in securing production and investment tax credits and preferential take-off arrangements as part of a concerted effort to encourage the large-scale deployment of these energy resources. These policies have constituted credible signals to investors of policy support for these technologies; the prevalent messaging in favor of expanded renewable deployment certainly contributed to enhancing credibility as well. The availability of similar incentives for nuclear power in the Inflation Reduction Act (IRA) may have a similar impact on investor confidence in nuclear technology.
To lay the groundwork for expanded adoption of new and advanced nuclear reactors, it is necessary to consider three facts. First, credibility is key: it is the prism through which most investors assess low-carbon investment opportunities and the policy instruments that encourage deep decarbonization. Second, new and advanced nuclear reactors must strive to secure credible incentives if the goal is to boost investor interest. This might warrant less faith in politically fragile incentives that generate delayed benefits, like carbon pricing, and more faith in incentives that retire system risks, that serve to reduce the disincentive associated with capital intensity and that facilitate low-cost debt financing. The recent IRA may change this dynamic but only time will tell if the additional government support drives a change in investor confidence in the technology. Third, although investors might endorse the role of nuclear in decarbonization and may remain participants in the conversation surrounding nuclear power, they will be shrewd in their capital allocations even if they are proponents of the technology.
With these factors in mind, there is a need to confront various risks associated with the commercialization of advanced nuclear projects, and the future landscape in which they may play a role. Fundamentally, the projects must convincingly and honestly demonstrate that they can compete in a marketplace in which there are alternatives. For deployment in the United States, they must demonstrate a pathway to overcome the concerns that legitimately arise from the historical cost and schedule performance of previously built reactors. These commercialization risks are presented in Box 4-3.
Two aspects bearing on the evaluation of the financial opportunity for advanced reactors require specific mention. First is the evaluation of the opportunities for nuclear power to provide alternative products. In an energy system with extensive renewables, nuclear power may be used to provide firm electricity generation at times when sufficient renewables are unavailable to meet grid needs. In such a situation, the economics of the plant can be highly dependent on whether other energy products—for example, industrial process heat, hydrogen and synfuels, or desalination—can be produced in the periods when the output of the nuclear plant is not dispatched to the grid.12 Some vendors anticipate that their designs will be entirely devoted to these alternative products. The financial evaluation of these opportunities is particularly complex because it requires not only an understanding of the risks of the reactor and of any ancillary systems to produce the alternative product, but also of the future market for the alternate products. For example, the high-temperature output from an advanced reactor may be particularly well suited to produce hydrogen, but how large will the market for hydrogen be and within what time frame and at what price? These alternative products are discussed in more detail in Chapter 5.
Second, an evaluation of the opportunities for international sales may be an essential element in the evaluation of the financial opportunity for advanced nuclear reactors. All the vendors hope for substantial sales as a means to exploit learning to drive down costs. Some vendors anticipate factory production of reactors to reduce costs, enhance efficiency, and improve quality. But a large number of sales is required to justify the construction of a factory. In light of these considerations, the global market for a vendor’s product must be part of the economic evaluation. This introduces additional complexity because it requires an understanding not only of the domestic market, but also an evaluation of the opportunities, competition, and incentives in foreign markets. International markets are discussed in detail in Chapter 10.
___________________
12 A recent study by the OECD Nuclear Energy Agency provides context and significant detail about the viability of nuclear in co-generation, covering a wide range of alternatives such as hydrogen production, desalination, and district heating (NEA 2022).
Government Incentives
There likely will be a need for government assistance in developing the finance structures and market incentives to help these emerging nuclear technologies make entry into the market. Some have argued that the federal government should refrain from establishing policies that influence the market. However, some targeted economic incentives may be necessary because the market does not always properly value certain important externalities such as climate change, system costs, and low emissions of other pollutants. As the advanced reactor technologies move beyond demonstrations, incentives may be necessary to enable advanced reactors to become an integral part of a carbon-free energy system in the United States. Such commercial incentives have allowed the penetration of renewables, and the same model should be followed for other low-carbon technologies, including advanced reactors.
These incentives and structures would need to be tailored for specific markets, and some may be more or less viable than others depending on prevailing market parameters. Deployment mechanisms exist that could create a clear and durable market signal for the commercialization of advanced reactors, several of which are briefly described below.
Loan Guarantees.
Commercial lenders are often unwilling or unable to take on the risk of supporting the deployment of a new technology until it has a clear market demand and solid history of commercial operation. DOE is authorized to issue loan guarantees pursuant to Title 17 of the Energy Policy Act of 2005 (DOE n.d.).13 Eligible projects for the Title 17 program must use a new or improved technology located in the United States that avoids, reduces, or sequesters greenhouse gases. In addition, the project needs to be credit worthy with a reasonable chance of repayment. Once the technology is proven at commercial scale through the first few projects, DOE stops providing financing and lets the private market take over. This program is intended to back investments that have financial risk, and some projects supported by the program should be expected to fail. To date, the DOE loan program has not lost money because the successful projects have more than compensated for the losses. Updates to the Loan Guarantee program were included in the Inflation Reduction Act of 2022. This included $40 billion in new loan guarantee authority— which is available for nuclear facilities—and another $250 billion in loan guarantee cap which is also available for some nuclear-related facilities. The Act also appropriated significant sums including $3.6 billion to cover borrowing costs and $5 billion for loan subsidies.14
Power Purchase Agreements (PPAs).
The financial risk associated with an advanced reactor can be alleviated if the operator can obtain a long-term contract by which a customer agrees to pay for plant output at a specified price (e.g., $/MWh). This retires the risk associated with selling into a market with uncertain prices and offers potential customers with certainty that the output will be available at a specified price. In addition, the government (federal, state, or local) may help supplement the cost of power for the new energy technology by paying the differential cost above what would be current cost for proven energy technologies (e.g., natural gas) or may provide for a longer guaranteed period of purchase to incentivize the development of the new technology. There are challenges with the use of a PPA. If the customer is a government entity, most federal power purchase contracts (outside the Department of Defense) are currently limited to 10 years (GSA 2020), while lenders and facility owners might desire contracts as long as 40 years in order to be sure that they can recover their investment.15
Strike Price Agreement.
This contractual vehicle is similar to a PPA and provides for a fixed price (strike price) for the electrical power supplied by the reactor. It is being used for the construction and eventual operation of an EDF European Pressurized Water Reactor (EPR) at Hinkley Point C (HPC) in the United Kingdom (Morison 2021). In strike price agreements, the government will supplement revenue based on this price if wholesale electricity prices are lower than this level, but the owner-operator will have to pay the government the price difference if wholesale prices are higher.
___________________
13 Details of the current program can be found in this DOE Fact Sheet (DOE 2020).
14 As originally configured, the loan program required the applicant to pay an upfront fee to cover the risk of default. This significantly reduced the attractiveness of loan guarantees for novel projects that presented high risk. The loan subsidies cover this fee and thus make the loan program more attractive for applicants.
15 Because the Department of Defense already allows long-term PPAs (up to 30 years), consideration should be given to targeting the defense facility market as a leading opportunity for new designs. Carbon-free electricity has been a focus area across most military branches though most have not seriously considered a nuclear power alternative owing to lower peak demand which would make even an SMR too large in most cases (DOA 2022).
Investment Tax Credit.
Investment Tax Credits have been used to benefit solar energy projects since 2006; currently 26 percent of the project cost (commercial or residential) can be used as a credit in 2021 (SEIA 2021). A significant portion of the cost of a solar energy project can be recovered as a credit against federal income taxes. The credit is not refundable, but it can be carried back for one year or carried forward for 20 years, enabling the credit to be applied in years in which the beneficiary has income taxes. Such an economic incentive is an obvious stimulus to investment in solar projects and could be applied to nuclear project investments as well.
Production Tax Credit.
Production tax credits are fixed at a prespecified unit amount ($/MWh) and awarded for the quantity of electricity generated over a time span. Typically, an upper limit is set on the quantity of production that can receive the production credit. Payment is made when energy production occurs—for example, when electricity is sold onto the grid. The owner of the energy production facility (e.g., nuclear) receives both the current market price for the electricity and the supplementary production credit. This has proven to be a valuable incentive for renewable energy technologies like wind and solar (EPA 2020). The renewable production credits range from 13–25 $/MWh for a time span of 10 years (26 U.S.C. § 45J; NC Clean Energy Technology Center 2022).
A production credit rewards performance, with the result that the owner-operator bears the risk of building and operating the advanced reactor technology. If the advanced reactor is never completed, the supplementary production credit will not be earned. If the reactor is completed with delays or at greater cost than originally estimated, the size of the credit is not adjusted, and the developer and/or the owner earns a smaller profit or suffers a loss. This creates a strong incentive for private investors to guide the innovation process in the most cost-efficient direction.
The 2022 Inflation Reduction Act created a zero-emission nuclear power production tax credit (PTC) aimed at preventing the decommissioning of existing nuclear plants. The Nuclear PTC is available with respect to existing nuclear plants for electricity produced and sold for taxable years beginning after December 31, 2023, and before December 31, 2032.
The IRA also modified the U.S. tax code in a way that may allow advanced reactors to qualify for the technology-neutral clean electricity production tax credit (the Clean Electricity PTC). It does so by changing the definition of “qualified facility” to mean any plant that is placed into service after December 31, 2024, and produces zero greenhouse gas emissions. Because eligibility is based on emissions rates instead of generation technology, nuclear facilities may use the Clean Electricity PTC.
Last, the IRA established a Clean Hydrogen PTC. The Act funded the development of a number of clean hydrogen hubs around the country and at least one of them must use nuclear power to produce clean hydrogen.
Construction Work in Progress (CWIP).
The recovery of the costs of an NPP in most regulated markets commences once the plant goes into operation. That is, the recovery of all the costs for constructing and operating the plant are recovered in the rates paid by rate payers during operations. These costs thus include the substantial interest costs that have been accrued over the many years of construction. If recovery of interest costs from ratepayers during the period before a plant goes into operation is allowed, the total cost of the plant is reduced (through the elimination of the interest on the interest) and some of the risk of the project is shifted from the investors to the ratepayers. CWIP has often proved unpopular with ratepayers because it serves to shift risk and results in increased rates before there is contemporaneous electricity production from the nuclear plant. Ratepayer education and buy-in is extremely important to utilize this financial tool. The Vogtle plant has the benefit of recovery of CWIP, subject to some limitations imposed by the Georgia Public Utility Commission.
IRA Energy Communities.
The Inflation Reduction Act provides a 10 percent enhancement of tax credits for clean-energy projects in an “energy community.” Among several criteria of eligibility for this bonus is a project be located in a census tract within which a coal power plant has closed since 2010. Several vendors of advanced reactors have examined the siting of new reactors at retired coal plants and these coal-to-nuclear projects may be eligible for this enhancement of tax credits.
Carbon Tax or a Clean Energy Portfolio.
In principle, equal treatment of a wide range of energy technologies could be implemented through a uniform carbon tax or through a requirement to deploy a portfolio of clean energy technologies. Various social costs have been associated with the production of CO2 (EPA 2021), and multiple proposals have been offered to either tax carbon emissions or otherwise provide incentives for technologies that
do not emit CO2.16 As noted above in the section on Production Tax Credits, the recent passage of the IRA finally placed nuclear on a level playing field with respect to other low carbon generators. Despite strong proponents, it has proven politically impossible to establish a carbon tax. And a carbon tax or a portfolio standard has the disadvantage that it favors established technologies, whereas a focused program of technology-related incentives creates new options. The IRA reflects a welcome signal by the Congress to facilitate a transition to a low carbon future by providing incentives for all low-carbon technologies while avoiding the political difficulties associated with a tax.
Recommendation 4-4: To enable a cost-competitive market environment for nuclear energy, federal and state governments should provide appropriately tailored financial incentives (extending and perhaps enhancing those provided recently in the Inflation Reduction Act) that industry can use as part of a commercialization plan, consistent with the successful incentives provided to renewables. These tools may vary by state, locality, and market type. Continued evaluation of the recently passed incentives will need assessment to determine their adequacy. The scale of these incentives needs to be sufficient not only to encourage nuclear projects but also the vendors and the supporting supply chains.
REFERENCES
26 U.S. Code § 45J—Credit for Production from Advanced Nuclear Power Facilities. 2014. https://www.law.cornell.edu/uscode/text/26/45J.
Abdulla, A., M.J. Ford, M.G. Morgan, and D.G. Victor. 2017. “A Retrospective Analysis of Funding and Focus in U.S. Advanced Fission Innovation.” Environmental Research Letters 12(8):084016. https://doi.org/10.1088/1748-9326/aa7f10.
American Nuclear Society Task Force on Public Investment in Nuclear Research and Development. 2021. The U.S. Nuclear R&D Imperative. La Grange Park, IL: American Nuclear Society. https://www.ans.org/file/3177/2/ANS%20RnD%20Task%20Force%20Report.pdf.
Bistline, J. 2021. Powering Decarbonization: Strategies to Achieve Net-Zero Emissions in the Electric Sector and Beyond. Palo Alto, CA: Electric Power Research Institute.
Black and Veatch. 2012. Cost and Performance Data for Power Generation Technologies. Black & Veatch Holding Company.
Boardman, R., C. Rabiti, S. Hancock, D. Wendt, K. Frick, S. Bragg-Sitton, H. Hu, et al. 2019. Evaluation of Non-Electric Market Options for a Light-Water Reactor in the Midwest. Light Water Reactor Sustainability Program. Idaho Falls, ID: Idaho National Laboratory. https://doi.org/10.2172/1559965. INL/EX—19-55090-Rev000.
Buongiorno, J., M. Corradini, J. Parsons, and D. Petti. 2018. The Future of Nuclear Energy in a Carbon-Constrained World. Cambridge, MA: Massachusetts Institute of Technology Energy Initiative.
Chemnick, J. 2021. “Cost of Carbon Pollution Pegged at $51 a Ton.” Scientific American; E&E News, March 1. https://www.scientificamerican.com/article/cost-of-carbon-pollution-pegged-at-51-a-ton.
Clack, C.T.M., A. Choukulkar, B. Coté, and S.A. McKee. 2022. Role of Electricity Produced by Advanced Nuclear Technologies in Decarbonizing the U.S. Energy System. Boulder, CO: Vibrant Clean Energy.
Demick, L.E. 2011. NGNP Project 2011 Status and Path Forward. Idaho Falls, ID: Idaho National Laboratory. https://doi.org/10.2172/1035900. INL/EXT-11-23907.
DOA (Department of the Army), Office of the Assistant Secretary of the Army for Installations, Energy and Environment. 2022. “United States Army Climate Strategy.” Washington, DC. https://www.army.mil/e2/downloads/rv7/about/2022_army_climate_strategy.pdf.
DOE (Department of Energy). 2020. “Advanced Nuclear Energy Loan Guarantees.” Department of Energy Loan Programs Office. https://www.energy.gov/sites/default/files/2020/01/f70/DOE-LPO-Nuclear-Energy-Jan2020.pdf.
DOE. 2021. “Budget (Justification and Supporting Documents).” https://www.energy.gov/cfo/listings/budget-justification-supporting-documents.
DOE. n.d. “Advanced Nuclear Energy Projects Loan Guarantees.” https://www.energy.gov/lpo/advanced-nuclear-energy-projects-loan-guarantees.
___________________
16 In January 2021, President Biden issued Executive Order 13990, which reestablished the Intergovernmental Working Group (IWG) and directed it to ensure that SC-GHG estimates used by the U.S. government reflect the best available science and recommendations of the National Academies (NASEM 2017) and work toward approaches that take account of climate risk, environmental justice, and intergenerational equity. The guidelines supporting the execution of the new Executive Order are covered in Technical Support Document: Social Cost of Carbon, Methane, and Nitrous Oxide Interim Estimates under Executive Order 13990.
DOE-NE (Office of Nuclear Energy). 2010. Next Generation Nuclear Plant: A Report to Congress. Washington, DC: Department of Energy.
EIA (U.S. Energy Information Administration). 2014. Behavioral Economics Applied to Energy Demand Analysis: A Foundation. Washington, DC: Department of Energy.
EIA. 2019. International Energy Outlook 2019 with Projections to 2050. Washington, DC.
EIA. 2020. Capital Cost and Performance Characteristic Estimates for Utility Scale Electric Power Generating Technologies. Washington, DC.
EIA. 2021. Electric Power Annual 2020. Washington, DC.
EIA. 2022. “Monthly Energy Review: Table 7.2a: Electricity Net Generation: Total (All Sectors).” https://www.eia.gov/totalenergy/data/browser/index.php?tbl=T07.02A#/?f=A.
EPA (U.S. Environmental Protection Agency). 2015. “Fact Sheet: Clean Power Plan—Opportunities for Nuclear Power.” https://archive.epa.gov/epa/cleanpowerplan/fact-sheet-clean-power-plan-opportunities-nuclear-power.html.
EPA. 2016. “Social Cost of Carbon Fact Sheet.” https://19january2017snapshot.epa.gov/climatechange/social-cost-carbon-fact-sheet.
EPA. 2020. “Renewable Electricity Production Tax Credit Information.” https://www.epa.gov/lmop/renewable-electricity-production-tax-credit-information.
EPRI (Electric Power Research Institute). 2016. Advanced Nuclear Technology: Using Technology for Small Modular Reactor Staff Optimization, Improved Effectiveness, and Cost Containment. 3002007071. Palo Alto, CA.
EPRI. 2017. Program on Technology Innovation: Expanding the Concept of Flexibility for Advanced Reactors, Refined Criteria, a Proposed Technology Readiness Scale, and Time-Dependent Technical Information Availability. 3002010479. Palo Alto, CA.
EPRI. 2018. Exploring the Role of Advanced Nuclear in Future Energy Markets: Economic Drivers, Barriers, and Impacts in the United States. 3002011803. Palo Alto, CA.
Eurostat. 2022. “Nuclear Energy Statistics.” https://ec.europa.eu/eurostat/statistics-explained/index.php?title=Nuclear_energy_statistics.
Ford, M.J., and D.P. Schrag. 2019. “The Case for a Tortoise Approach to U.S. Nuclear Research and Development.” Energy Policy 135(December):111013. https://doi.org/10.1016/j.enpol.2019.111013.
Ford, M.J., A. Abdulla, M.G. Morgan, and D.G. Victor. 2017. “Expert Assessments of the State of U.S. Advanced Fission Innovation.” Energy Policy 108(September):194–200. https://doi.org/10.1016/j.enpol.2017.05.059.
GSA (U.S. General Services Administration). 2020. “For Federal Agency Customers.” https://www.gsa.gov/real-estate/environmental-programs/energy-water-conservation/areawide-public-utility-contracts/for-federal-agency-customers.
Ingersoll, E., K. Gogan, J. Herter, and A. Foss. 2020a. Cost and Performance Requirements for Flexible Advanced Nuclear Plants in Future U.S. Power Markets. Report for the ORNL resource team supporting ARPA-E’s MEITNER Program. LucidCatalyst LLC. https://doi.org/10.2172/1646858.
Ingersoll, E., K. Gogan, J. Herter, and A. Foss. 2020b. The ETI Nuclear Cost Drivers Project: Full Technical Report. Energy Systems Catapult and LucidCatalyst. https://www.lucidcatalyst.com/eti-nuclear-cost-drivers-full.
INL (Idaho National Laboratory). 2004. U.S. Job Creation Due to Nuclear Resurgence in the United States. Idaho Falls, ID.
INL. 2008. Next Generation Nuclear Plant Project Preliminary Project Management Plan. PLN-2489; INL/EXT-05-00952. Idaho Falls, ID.
INL. 2022. “Systems Analysis and Integration.” https://fuelcycleoptions.inl.gov/SitePages/CostBasisReport.aspx.
Jenkins, J.D., and N.A. Sepulveda. 2017. Enhanced Decision Support for a Changing Electricity Landscape: The GenX Configurable Electricity Resource Capacity Expansion Model. Cambridge, MA: Massachusetts Institute of Technology Energy Initiative.
Jenkins, J.D., Z. Zhou, R. Ponciroli, R.B. Vilim, F. Ganda, F. de Sisternes, and A. Botterud. 2018. “The Benefits of Nuclear Flexibility in Power System Operations with Renewable Energy.” Applied Energy 222(July):872–884. https://doi.org/10.1016/j.apenergy.2018.03.002.
Kahneman, D., and I. Ritov. 1994. “Determinants of Stated Willingness to Pay for Public Goods: A Study in the Headline Method.” Journal of Risk and Uncertainty 9(1):5–37.
Kim, S.H. 2022. Scenarios of Nuclear Energy Use in the United States for the 21st Century. PNNL-33234.
Krishnan, M., H. Samandari, J. Woestzel, S. Smit, D. Patchod, D. Pinner, et al. 2022. The Net Zero Transition: What It Would Cost, What It Could Bring. McKinsey & Company. https://www.mckinsey.com/~/media/mckinsey/business%20functions/sustainability/our%20insights/the%20net%20zero%20transition%20what%20it%20would%20cost%20what%20it%20could%20bring/the-net-zero-transition-executive-summary.pdf?shouldIndex=false.
Larson, E., C. Greig, J. Jenkins, E. Mayfield, A. Pascale, C. Zhang, et al. 2021. Net-Zero America: Potential Pathways, Infrastructure, and Impacts. Final Report. Princeton, NJ: Princeton University Press. https://www.dropbox.com/s/ptp92f65lgds5n2/Princeton%20NZA%20FINAL%20REPORT%20%2829Oct2021%29.pdf?dl=0.
Lo, C. 2020. “Could the Regulated Asset Base Model Unlock UK Nuclear?” Power Technology. Originally published November 14, 2019; updated July 15, 2020. https://www.power-technology.com/features/regulated-asset-base-model-uk-nuclear.
Longnecker and Associates. 2012. Nuclear Power 2010 Program: Combined Construction and Operating License and Design Certification Demonstration Projects Lessons Learned Report. Washington, DC: Department of Energy.
Lovering, J., et al. 2020. “Chasing Cheap Nuclear: Economic Trade-Offs for Small Modular Reactors.” The Bridge 50(3):40–46. https://www.nae.edu/239267/Chasing-Cheap-Nuclear-Economic-TradeOffs-for-Small-Modular-Reactors.
Morison, R. 2021. “U.K. Power Is So High That EDF Hinkley Reactor Looks Good Value.” Bloomberg.Com, July 30. https://www.bloomberg.com/news/articles/2021-07-30/u-k-power-is-so-high-that-edf-hinkley-reactor-looks-good-value.
NASA (National Aeronautics and Space Administration). 2021. “Technology Readiness Level.” https://www.nasa.gov/directorates/heo/scan/engineering/technology/technology_readiness_level.
NASEM (National Academies of Sciences, Engineering, and Medicine). 2022. Merits and Viability of Different Nuclear Fuel Cycles and Technology Options and the Waste Aspects of Advanced Nuclear Reactors. Washington, DC: The National Academies Press. https://doi.org/10.17226/26500.
National Research Council. 2008. Review of DOE’s Nuclear Energy Research and Development Program. Washington, DC: The National Academies Press. https://doi.org/10.17226/11998.
NC (North Carolina) Clean Energy Technology Center. 2022. “Renewable Electricity Production Tax Credit (PTC).” https://programs.dsireusa.org/system/program/detail/734.
NEA (Nuclear Energy Agency). 2022. Beyond Electricity: The Economics of Nuclear Cogeneration. NEA No. 7363. Paris: Organisation for Economic Co-operation and Development.
Nicholson, W., and C. Snyder. 2012. Microeconomic Theory: Basic Principles and Extensions. 11th ed. Mason, Ohio: SouthWestern/Cengage Learning.
NRC (Nuclear Regulatory Commission). 2021a. “NRC Issues License to Interim Storage Partners for Consolidated Spent Nuclear Fuel Interim Storage Facility in Texas.” NRC News, September 13. https://www.nrc.gov/reading-rm/doc-collections/news/2021/21-036.pdf.
NRC. 2021b. “Consolidated Interim Storage Facility (CISF)” https://www.nrc.gov/waste/spent-fuel-storage/cis.html.
Nuclear Newswire. 2021. “Trillion-Dollar Infrastructure Bill Passes House.” Nuclear Newswire, November 8. https://www.ans.org/news/article-3413/trilliondollar-infrastructure-bill-passes-house.
NuScale. 2021. NuScale SMR Technology An Ideal Solution for Repurposing U.S. Coal Plant Infrastructure and Revitalizing Communities.
Petti, D., R. Hill, J. Gehin, H. Gougar, G. Strydom, T. O’Connor, F. Heidet, et al. 2017. “A Summary of the Department of Energy’s Advanced Demonstration and Test Reactor Options Study.” Nuclear Technology 199(2):111–128. https://doi.org/10.1080/00295450.2017.1336029.
Ritchie, H., and M. Roser. 2020. “Energy.” Our World in Data. https://ourworldindata.org/energy.
Savage, I. 1993. “Demographic Influences on Risk Perceptions.” Risk Analysis 13(4):413–420. https://doi.org/10.1111/j.1539-6924.1993.tb00741.x.
SEIA (Solar Energy Industries Association). 2021. “Solar Investment Tax Credit (ITC).” https://www.seia.org/initiatives/solar-investment-tax-credit-itc.
St. John, J. 2020. “7 Transmission Projects That Could Unlock a Renewable Energy Bounty.” Greentech Media, April 9. https://www.greentechmedia.com/articles/read/9-transmission-projects-laying-the-paths-for-cross-country-clean-energy.
Talabi, S.M., and P. Fischbeck. 2015. “Advancing Risk Management in Nuclear Power Plant EPC Projects: An Empirical Evaluation of Risk Management Practices on Steam Generator Replacement Projects.” Pp. 545–557 in Proceedings of the 7th World Congress on Engineering Asset Management, edited by W.B. Lee, B. Choi, L. Ma, and J. Mathew. https://doi.org/10.1007/978-3-319-06966-1_49.
U.S. DOE Nuclear Energy Research Advisory Committee and Gen IV International Forum. 2002. A Technology Roadmap for Generation IV Nuclear Energy Systems. GIF-002-00. https://www.gen-4.org/gif/jcms/c_40481/technology-roadmap.
World Nuclear Association. 2022. “Economics of Nuclear Power Economics.” https://world-nuclear.org/information-library/economic-aspects/economics-of-nuclear-power.aspx.