2
The Uninhabited Air Vehicle as a System
To identify key technologies for future UAVs, the air vehicle system design should be considered as a whole. Although UAVs in operational environments will be part of a larger family of systems that could include multiple UAV types, manned combat and surveillance aircraft, communications satellites, and remote command and control centers, the focus of this chapter is on the UAV as a system capable of working within the future environment. Up to now, UAVs have generally been developed as advanced concepts technical demonstrators (ACTDs), with an emphasis on mission payloads. This practice has placed the design of UAVs beyond the DOD procurement process for weapon systems and enabled developers to apply available advanced technologies aggressively (CBO, 1998). The ACTD approach to technology development includes the following steps:
-
creating a point design for a system that satisfies mission requirements
-
defining the differences between currently available technology and the point design
-
establishing a development program to address the differences
This approach has been effective in the near term but has provided only limited opportunities for fundamental technology development and has favored adaptations of available technologies. In effect, the short design-cycle times and limited budgets for fundamental research and technology development has inhibited the development of technologies optimized for UAVs.
Recommendation. The U.S. Air Force should establish a research and development program to develop fundamental technologies that will advance the use of
UAVs by enabling them to carry out unique missions or by providing significant cost savings.
The committtee’s recommended approach is shown schematically in Figure 2-1. First, requirements for a range of missions and system attributes should be established with a focus on key air-vehicle concepts. Next, technologies that can address the requirements should be identified and technology forecasts and trends for applicable technology areas developed. Finally, the research on the required technologies should be initiated. Fundamental research and technology development will be required to make advanced technologies generally available so that military UAVs can be developed with significantly lower system development costs. The goal should be to develop enabling technologies for a range of UAVs.
This approach has two principal advantages. First, the development of advanced technology is separated from the development of the UAV system so that basic research and technology development can be undertaken in a more realistic time frame. Second, the recommended approach allows revolutionary advances to be pursued for implementation in future systems. The balance of this report demonstrates the value of the recommended approach for developing technological needs and suggested research and technology development for a range of UAV systems.
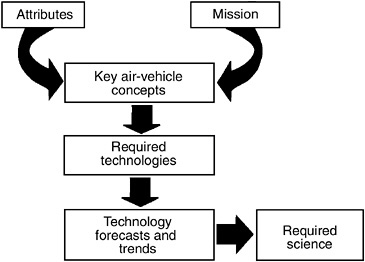
FIGURE 2-1 Recommended approach to technology prioritization. Source: Adapted from Lang, 1998.
DESIGN DRIVERS
The committee identified several general characteristics or trends that would drive all aspects of the UAV system design. Most of these characteristics have to do with the competing motivations for developing UAVs for defense applications—unique mission capabilities and significantly reduced life-cycle costs (including acquisition, operation, and sustainment costs). In general, the development of UAVs is being driven by a combination of “mission pull” (e.g., risk avoidance and cost avoidance), which requires that systems be developed for certain missions, and “technology push,” which is fueled by advances in particular technologies (e.g., microelectromechanical systems [MEMS], electronics, and composites). The committee feels that the following considerations will drive the development of future UAVs:
-
UAVs will be smaller, easier to maintain, and have lower peacetime operational costs than inhabited military aircraft.
-
To maintain low peacetime operational costs (e.g., maintenance and field support costs), UAVs may be stored for long periods of time, with little use except during combat.
-
Continued development of small precision weapons, higher levels of automatic control, and improved human-machine interactions will enable UAVs to carry out limited combat missions that are not yet envisioned.
-
With the continued development of software, miniaturized electronics (including information systems), specialized actuators and sensors, and innovative component design and manufacturing processes, UAVs could be produced at significantly lower cost than similar inhabited systems.
-
The continued development of human-machine interfaces, software, computer hardware, and miniature components will have a substantial effect on next-generation UAVs.
-
The feasibility of advanced UAVs and combat UAVs will require a highly capable and secure communications network.
MISSIONS
A wide range of potential missions—from surveillance through combat strikes—were described in the USAF SAB report (SAB, 1996). The objective of the SAB’s analysis was to identify technological needs in terms of threat environment, altitude, range, level of autonomy, and maneuverability. Twenty-two missions were identified to support five fundamental USAF capabilities: deterrence (conventional and nuclear), power projection, global mobility, situational awareness, and information domination. The missions and the time frames for operational demonstrations are shown in Figure 2-2. Of these missions, the SAB selected nine that would address USAF needs and requirements; would be operationally useful for joint military forces; would be technically feasible in a defined time
frame; and would be representative of the design, development, and enabling technologies for all 22 missions. The nine missions selected are listed below:
-
countering weapons of mass destruction
-
theater missile defense (ballistic missiles/cruise missiles)
-
attacking fixed targets
-
attacking moving targets
-
jamming enemy communications
-
SEAD
-
intelligence, surveillance, and reconnaissance (ISR)
-
communications and navigation
-
air-to-air combat
The SAB report identified three vehicle types the USAF would require in the near term to provide the size, configuration, observability, loiter altitude, endurance, and payload capacity and power to support the nine priority missions. The vehicle types were (1) penetrating HALE vehicles; (2) stand-off HALE vehicles; and (3) combat, medium-altitude, medium-endurance vehicles. The report recommended that the existing programs on HALE vehicles (Predator, Global Hawk, and Darkstar) be completed and that the USAF pursue the SEAD mission as the initial combat role for UAVs.
Finding. The USAFSAB has provided a comprehensive analysis of the USAF’s needs and potential missions for UAVs. This analysis of short-term and midterm needs was the basis for the committee’s assessment of the long-term technical and operational requirements.
VEHICLE ATTRIBUTES
The committee identified vehicle attributes to determine some of the technology trade-offs that will be required to develop a UAV system design. On the basis of vehicle attributes, design trade-offs, and technology trends, the committee was able to identify the technologies that would enable the development of potential UAV systems. Vehicle attributes in the general areas of configuration, performance, operation, and control (see Figure 2-3) ranged from conventional capabilities (i.e., capabilities that are commonly used or are readily available) to special capabilities (i.e., capabilities that will require research and development).
Configuration
Conventional, manned aircraft configurations are dominated by the need to accommodate human operators. If the considerations of pilot comfort and safety
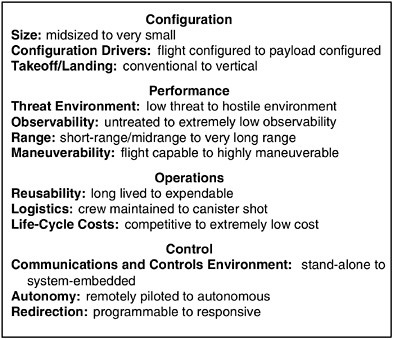
FIGURE 2-3 Range of vehicle attributes (from conventional missions to special applications).
are eliminated, the configuration of the vehicle can be determined by other considerations, such as mission requirements, operating environment, and size and configuration of major subsystems or payloads (e.g., aperture area required for sensors). The principal attributes the committee considered were vehicle size, configuration drivers, and mode of takeoff/landing. Vehicle size was assumed to range from conventional midsized to very small (e.g., micro air vehicles [MAVs] less than six inches in their largest dimension). Air vehicle configuration drivers ranged from conventional flight-configured vehicles, in which the operating regime or survivability determine configuration, to advanced payload-configured vehicles, in which factors such as aperture size and orientation and payload volume determine the configuration. Finally, although the more advanced vertical takeoff and landing systems were considered, the committee concluded that future USAF requirements could be met without advances in the mode of takeoff/landing.
Performance
Performance attributes include the capabilities required to perform the missions described earlier in this chapter. The principal attributes of UAVs considered
by the committee include threat environment, observability, range, and maneuverability. The threat environment was considered to range from low threat (for which no extraordinary measures are needed for survival) to hostile environments (for which potentially extreme measures are necessary to ensure survival). Depending on the threat environment, observability can range from conventional untreated vehicles to vehicles with extremely low observability, either as a result of design or surface treatments. Range involves two related factors—distance from station and endurance. Range capabilities were considered to vary from conventional short-range/midrange capabilities (i.e., the aircraft is not required to travel more than hundreds of miles from base or loiter for more than a few hours) to very long-range capabilities (i.e., where the vehicle is required to travel thousands of miles or loiter for as long as several days). Finally, maneuverability was considered to range from flight capable to advanced, highly maneuverable capabilities, free of pilot’s inability to withstand high accelerations.
Operations
The principal design trade-offs between mission capability and cost are most evident in the operational attributes. The operational attributes the committee considered included reusability, logistics, and life-cycle costs. Reusability, a reflection of the design trade-off between cost and durability or survivability, ranged from long-lived vehicles like conventional inhabited vehicles, (i.e., designed for indefinite life) to expendable, low-cost vehicles suited to high-threat environments. Vehicle logistics, which include transportability and maintainability, was considered to vary from crew-maintained vehicles (i.e., the conventional attribute for vehicles flown periodically and serviced by a maintenance crew between combat operations) to canister-shot vehicles (i.e., vehicles kept in storage until they are needed). Finally, life-cycle costs were considered to range from competitive costs, for vehicles with productivity and support requirements similar to those of inhabited aircraft, to extremely low costs, for which design and operational issues have been optimized for extremely low acquisition and support costs.
Control
Control attributes considered by the committee included the communications and control environment, the level of autonomy, and the ability to provide redirection. The communication and control environment ranges from stand-alone capability (i.e., individual vehicles operate independently) to system-embedded capability (i.e., individual vehicles operate in concert with other vehicles, both inhabited and uninhabited). Autonomy, the degree of self-reliance and independence the system is given, ranges from remotely piloted vehicles (i.e., the operator retains control throughout the mission) to autonomous vehicles (i.e., vehicles perform assigned missions without human intervention). Finally, redirection, an
attribute related to autonomy, varies from programmable vehicles (i.e., vehicles perform preprogrammed missions and have limited ability to be redirected) to responsive vehicles (i.e., vehicles that can be easily directed to change the mission during flight).
SYSTEM DESIGN
A number of system technologies, through their influence on air vehicle design, affect the basic vehicle subsystem technologies that are the focus of this report. The crosscutting technologies include communications and human-machine science, which are fundamental to the development of vehicle controls, as well as low-cost manufacturing. These technologies are discussed in the following sections.
Communications
The communications systems associated with a UAV can be divided into three categories: (1) external communications used to communicate commands to the UAV or extract data from the UAV; (2) internal communications to interconnect the payload, the flight and engine control systems, and other mission-management subsystems; and (3) relayed communications, in which a UAV communications payload is used to extend the horizon of ground-based communications systems or to relay command data to and from other UAVs. Communications technology is neither an enabling nor a limiting factor in UAV design, except in the case of MAVs, for which external and internal communications would be a challenge because of their small size.
The arrangement of internal communications to support the operation of a UAV is shown in Figure 2-4. A wideband (on the order of tens of megahertz) onboard bus interconnects all of the subsystems in the UAV. For current designs, the standard MIL-STD-1553B avionics bus would suffice. Many of the current avionics management systems used with the 1553 bus could be adapted for UAVs. Integrated weapons control systems have been configured to integrate communications, navigation, identification (CNI), and both internal and external sensor systems. These integrated systems are available for aircraft ranging from high-performance fighters to helicopters and can be preprogrammed for routine sorties or configured to be programmed during flight through external communications systems. Systems are available from U.S., Canadian, and European avionics suppliers (Johnson, 1998). The representative system shown in Figure 2-4 includes individual sensors, data conditioners, processors, and interfaces that do not exceed the bandwidths available in current hardware.
Development programs for future systems are under way, such as the USAF Pave Pace program for a totally integrated avionics architecture that will use a modular, digital approach to integrate CNI and sensor functions (Carmichael et
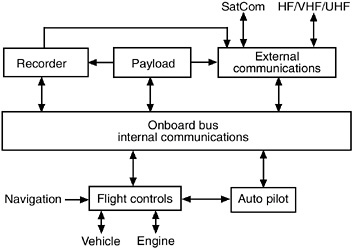
FIGURE 2-4 UAV internal communications system.
al., 1996). A high-speed optical network with crossbar switches operating at 1 to 2 Gbytes per second will interconnect the sensors, processors, and CNI functions and distribute the data using a fault tolerant approach. Similar processing will integrate the radio-frequency system of synthesizers, receivers, transmitters, and antennas. Millimeter and microwave integrated circuits will be used along with ceramic packaging. Multi-arm, spiral, coplaner antennas will span the frequency spectrum from about 200 Mhz to 6 Ghz. There will also be broadband active arrays for radar and electronic warfare functions.
The Pave Pace program started in 1994, and laboratory demonstrations were conducted in 1998 and 1999. The development is being managed by the Air Force Research Laboratory, and virtually all major airframe companies and electronics/avionics houses are participating in the program. The availability of this integrated avionics architecture would substantially reduce the number of external sensors, as well as the size and weight of the data processing and distribution system. These reductions in payload and control-system weight and volume would enable improvements in mission performance and/or vehicle range.
Figure 2-5 shows external communications for several potential military missions in which UAVs would act independently. The data-gathering, processing, and relaying functions could be accommodated by communications systems currently available. The deployment of many of these vehicles simultaneously, either as combat or surveillance units, might appear to create an excessive bandwidth requirement. However, data compression techniques will be available to reduce the required bandwidth by as much as a factor of 10. The housekeeping data from each vehicle will be minimal and can be handled on a narrowband
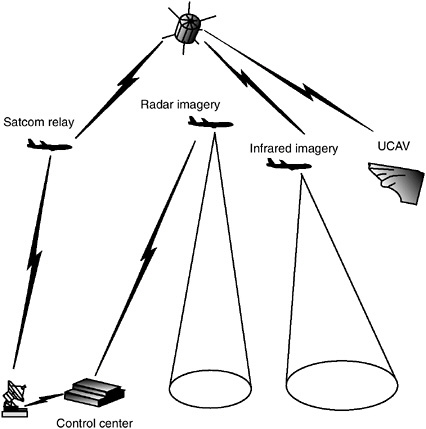
FIGURE 2-5 Notional UAV external communications system.
channel. Time division multiple access (TDMA) techniques can easily accommodate the multiple data streams. For vehicle control from the ground or reprogramming of payloads, telecommunications bandwidths of about 50 kHz should suffice. The approach used by the Internet’s Worldwide Web has demonstrated the simultaneous service of many users at telecommunications bandwidths, albeit in an unstressed, benign environment.
In a wartime environment, the MILSTAR satellite could be used for control. Although the operating bandwidth is narrow (2.4 Kbits per sec), superb antijamming protection is provided. The number of channels available would be determined on a mission priority basis. The MILSTAR medium data rate channels could be used for relaying essential communications in a wartime environment or delivering volatile, high-priority surveillance data to the ground. MILSTAR would be a factor to be considered in the mission-planning phase of a wartime operation; the procedures for implementation would be an operational issue.
State-of-the-art throughput, storage capacity, processing speed, input/output bandwidth, and other basic parameters of signal processing and data processing has been doubling every two to three years (SAB, 1996). At that rate, within a few years, processors capable of 100 giga-operations per second (GOPS) will be available, along with gigabytes of memory. Current multimode signal processors require 5 to 10 GOPS, indicating that digital processing is not likely to be a limiting factor for UAV performance in the near term.
Finding. Communications and data processing are not limiting technologies for the development and operation of military UAVs. Available technologies can accommodate the needs of currently conceived missions, and developments under way in the telecommunications community will be able to satisfy the needs of expanded military missions for UAVs.
The DOD, through DARPA, has developed a program to apply advances in high-speed computation, signal processing, and miniaturization to mobile, wireless, multimedia information systems. This program, called Global Mobile Information Systems, recognizes that commercial advances will not meet all defense needs for security, interoperability, and other capabilities. A previous NRC study recommended ways for the military to “ride the wave of commercial technology advances while retaining technical capabilities that exceed those of any potential adversary” (NRC, 1997a). The study recommended component and systems development for modeling and simulation of military information networks, integrating commercial components into network architectures, upgrading network security, reducing co-site interference, fielding software radio technology, adapting smart antennas, developing transmission techniques that can adapt to a wide range of operating conditions, improving current filter technology for use in military software radios and high-density platforms, and enhancing the flexibility of software radios.
Human-Machine Science
The goal of human-machine science, also known as “human factors” or “human-system integration,” is to take advantage of human capabilities and compensate for human limitations in the design, manufacture, and operation of systems of all kinds. Human-machine science includes not only the primary system, but also all ancillary activities, such as logistics, operational procedures, maintainability, and training. The field is supported by, and draws on, several other disciplines, including psychology, physiology, medicine, engineering, sociology, anthropology, mathematics, and computer science.
Finding. The design decision that has the most profound effect on the human-machine sciences is degree of autonomy.
The degrees of autonomy for UAVs are listed below:
-
completely autonomous (once programmed)
-
quasi-independent (highly autonomous)
-
semi-autonomous
-
remotely piloted
The level of autonomy for some UAVs will vary depending on mission segment and/or unforseen events, such as system failures or enemy action.
A great deal of automation has already been implemented in current systems, including modern aircraft systems. Generally, decision making and override of automated systems have been retained for human operators, although UAV designs may change this. Experience with current operational and developmental systems (or concepts) has shown, however, that the integration of the human and machine components of the system is a much greater challenge than many anticipated (Munson, 1998).
Like most design alternatives, automation has both positive and negative aspects. Potential advantages of automation are greater operator safety, fewer human errors, more precise control, the capability to perform functions beyond the environmental or physical limitations of human operators, the capability to perform functions that humans do not want to do, and greater human comfort (Gabriel, 1992). The disadvantages of highly automated systems include boredom and a resulting loss of vigilance and situational awareness; interruptions or lags in communication links; more complex training because of the increase in operational modes, both normal and abnormal; higher costs for defining, coding, and checking automated functions; reduced ability to deal with unanticipated situations; and fewer cues available to the operator assigned to intervene. To remain effective, human operators must have meaningful tasks that are challenging but achievable and significant feedback on their performance.
A more comprehensive use of automation might increase, rather than diminish, the importance of human considerations in system design, development, and operation (Figure 2-6). Success will depend on the effective allocation of functions to humans and machines. For instance, humans will be able to intervene only if provisions have been made for intervention and only if the human is attentive.
Even though the methods and tasks involved in interacting with any system may vary significantly in terms of frequency and specific actions, the fundamental human functions to support future UAV operations will be similar to
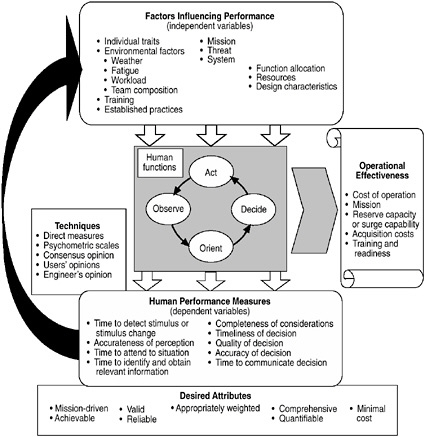
FIGURE 2-6 Human performance measures. Source: USAFSAB, 1998.
the ones carried out by humans today (i.e., observe, orient, decide, and act). Examples include:
-
logistics support, including planning, air vehicle breakout, mission programming, system checkout, systems management, service, and maintenance
-
execution of control and/or monitoring system performance, including mission redirection/reprogramming, direct control of systems or vehicle (e.g., sensors, weapons, and various other subsystems), communication with other operators, and coordination with other vehicles
The degree of independence of the automated system from human intervention is a vital design decision that will be influenced by many factors for a specific vehicle. The following operational considerations must be considered in determining the degree of automation:
-
status of related technology (i.e., can the functions be automated effectively)
-
mission type and importance (e.g., ISR, SEAD)
-
control of multiple UAVs
-
deconfliction requirements
-
environmental factors (e.g., visibility, terrain)
-
enemy activities, threats, and/or countermeasures
-
stores, including weapons, sensors, and communications
-
size of vehicle
-
range of vehicle
-
launch and recovery method
-
mission complexity
-
system reliability requirements
-
cost of total loss (e.g., system costs, availability of replacements, potential for mission failure, potential for collateral damage, potential for enemy capture of part, or all, of system)
-
user characteristics (e.g., aptitude, training, strength, fatigue)
-
cost of training
-
logistics requirements
-
duration of the mission
-
other friendly forces involved on ground near target or over flight path
-
number of other aircraft involved in the mission
Recommendation. The U.S. Air Force should continue to strengthen its activities on human-machine science related to the design and development of UAVs. Research should be pursued in the following key areas:
-
integration of human-machine systems into the design process, including (1) the optimal and dynamic allocation of functions and tasks and (2) determination of the effects of various levels of automation on situational awareness
-
human performance, including (1) the investigation of human decision-making processes, (2) the development of methods to define and apply human-performance measures in system design, and (3) the enhancement of force structure through improved methods of team interaction and training
-
information technologies, including (1) the determination of the effects of human factors on information requirements and presentation and (2) the development of enhanced display technologies to improve the human operator’s ability to make effective decisions
Low-Cost Manufacturing
Substantially reducing the total life-cycle costs of UAVs compared to the costs of conventional, inhabited air vehicles will be vital to the successful introduction and deployment of UAV technologies. Reducing the life-cycle costs to acceptable levels will necessarily entail reducing all system acquisition costs, including design costs, personnel costs, and manufacturing costs. This section focuses on low-cost manufacturing, specifically (1) designing for low-cost fabrication and (2) low-cost product realization. These considerations will influence the way that UAVs are designed and how technologies are prioritized.
Designing for Low-Cost Fabrication
Overall air-vehicle concepts, as well as specific subsystem concepts described in subsequent chapters, will have to be designed for low-cost fabrication. The following steps have the potential to reduce the overall cost of air-vehicle fabrication:
-
Reduce vehicle size and weight.
-
Reduce the part count for major assemblies.
-
Sacrifice weight (e.g., constant thickness, resin transfer molded composites instead of weight-optimized hand-layup constructions).
-
Maximize the use of room temperature processes.
Reductions in vehicle size and weight will generally result in reductions in fabrication costs and total life-cycle costs. Fabrication costs will be reduced simply because less raw and processed material will be required to produce components. Cost reductions through size and weight reduction will be realized as long as the manufactured cost per pound remains stable as size is reduced. Limits on the size of vehicle payloads (including weapons) and major subsystems (especially sensor apertures and propulsion systems) will determine the lower limit of vehicle size.
Modular vehicle designs could significantly reduce both design and manufacturing costs. One concept for a modular design would use a common centerbody module for a range of vehicle configurations (Lang, 1998). With a modular design, design and tooling costs could be amortized over multiple systems. Modular design could also be used for major subsystems by including common components (e.g., propulsion systems, avionics, communications systems, and sensors) for a range of air vehicles.
Low-Cost Product Realization
Substantial advances in commercial manufacturing have reduced the time and cost of getting product innovations to market. Although not all of these
advances are applicable, some of them could be adapted for defense applications (NRC, 1999). Rapid (and flexible) product realization requires low-volume production at a reasonable cost. Future UAVs could be developed and manufactured more rapidly and at lower cost if cycle times and nonrecurring costs can be significantly reduced through new approaches to product design, manufacturing processing, and the consideration of cost as an independent variable (CAIV) (NRC, 1999).
Practices that have been proven effective include integrated product and process development, the standardization of parts, and reduction in parts count. Three-dimensional digital product models, modeling and simulation of manufacturing processes, and virtual prototyping can also reduce cycle time and the need for late redesigns by predicting problems before resources have been committed for physical prototypes. Assembly modeling can complement simulations to optimize the assembly of complex systems.
Two new approaches to manufacturing processes could be explored. First, innovative processes that use low-cost tooling, including soft tooling (e.g., wood or composite tools for out-of-autoclave composite molding), flexible tooling that can be used for multiple parts or configurations, and toolless assembly could enable cost-effective, low-rate production. Second, generative numerical control (GNC), the automatic creation of process control data sets as the designer creates the three-dimensional product description, can be coupled on the factory floor with other knowledge bases to reduce flow times and can be configured to generate the manufacturing plan or process automatically from the three-dimensional data set.
CAIV is a means of treating cost as the principal input variable in the program structure, development, design, and support of a product. In past acquisition programs, the buyer and seller either accepted high costs as unavoidable or waited until late in the system development process to attempt to reduce manufacturing costs. In a general guidance document for implementing CAIV, the undersecretary of defense for acquisition and technology called for the early establishment of unit-cost goals based on performance-cost trade-offs. The document also stresses that strong incentives should be provided for program managers and contractors to implement CAIV objectives.
Development programs for UAVs must be structured from the outset to take full advantage of CAIV. Trade-offs of mission requirements and performance against cost and the establishment of unit-cost production goals should be done during the preliminary design and development phases. UAV designers, for example, may be willing to sacrifice “that last 100 miles of range” if it would drive up the unit cost by 20 percent. CAIV concepts can also be used for designing subsystems. For example, the avionics system, which accounts for 30 to 40 percent of the air vehicle cost, could (and should) be treated in a similar fashion to arrive at the best, low-cost solution. CAIV methods were successfully used to optimize product design and materials and process selection in the development of DARPA’s Miniature Air-Launched Decoy (MALD) (Price, 1998).