3
Alternative Technology Adoption and Development
Domestic and international organizations have increasingly recognized the safety and security risks and liabilities associated with possessing and using Category 1 and Category 2 radioactive sources, and some are voluntarily removing and replacing them with alternative technologies. However, challenges to voluntary adoption of these technologies remain, especially where there are no suitable alternative technologies or where alternatives would impose higher costs on organizations to provide comparable services. This chapter examines the economic and other considerations that affect decisions related to adoption of alternatives to radioactive sources as well as efforts to develop alternative technologies.
3.1 ALTERNATIVE TECHNOLOGIES TO RADIOACTIVE SOURCES
In this report, the primary alternative technologies considered are those that do not use a radionuclide as a source (nonradioisotopic alternatives). Radioisotopic alternatives, for example, those that use the same radionuclide but with a different chemical or physical form or use a different radionuclide that potentially poses less of a security risk, are not generally considered. The exception is use of vitrified cesium-137 as a possible alternative to cesium chloride sources as discussed in Sections 4.1.3 and 6.4.
In many cases, alternative technologies are already the standard of practice. For example, in high-income countries, external beam therapy using a linear accelerator (linac) is now standard practice for radiotherapy to treat cancer and other disease as opposed to teletherapy using cobalt-60 sources (see Section 4.3). For teletherapy, discussion of alternative technologies is most relevant to low- and middle-income countries (LMICs), many of which are transitioning to using linacs for radiotherapy.
3.1.1 Nonradioisotopic Alternative Technologies
In general, the most advanced and commercially viable alternative technologies for the applications discussed in this report are devices that use electricity to produce electron beams (e-beams) that directly irradiate objects or indirectly produce x-rays for irradiation by collision with a metal anode. For either purpose, the electron beams can be roughly categorized as low- or high-energy electrons. Low-energy electrons range from tens of keV (kiloelectron volts) to approximately 500 keV and are generated by a fixed potential difference between a cathode and an anode that determines the energy of the electrons. This category includes conventional x-ray tubes and electron
sources for surface treatment of materials. High-energy electrons are generated by both fixed potential machines and linacs. High-energy, fixed potential machines include Van de Graff generators and Dynamitrons. Their energies range from 1 to approximately 5 megaelectron volt (MeV). Although they also use a fixed potential, they are much larger than x-ray tubes. Linacs use electromagnetic energy, typically at microwave frequencies, in resonant cavities to accelerate electrons without having to sustain very large electrical potentials. Of relevance to radioactive source replacement, they are used to create electron beams ranging from 1 to greater than 20 MeV. Linacs are the electron source for the types of irradiation referred to in many applications in this report.
All electron sources start with a cathode that emits the electrons. In a linac, the cathode is part of an electron “gun” that emits electrons that are then accelerated through an electric field. To accelerate these electrons to the required energies, linacs use an accelerating waveguide—electromagnetic fields—to produce higher kinetic energies. To produce x-rays, these high-energy electrons strike a heavy metal target and are rapidly decelerated, generating “braking radiation” (bremsstrahlung) in the form of a broad and continuous energy spectrum of x-rays. In e-beam applications, magnets steer these high-energy electrons toward the target. A schematic representation of the basics of gamma, e-beam, and x-ray radiation sources is shown in Figure 3.1.
To produce neutrons, deuterium (D or 2H) ions rather than electrons are accelerated. These ions are accelerated in a high-speed beam using a linac and directed into a target containing deuterium or tritium (T or 3H) atoms to generate neutrons. D-T collisions are preferred because their neutron yield is 50 to 100 times higher than that from D-D collisions; however, tritium is a radioactive hydrogen isotope with a half-life of 12.5 years, while deuterium is a stable isotope of hydrogen.
The energy distributions for radioisotope, x-ray, and e-beam sources are fundamentally different. Radioisotope decay typically produces sharply peaked energy spectra, with primary gamma rays at just one or a few energies. For example, the primary gamma rays of cobalt-60 are at 1.173 MeV and 1.333 MeV and that of cesium-137 is at 662 keV. In contrast, x-rays produce a broad spectrum that includes x-rays of all energies below the maximum energy and an average energy of about one-third the maximum energy. Sidebar 3.1 discusses three quantities—power, energy, and dose—that are relevant to discussions of equivalency across the three radiation sources.
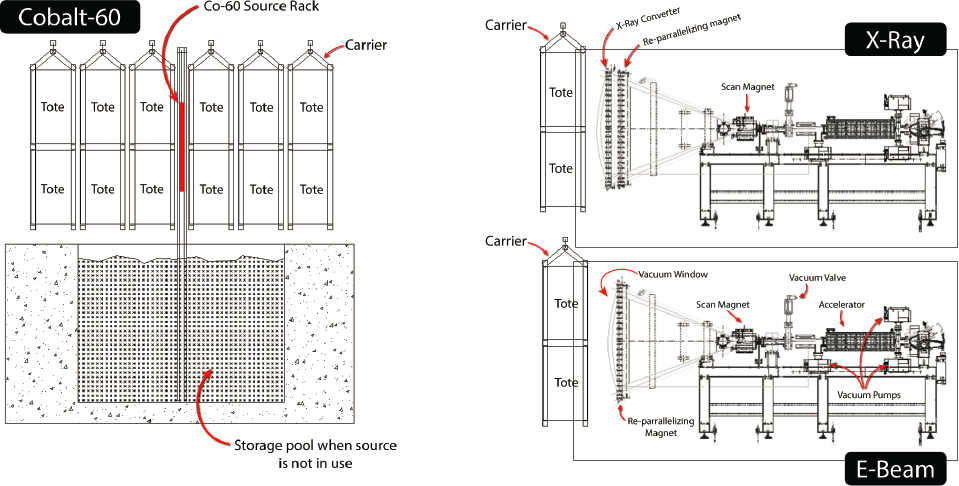
SOURCE: Reproduced and modified from Miller, 2003, with the permission of AIP Publishing.
3.1.2 General Characteristics of Alternative Technologies
The primary considerations with alternative technologies are typically technical performance and cost. Assuming that technical performance in a specific application is comparable, then the pros and cons of a radioactive
source and the alternative technology are considered, including factors such as capital costs (including purchase price and facility adaptation), operating costs (including training and maintenance), throughput, regulatory requirements and accreditations, safety and security risks and liabilities, and disposal options (see Appendix E). Typically, alternative technologies, when compared to radioactive sources, involve
- Higher initial purchase costs;
- More highly trained personnel to operate them;
- More frequent failures and repairs that require expensive service agreements;
- Lower regulatory requirements and licensing activities associated with secure management and transportation;
- Likely higher safety requirements related to high-voltage, high-vacuum, compressed gases used in these technologies;
- Higher costs associated with expansion of production facilities;
- More dependence on electric and water grid stability;
- Significantly lower decommissioning costs and better-defined guidelines and disposal pathways; and
- Lower liability risks for third-party damages related to misuse.
3.2 INSTITUTIONAL CONSIDERATIONS FOR ADOPTING ALTERNATIVE TECHNOLOGIES
Effective technology adoption requires both engineered devices and organizations that facilitate their efficient adoption and use. In high-income countries, institutional environments typically support both technology development and technology adoption. Therefore, the assessment of replacement technologies in high-income countries can largely take the institutional environment as given and focus on the extent to which the technologies can provide comparable services and their incremental costs, a topic addressed in the next section.
In LMICs, institutional environments often do not support adoption of alternative technologies. Governments and other domestic organizations may not have adequate resources to support the personnel required to implement, maintain, and effectively use new technologies in the delivery of public services such as health care. Consequently, the assessment of replacement technology in LMICs must necessarily consider the institutional environment in which technologies are intended to operate as well as the capabilities and costs of the technologies.
The feasibility of linacs as alternatives to cobalt-60 teletherapy machines is discussed in some detail in Section 4.3.3 through several case studies that illustrate the challenges with adopting alternative technology in LMICs. In the United States, the almost complete phasing out of cobalt-60 devices in teletherapy depended primarily on the superior technical capabilities of linacs. In LMICs, however, assessing the feasibility of adopting the technology requires explicit consideration of the institutional resources to support it. Several institutional and general infrastructure weaknesses often hinder, or even prevent, effective use of linacs: coordinating the timing of construction and delivery of devices may be difficult because of understaffed customs offices; the supply of electricity may not be reliable; physicians may not have training for effective use of the devices; and personnel trained to repair the devices may not be available in-country. An institution that considers replacement of cobalt-60 teletherapy machines may not have the resources to overcome these weaknesses to make the linacs viable alternatives to the cobalt-60 teletherapy machines. Several organizations, including the International Atomic Energy Agency, the OPEC Fund for International Development, and the U.S. Agency for International Development, have assisted in the adoption of alternative technologies in LMICs by providing training, assisting with contractual arrangements for the linacs, or sharing costs of adopting the technologies. However, several challenges remain that negatively affect delivery of health services.
3.3 ECONOMICS OF ADOPTING ALTERNATIVE TECHNOLOGIES
Decisions about whether and when private organizations should adopt alternative technologies to replace radioactive sources are complex. To understand these complexities, the committee reviewed the basic economics of the replacement decisions (see Appendix E). In its review, the committee makes two claims that suggest the importance of public policy in promoting the adoption of replacement technologies.
- Institutional factors affect the economic feasibility of replacement technologies as well as potentially their desirability from the social perspective. Within the United States and other high-income countries, regulations affect the relative costs and benefits of alternative technologies. In LMICs, infrastructure and human capital constraints weigh more in the decisions.
- Externalities in radioactive source use may result in private organizations not adopting commercially available replacement technologies that would be socially beneficial, for example, because they reduce the risks of a malevolent act such as use of a radiological dispersal device (RDD).
The viability of any potential replacement alternative technology depends on the institutional context of its use as well as its physical characteristics. Public policies can change the institutional contexts. For example, domestic regulations may reduce the external costs of radioactive source use by making malevolent use less likely. International efforts to adapt replacement technologies to contexts in developing countries may make them more viable. Because private organizations do not bear the full costs of radioactive source use, they may not adopt socially beneficial replacement technologies even when they are commercially available. Public policies that internalize more of the currently external costs of radioactive sources, such as requiring larger contributions toward covering the costs of disposal, make replacement technologies relatively more attractive.
Private entities can be expected to choose technologies that maximize the difference between anticipated benefits and costs, taking account of perceived risks. If these benefits and costs are inclusive, in the sense that they include all the costs and benefits borne by others in society, then this private calculus also maximizes the social value of the choice in the sense of allocating resources to their most valued uses. However, if the benefits and costs to the private entities that consider adoption of a technology are not inclusive, then a choice that is beneficial for the private entity may not be beneficial for the broader society. The benefits and costs of choices not borne by the private entity making the choice are called externalities. Use of radioactive sources typically involves external costs due to the risk of accident or a malevolent act that involves the radioactive source and source disposal.
As discussed in Appendix E, the economics of replacement of radioactive sources already in service may differ substantially from de novo decisions comparing their life-cycle costs with those of alternatives. The typically high up-front costs of radioactive sources are “sunk” when considering replacement of these in-service devices with long remaining useful lives. Consequently, alternative technologies that are economical in de novo decisions may not be economical from the perspective of the user of a device already in service. Most devices that contain radioactive sources are based on mature technologies that have been in service for many years and in general have proven to be reliable. This maturity contributes to current cost advantages. However, it also suggests that costs are unlikely to further decrease. Because radioisotopes are sometimes scarce, as seems to be the case currently with cobalt-60, it is possible that the costs of devices that contain radioactive sources may increase. At the same time, many alternative technologies that are currently commercially available but not yet mature are likely to become more cost-effective as they gain larger market shares and as research and development (R&D) efforts focus on making them simpler and more cost-effective. Additionally, some replacement technologies may provide higher-quality services as they mature, as is the case with linacs compared to cobalt-60 teletherapy. These trends will likely make alternative technologies increasingly more attractive.
3.4 PROMOTERS OF ALTERNATIVE TECNOLOGIES
Regulators can play a role in encouraging users to consider alternative technologies because enhanced regulation can act as a disincentive for the continued use of radioactive sources. Disincentives could include increased regulatory requirements for the security of sources, implementation of financial guarantees for end-of-life management (see Section 2.8.4), and requirement for licensees to justify the need to use a high-activity radioactive source before being authorized to do so. Disincentive policies have market and societal implications and these implications warrant careful examination before the policies are implemented.
The U.S. Nuclear Regulatory Commission (U.S. NRC) does not promote or encourage use of alternative technologies to reduce security risks related to radioactive sources. If an applicant for a U.S. NRC radioactive materials license can demonstrate safe and secure use of materials, the application will be approved, even if there
is a nonradioisotopic alternative available. The U.S. NRC conducts a pre-licensing inspection of an applicant’s facility to ensure that the applicant has implemented the applicable security requirements prior to taking possession of Category 1 or Category 2 radioactive materials. Several organizations including the Nuclear Threat Initiative (NTI) (Iliopulos et al., 2019) and the Government Accountability Office (GAO) (2019) have been critical of the U.S. NRC’s lack of a proactive role in supporting regulatory changes to constrain use of radioactive sources or to promote broader use of alternative technologies.
Across Agreement States, some regulators encourage proactive, preventive policies that provide regulatory disincentives for holding radioactive source licenses, whereas others follow the minimum federal requirement and play the role of code enforcers. For example, the California Department of Public Health Radiological Health Branch encouraged the use of alternative technology by providing information on cesium-137 licensing requirements and x-ray machine registration requirements to make the information readily available in the adoption process and more comprehensive. If a new cesium-137 irradiator license application is received for regulatory approval or renewal, the state regulator informs the licensee about available alternative technologies and requires a justification for use of cesium-137 (Iliopulos and Boyd, 2020). In contrast to the U.S. NRC, which does not regulate radiation-emitting devices, Agreement States regulate both radioactive sources and radiation-emitting devices.
Several organizations have facilitated adoption of alternative technologies, primarily by creating networks of stakeholders to raise awareness on risks and liabilities related to radioactive sources; by facilitating dialogues about performance data, costs, and the challenges of adopting alternatives; and by providing tools to support more-informed decisions. As a result of these efforts, some hospitals, research centers, and governments are increasingly recognizing the risks associated with radioactive sources, and some are voluntarily removing and replacing them when viable options are available. However, most of these organizations identify themselves as “technology neutral” and do not promote alternative technologies to radioactive sources as part of their mission. Also, no organization is currently a “one-stop shop” that provides easy access to complete information relevant to the adoption of alternative technologies in the different applications.
The International Irradiation Association (IIA) and the World Institute for Nuclear Security (WINS) are two examples of organizations that have addressed technical or procedural aspects related to adoption of alternative technologies (see brief descriptions of their work in Section 1.5) without being promoters of specific technologies. For example, the IIA has noted that it supports the safe and beneficial use of all forms of irradiation including gamma irradiation (IIA, 2018) and opposed draft legislation Section 402 of the fiscal year 2015 Senate Energy and Water Development Appropriations Bill that would have required the “phasing out” of radioactive sources in medicine over a period of years (IIA, 2014). In addition, the expertise, and audiences, of the IIA and WINS are primarily involved in irradiation technologies and nuclear or radiological security issues, respectively, and do not address nonradiation modalities that may be alternatives to radioactive sources, for example, genetic methods to replace the sterile insect technique. NTI is another organization with valuable contributions in the adoption of alternative technologies but, as with WINS, its primary focus is risk reduction.
In 2016, several U.S. agencies including the U.S. NRC, the Department of Energy, the Department of Homeland Security, and the Environmental Protection Agency, formed the Interagency Working Group on Alternatives to High-Activity Radioactive Sources (GARS). The group’s scope was to provide an assessment on how federal agencies are engaged in activities related to high-activity radioactive sources and their alternatives; engage relevant federal agencies in the development of ideas regarding their potential transition to alternative technologies; support the process to further the R&D of alternative technologies; and develop a best practices guide for federal agencies to adopt a long-term transition to alternative technologies. GARS members provided a set of recommendations to federal agencies on best practices for the successful transition of high-risk radioactive sources to alternative technologies and how this can be incorporated in each agency’s strategic plan (NSTC, 2016). The recommendations covered four categories of possible federal action: (1) federal procurement and grant making; (2) agency priorities; (3) education and outreach; and (4) R&D. In addition, the working group made several recommendations to federal agencies, including that they should:
- Promote adoption of alternative technologies in federally funded programs and facilities by encouraging use of voluntary incentives, dedicated funding, and facilitated conversion.
- Involve all key stakeholders in adoption of alternative technologies in the transition and recognize the role of manufacturers and distributors.
- Consider the full life-cycle costs of high-activity sources, including costs of security, disposition, and potential liability.
- Balance the respective operational and technical needs of the user.
The National Nuclear Security Administration’s (NNSA’s) Office of Radiological Security (ORS) has been promoting the adoption and development of nonradioisotopic alternative technologies as part of its mission. The NNSA representative who briefed the committee1 noted that this task became part of the agency’s mission following the 2008 National Academies report recommendation to the U.S. government to adopt policies that provide incentives to facilitate the introduction of replacement technologies. The representative also noted that further support for incorporating this task in the agency’s mission was provided by the Task Force on Radiation Source Protection and Security reports to Congress that recommended that the U.S. government incentivize alternatives and lead by example (U.S. NRC, 2010, 2014c, 2018).
The activities that ORS leads domestically and internationally to achieve the alternative technology mission are described in Sections 2.4.2 and 3.6. Despite significant progress, one U.S. government agency cannot work in this area alone to address the issue in a global context. Greater impact in promotion of the adoption and development of nonradioisotopic alternative technologies globally could be enabled by the increased participation and cooperation of additional U.S. government agencies, non-U.S. governments, international organizations, and other stakeholders. Some have recommended that the International Atomic Energy Agency (IAEA) establish a program focusing on alternative technologies with a defined scope, mission, and timeline (Roughan, 2018). The committee agrees that the IAEA has the technical capabilities to create and manage a successful program. In addition, it has access to contacts and data from Member States and could use these resources to enhance capacity to acquire, operate, and maintain alternative technologies. However, it is not clear to the committee whether promoting alternative technologies is currently within the IAEA’s mission. Although some IAEA activities clearly encourage the adoption of alternative technologies, the agency may still provide high-activity sources for medical or industrial applications through its Technical Cooperation program to its Member States requesting such support, as long as they meet the required safety standards. In addition, the IAEA engages in activities at the request of its Member States, so unless Member States request that the IAEA take on specific actions regarding alternative technologies, it is unlikely that such a program would be a priority for the agency.
There is a need for an organization or a network of organizations to take the leading role of stimulating the promotion and development of alternative technologies to radioactive sources. This entity could help enhance access to information by establishing national and international information centers (one-stop shops), make that information reachable and widely publicized, and provide easy access to complete information. Such an organization could help make significant progress in promoting adoption of alternatives.
3.5 PROGRESS IN ADOPTING ALTERNATIVE TECHNOLOGIES
WINS identified a set of questions that organizations would need to consider when assessing adoption of alternative technologies (WINS, 2018a). These included:
- What are the organizational needs?
- Which replacement options would serve the needs best?
- Will the new technology provide comparable results?
- Will it be necessary to redesign an existing facility and retrain staff?
- What about reliability and service?
- What are the costs?
- What are the safety and radiation protection implications of the change?
___________________
1 Lance Garrison, NNSA, presentation to the committee on February 26, 2021.
- What are the regulatory implications of the change?
- What is the level of exposure to potential liabilities?
The answers to these questions vary by application as well as by adopting organization. In Chapters 4 to 6, the committee examines the alternative technology options and progress with adopting these alternative technologies in medical and research (Chapter 4), sterilization (Chapter 5), and other industrial applications (Chapter 6). Some of the main points discussed in these chapters are summarized in Table 3.1.
3.6 ALTERNATIVE TECHNOLOGY DEVELOPMENT
Several large companies are investing in R&D to improve an existing product or develop a new product that provides a specific commercial solution. Large companies, for example, those that manufacture medical devices, often have significant budgets for R&D and often have in-house R&D departments that conduct ongoing R&D projects to further their business goals. In Chapter 4, the committee discusses two efforts by manufacturers of external beam therapy systems to produce linacs that can reliably operate in challenging environments such as in LMICs where there are frequent interruptions of electric supply. Details of ongoing projects by these larger companies are often not revealed until they are in later stages of development. The transition of a creative idea to a commercial product, if successful, can take years (often more than a decade) and requires substantial investments.
NNSA, like other federal agencies, allocates some of its annual extramural R&D budget (about 3 percent) to fund small businesses through the Small Business Innovation Research (SBIR) program. ORS and NNSA’s Office of Defense Nuclear Nonproliferation R&D fund about 30 SBIR and Small Business Technology Transfer (STTR) projects, and about one-third of those concern alternative technologies to radioactive sources. Other SBIR-funded topics include radiation detection, space-based sensors, and remote detection. The NNSA offices designate R&D topics in their solicitations, and awards are made on a competitive basis after proposal review and evaluation jointly by ORS and NNSA’s Office of Defense Nuclear Nonproliferation R&D.
The SBIR Program is structured in three phases2:
- The objective of Phase I is to establish the technical merit, feasibility, and commercial potential of the proposed research or R&D efforts and to assess the performance of the small business awardee organization prior to advancement to Phase II. SBIR and STTR Phase I awards are generally $50,000 to $250,000 for 6 months (SBIR) or 1 year (STTR). Phase I awards are managed by the NNSA’s Office of Defense Nuclear Nonproliferation R&D.
- The objective of Phase II is to continue the research or R&D efforts initiated in Phase I. Typically, only Phase I awardees are eligible for a Phase II award and about 50 percent of Phase I awardees progress to Phase II. SBIR and STTR Phase II awards are generally $750,000 for 2 years. Phase II awards are also managed by the NNSA Office of Defense Nuclear Nonproliferation R&D.
- The objective of Phase III is for the small business to pursue commercialization objectives resulting from the previous phases’ activities. Phase III awards for alternative technology projects are typically managed by NNSA ORS.
As of December 2020, NNSA funded four Phase I, six Phase II, and two Phase III projects on alternative technologies to radioactive sources (see Table 3.2). Some of the SBIR and STTR funding on alternative technologies was directed toward projects that provide potential improvements to existing solutions, for example, the development of flat-panel x-ray sources for use in blood irradiation and research applications. Other funding was directed toward projects that aim to fill a gap and develop novel innovative solutions to problems that currently do not have a solution, for example, to build inexpensive, compact linacs for sterile insect technique and other applications.
The committee invited researchers and technology developers involved in these SBIR projects to present at its information-gathering meeting in December 2020 (see Appendix B for details), and it summarizes the status of
___________________
TABLE 3.1 Progress in Adopting Alternative Technologies in Different Applications
Application (chapter discussed) | Common Devices (primary isotopes) | Replacement Technology | Trend of Adoption of Alternative | Major Drivers for Adoption (other than security risks) | Primary Replacement Challenges | Promising Research and Development Focus Areas to Facilitate Adoption |
---|---|---|---|---|---|---|
Medical | ||||||
Blood irradiation (Chapter 4) | Self-shielded irradiators (cesium-137 and cobalt-60) | X-ray technology | Broad adoption nationally and internationally | CIRP in the United States and national government regulatory initiatives in other countries; cost savings throughout device life cycle; efficacy | User preference | Pathogen reduction methodologies for red blood cells |
Cancer treatment—external beam therapy (Chapter 4) | Teletherapy (cobalt-60) | Linac | Almost complete phaseout of radioactive sources in high-income and many middle-income countries; increasing adoption in LMICs | Versatility; superior treatment delivery; improved patient outcome; shorter treatments | None in high-income countries; economic, infrastructure; and resources in LMICs | Linacs that are affordable and resilient to interruptions to the electric supply |
Cancer treatment—stereotactic radiosurgery (Chapter 4) | Gamma-based radiosurgery including Gamma Knife® (cobalt-60) | Linac-based radiosurgery including CyberKnife® | Increasing adoption in high-income countries; low adoption of radiosurgery overall in LMICs | Treatment site versatility; lower setup costs | Presumed lower accuracy; user preference | Technologies aiming to reduce setup costs including for shielding |
Cancer treatment—HDR brachytherapy (Chapter 4) | HDR brachytherapy (iridium-192) | External beam therapy; electronic brachytherapy | Some adoption in high-income countries | Favorable reimbursement for external beam therapy | Electronic brachytherapy is not a viable alternative to the most common uses of HDR brachytherapy to treat gynecologic cancers | Electronic brachytherapy suitable for treatment of gynecologic cancers |
Research (Chapter 4) | Self-shielded irradiators (cesium-137 and cobalt-60) | X-ray technology | Increasing adoption | CIRP in the United States and national government regulatory initiatives in other countries; cost savings throughout device life cycle | Equivalency studies; legacy data; scarce resources in research institutions | Equivalency studies; development of x-ray devices with a mean energy of 600 keV and higher |
Application (chapter discussed) | Common Devices (primary isotopes) | Replacement Technology | Trend of Adoption of Alternative | Major Drivers for Adoption (other than security risks) | Primary Replacement Challenges | Promising Research and Development Focus Areas to Facilitate Adoption |
---|---|---|---|---|---|---|
Sterilization | ||||||
Medical device sterilization (Chapter 5) | Panoramic irradiators (cobalt-60) | E-beam and x-ray | Increasing adoption | Market needs due to growing demand; scarcity of cobalt-60 availability; safety concerns and possible stricter regulation of EtO fumigation | Equivalency and revalidation | Development of compact linacs to reduce capital costs; development of economical x-ray sources |
Food safety treatments (Chapter 5) | Panoramic or other high- and low-activity irradiators (cobalt-60) | E-beam and x-ray | Stagnant in the United States; declining in Europe; increasing adoption in certain parts of the world, especially in China | Market needs | Public acceptance; lack of harmonization of regulations in international trade; outsourcing of treatments; labeling requirements | Development to reduce capital costs; more development of economical x-ray sources |
Phytosanitary treatments (Chapter 5) | Panoramic or other high- and low-activity irradiators (cobalt-60) | E-beam and x-ray | Increasing | Market needs; simplicity of treatment | Economics; pressures to reduce use of methyl bromide fumigation | Development to reduce capital costs; more development of economical x-ray sources |
Insect sterilization (Chapter 5) | Panoramic or other high-activity irradiators (cobalt-60); self-shielded irradiators (cesium-137 or cobalt-60) | E-beam, x-ray, and genetic modification | Increasing | Availability and transport of self-shielded irradiators; increasing demand for application especially for regional mosquito control; negative public perception toward genetic modification of insects | Unfavorable first experience due to unreliability of early (first-generation) x-ray devices | Development of x-ray sources to match use requirements |
Industrial Applications | ||||||
Industrial radiography (Chapter 6) | Radiography (cobalt-60, iridium-192, and selenium-75) | X-ray and ultrasonics | Increasing | Complementarity to radioactive sources | Not one-for-one replacement; technical and operational requirements in challenging environments; costs; higher level of technical qualifications; indirect as opposed to direct imaging | Image representation for ultrasonics; size, weight, and power improvements |
Industrial gauges (Chapter 6) | Cesium-137, cobalt-60 | Ultrasonics, differential pressure, radar and guided radar | Increasing | Complementarity to radioactive sources | Operational requirements in challenging environments, | Improve ruggedness of alternatives in challenging environments |
Well logging (Chapter 6) | Americium-241-mixed with beryllium | Neutron generators | Stagnant | None | Decline in market demand for the application; equivalency and reliability; legacy data | Equivalency studies; improvements in reliability of neutron generator |
Cesium-137 (ceramic or glass) | X-rays | None | None | Development of compact, rugged x-ray source; need for isotropic radiation | ||
Calibrators (Chapter 6) | Cesium-137 chloride | None | None | Possible policy to eliminate cesium-chloride from medical, research, and commercial application | Currently viewed as an application that needs to be exempt from replacement s efforts | Development and use of less dispersible form of cesium-137; mean x-ray at 600 keV and higher |
Radioisotope thermoelectric generators for space applications (Chapter 6) | Cobalt-60 | None | None | None | None | |
Plutonium-238 in pressed oxide form | None | None | None | Not recognized as a problem | None | |
Strontium-90 | None | None | None | None |
NOTE: CIRP = Cesium Irradiator Replacement Project; e-beam = electron beam; EtO = ethylene oxide; HDR = high dose rate; keV = kiloelectron volts; linac = linear accelerator; LMIC = low- and middle-income country.
TABLE 3.2 SBIR and STTR Projects Funded as of December 2020
SBIR or STTR Phase | Project Title | Small Business Awardee |
---|---|---|
Phase 1 |
|
|
Phase 2 |
|
|
|
|
|
|
|
|
Phase 3 |
|
|
NOTE: SBIR = Small Business Innovation Research; STTR = Small Business Technology Transfer.
some of these projects in Chapters 4 to 6 of the report. The committee did not seek presentations from other small businesses that are developing similar relevant technologies but are not supported by NNSA.
Development of these new technologies to potential practical applications would likely require both private and public funding. At different steps in the process, decisions are made by the funder about whether additional investment is warranted, and if so, how it can be secured. Continuation of development of the technology is often driven by the ability to tackle challenges related to the underlying physics and engineering. As most of these projects are at early stages of development it is not possible yet to fully assess how difficult it is to achieve these goals. However, technological challenges aside, a number of other factors influence the continuation of development of the technology including the investor’s faith in the ultimate commercial value of the technology, salesmanship of the developer, expected time frame for the product to reach maturity, patents and intellectual property claims, collaboration between initial developers with subsequent participants from the private sector, and the real or perceived promise of competing alternative technologies.
U.S. federal agencies often assess new technologies in terms of a nine-point Technology Readiness Level (TRL) scale: Basic technology research and proof of feasibility, TRL 1, 2, and 3; Technology development, TRL 4 and 5; Technology demonstration, TRL 6; System commissioning, TRL 7 and 8; Systems operation, TRL 9 (DOE, 2011). SBIRs are typically at early levels of maturation (TRL 2 to 5), and their progress to commercialization is uncertain because they are subject to the obstacles of bringing promising research ideas and discoveries to market. Significant investments will be needed to mature and potentially commercialize these technologies, a process that can take years or even decades. This timeline for developing alternative technologies is likely incompatible with the political desire to eliminate high-risk radioactive sources in much shorter timelines.
There is a crucial period between discovery and commercialization when critical funding may not be available to sustain the innovation process. This period, illustrated in Figure 3.2, is known as the “valley of death” (Islam, 2017; Klitsie et al., 2019; Nemet et al., 2018). While either public or private funding may support the early stages of development, commercialization is usually assumed to be the responsibility of the private sector. However, several uncertainties may deter private investors: development progresses through trial and error and may not lead to a viable commercial product; development may stretch over years or even decades, delaying the realization of returns on investment; and the extended development period may make it difficult to predict whether there will be a profitable market for the product. In general, these sources of uncertainty will be greater for products involving more novel and complex technologies. Furthermore, just as private adopters do not take
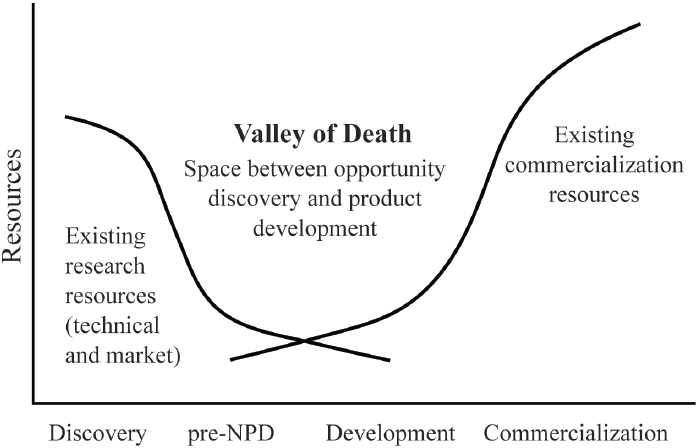
NOTE: NPD = new product development.
SOURCE: Klitsie et al., 2019.
account of externalities in their choices among commercially available technologies, investors cannot be expected to take account of externalities in their decisions about investing in the development of replacement technologies. Intermediary organizations, such as networks of potential users or government sponsors, may be necessary to help socially desirable technologies survive the valley of death (Islam, 2017).
3.7 CHAPTER 3 FINDINGS AND RECOMMENDATIONS
Finding 7: Many national and international government and nongovernmental organizations have contributed to the increasing visibility of alternative technologies as a way to reduce security risks from radioactive sources. However, no organization is currently equipped to promote the broad range of alternative technologies and address adoption issues in a global context. Such an organization or network of organizations could unite technical, regulatory, financial, policy, and country-specific resource information to influence decisions about adopting alternative technologies and facilitate the transition to alternative technologies for medical, research, and commercial applications, where appropriate.
Several organizations including NNSA, the IIA, WINS, NTI, and the IAEA have facilitated the adoption of alternative technologies, primarily by creating networks for stakeholders to raise awareness on risks and liabilities related to radioactive sources; by facilitating dialogues about performance data, costs, and challenges in adoption of alternatives; and by providing decision tools. In addition to these, NNSA also funds R&D and comparison studies. As a result of these efforts, hospitals, research centers, and governments increasingly are recognizing the risks associated with radioactive sources, and some are voluntarily removing and replacing them, if viable options are available.
There is a need for an organization or a network of organizations to take the leading role of stimulating the promotion and development of alternative technologies to radioactive sources. This organization or network of organizations could help enhance access to information by establishing national and international information centers (one-stop shops), make that information reachable and widely publicized, and provide easy access to complete information. Significant progress with adopting alternative technologies could be made if such an organization or network existed.
Finding 8: Progress with developing alternative technologies has been uneven across different applications and radionuclides (see Table 3.1). Except for blood irradiation, where x-ray technology is considered equivalent to cesium-137 irradiation, and external beam therapy, where linac technology is considered superior to cobalt-60 teletherapy, there are no broadly accepted replacement technologies for other applications. In some applications, no suitable replacement technology has been developed.
As described in Finding 12, despite technological advancements for medical applications, challenges exist in adopting alternative technologies in LMICs.
Table 3.1 summarizes the current status of alternative technology development for medical, research, and commercial applications. Progress in adoption of alternative technologies ranges from broad adoption as is the case with x-ray technology for blood irradiation and linac for external beam therapy, to increasing adoption as is the case with e-beam technology for medical device sterilization, to no adoption as is the case with alternative technology development for cesium-137 calibration sources.
Finding 9: Several large companies are investing in research and development to provide solutions to specific challenges associated with adoption of alternative technologies. The transition of a creative idea to a commercial product, if successful, can take years (often more than a decade) and requires substantial investments.
Large companies, for example, those that manufacture medical devices, can have significant budgets for R&D and often have in-house R&D departments that run ongoing projects to further their business goals. Details of developing projects by these larger companies are not often revealed until products are at later stages of development.
Finding 10: Several smaller companies have alternative technology development projects under way with financial support from the Small Business Innovation Research and Small Business Technology Transfer programs administered by the National Nuclear Security Administration.
ORS, in collaboration with NNSA’s Defense Nuclear Nonproliferation R&D, funds approximately 10 SBIR and STTR projects on developing alternative technologies to radioactive sources. These NNSA offices designate R&D topics in their solicitations, and awards are made on a competitive basis after proposal review and evaluation. Some of the SBIR and STTR funding is directed toward projects that provide potential improvements to existing solutions, for example, the development of flat-panel x-ray sources for use in blood irradiation and research applications. Other funding is directed toward projects that aim to fill in a gap and develop novel innovative solutions to problems that currently do not have a solution, for example, to build inexpensive, compact linacs for insect sterilization and other applications.
Recommendation E: The National Nuclear Security Administration should prioritize funding of projects that aim to develop alternatives to use of radioactive sources in applications where there are currently no acceptable nonradioisotopic alternative technologies.
Today, not all radioactive sources can be replaced by an alternative technology because such a replacement technology either does not exist or it has not demonstrated that it provides equivalent or improved performance compared to a radioactive source. NNSA has an opportunity to advance progress with identifying promising alternatives for these applications through the SBIR and STTR programs. The committee has identified three such applications—research irradiation, well logging, and calibration—and makes specific recommendations to NNSA and other federal partners to dedicate R&D and support equivalency studies for alternatives (see Recommendation F in Chapter 4 and Recommendations H and I in Chapter 6).