3
Indoor Sources of Indoor Particulate Matter
While the workshop’s first session focused on outdoor sources of indoor particulate matter, the second featured four presentations on indoor sources of indoor particulate matter. Marina Vance (University of Colorado Boulder) discussed the role of cooking in generating fine particulate matter (PM2.5). Michael Waring (Drexel University College of Engineering) described how secondary aerosols form PM2.5, and Linsey Marr (Virginia Tech) talked about the effect of humidity on the chemistry and biology of indoor air. In the session’s final presentation, Andrea Ferro (Clarkson University) explained how different sources of indoor PM2.5 influence the manner in which exposure and health effects are characterized. Kimberly Prather again moderated an open discussion after the presentations.
FINE PARTICULATE MATTER EMISSIONS FROM COOKING
Cooking is an important source of indoor PM2.5 and can vary dramatically from one home to another, said Marina Vance. As a result, the inhabitants of two adjacent homes could have very different exposures to PM2.5. She explained that there are two components to the air pollution generated by cooking: the heat source and the food. Primary emissions from cooking can include particles, VOCs, nitrogen oxides, and other pollutants.
Heat sources can include solid fuels such as coal, wood, charcoal, and crop residues; gaseous fuels such as propane, natural gas, liquefied petroleum gas, and biogas; electricity; and induction. Vance noted that some 2.8 billion people worldwide depend on solid fuels for cooking and those fuels
led to upwards of 4 million premature deaths in 2010, according to data from the Global Burden of Disease Study (Chafe et al., 2014; Smith et al., 2014). In the developed world, gas and electric heat sources are cleaner, so the emissions from food are the main source of indoor pollutants.
Cooking releases primary emissions that grow into a specific particle size and move through the indoor environment, where they can take up semivolatile organic compounds (SVOCs) from building materials and other sources, Vance explained. In addition, the volatile fraction of cooking emissions can undergo reactions indoors that may produce particles, and a large fraction of indoor particulate matter from cooking is likely to deposit on indoor surfaces. Some of these emissions may find their way outdoors, where they may undergo atmospheric processing, and the volatile components in particular could form secondary organic aerosols outdoors and impact ambient air quality as well.
Primary Emissions
The House Observations of Microbial and Environmental Chemistry (HOMEChem) study,1 for which Vance and Delphine Farmer were the principal investigators, aimed to identify the most important aspects of the chemistry that controls the indoor environment using the University of Texas at Austin’s test house, a 1200-square-foot manufactured home. One set of experiments observed particle emissions during various cooking activities (Figure 3-1) (Patel et al., 2020).
In one experiment that involved no cooking and opening and closing all the doors and windows in the house to allow maximum penetration of outdoor air pollution, indoor concentrations of coarse particulate matter (PM10) predominated at levels of 5–10 micrograms per cubic meter (μg/m3) (Figure 3-1, bottom panel). When intense cooking activities occurred with no ventilation—the so-called “Thanksgiving Day” experiment because it involved cooking a large meal meant to serve 12–16 people—there were intense peaks of all sizes of particulate matter, including PM2.5, that soared to hundreds of μg/m3 (Figure 3-1, top panel). Vance noted that the levels of ultrafine aerosols (PM0.1) rose significantly at some specific times of that day, demonstrating that it is not always true that PM0.1 accounts for very little of the particle mass in the indoor environment (Figure 3-2). In fact, at some times during this particular experiment PM0.1 accounted for most of the PM2.5, while at other times, PM0.5 accounted for the majority of the total particulate mass. This finding, she said, has implications for health, exposure, and the use of low-cost sensors as a means of evaluating air quality in the indoor environment.
___________________
1 Additional information is available at https://indoorchem.org/projects/HOMEChem/.
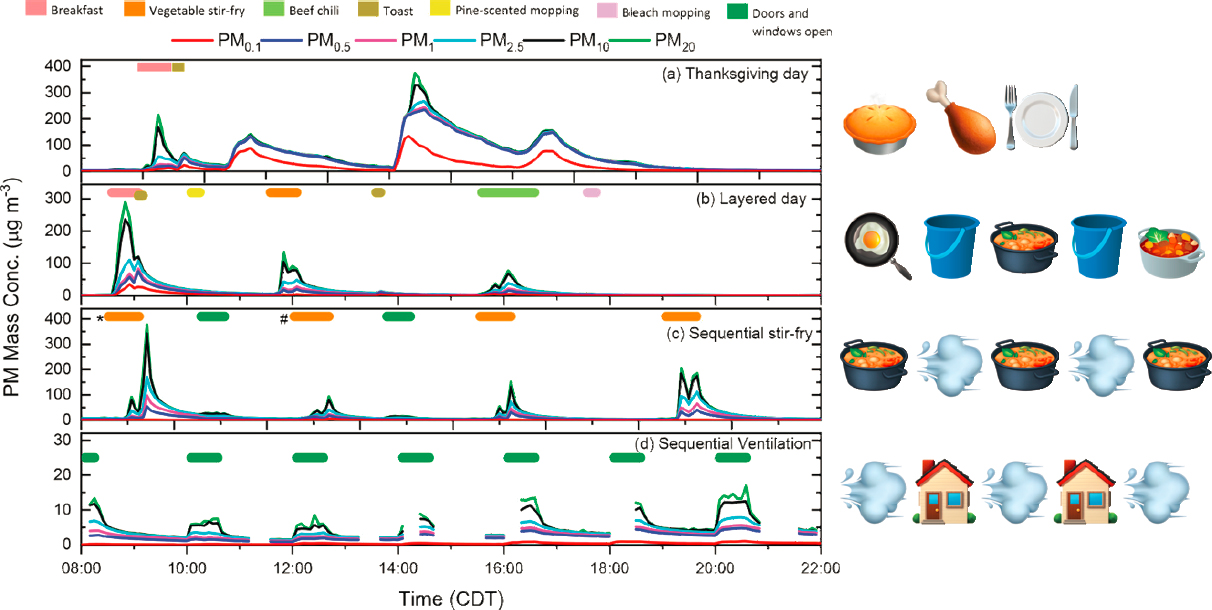
SOURCE: Vance slide 7, adapted from Patel et al. (2020) Figure 1.1
1https://pubs.acs.org/doi/10.1021/acs.est.0c00740; reprinted with permission from American Chemical Society Publications. Further permission related to the material excerpted should be directed to the American Chemical Society.
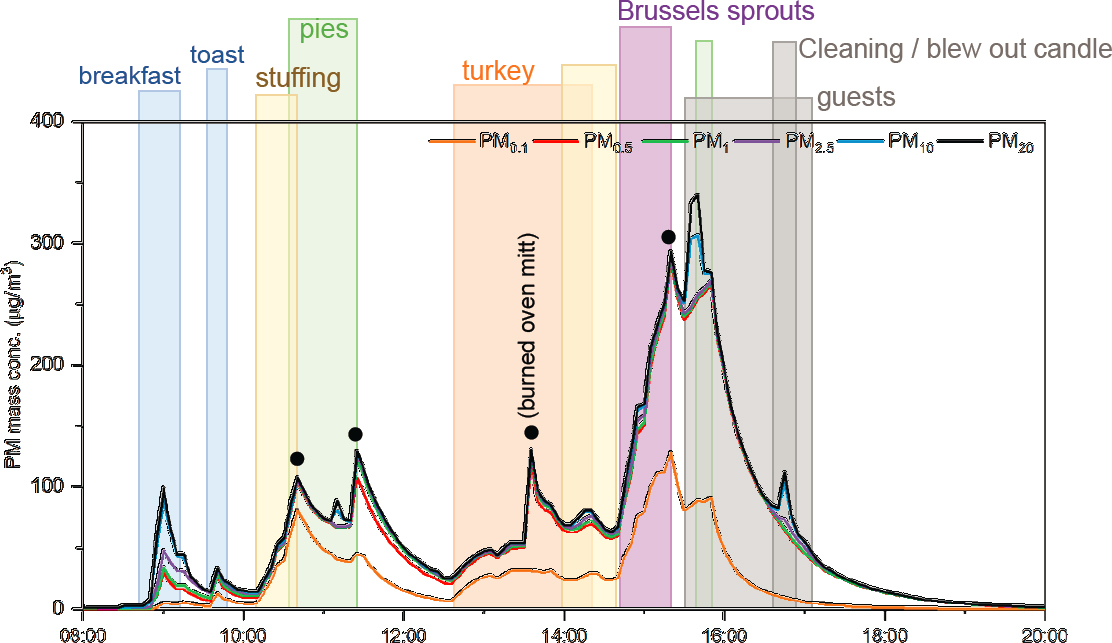
SOURCE: Vance slide 8, adapted from Patel et al. (2020) Figure S5.1
1https://pubs.acs.org/doi/10.1021/acs.est.0c00740; reprinted with permission from American Chemical Society Publications. Further permission related to the material excerpted should be directed to the American Chemical Society.
Scenarios designed to reproduce more typical cooking activities found that meals prepared on a propane stove produce approximately fourfold higher levels of particulate matter than meals cooked on an electric hot plate or in the oven or toaster. Another finding was that the heat source might be responsible for particle number levels, while the food may dominate mass concentrations.
In terms of composition, multiple studies have shown that indoor cooking produces substantial amounts of primary organic aerosol (Farmer et al., 2019; He et al., 2004; Katz et al., 2021; Klein et al., 2019), although there is a great deal of variability that could depend on what is being cooked, the cooking style, heat source, type of cooking oil, and other details (Farmer et al., 2019; Torkmahalleh et al., 2017). In particular, the concentration of polycyclic aromatic hydrocarbons associated with cooking emissions was highly variable, which can have serious implications with regard to health effects (He et al., 2004; Torkmahalleh et al., 2017).
Emitted particles can adsorb SVOCs released during cooking, but the HOMEChem experiments found that other substances, such as phthalates and adipates used in vinyl flooring and cyclic siloxanes from the oven and building materials, also ended up as part of the PM2.5 (Lunderberg et al., 2020). A similar study conducted in a house during normal occupancy found that the total concentration of SVOCs rose when there were indoor activities going on, particularly cooking (Kristensen et al., 2019) (Figure 3-3). In fact, 14 percent of all the volatile organic compounds present during cooking, and 25 percent when the oven was in use, were in the particle phase. Vance added that the SVOCs and particles emitted during cooking may undergo chemical reactions indoors. For example, the HOMEChem study found that SVOCs emitted during cooking could remain in the air and react later with components of a bleach solution used to clean the house.
Surface deposition can serve as an important sink for indoor particulate matter rich in organic matter. HOMEChem experiments found that although the Thanksgiving Day experiment produced higher airborne particulate matter concentrations than during a normal cooking event, the amount of particles deposited on a piece of glass (which was set up for testing purposes) and their size distribution were similar (Or et al., 2020). Another analysis of the HOMEChem data showed that the material accumulated on a glass surface had similar properties to the organic aerosol generated by cooking as measured by online aerosol mass spectrometry (O’Brien et al., 2021).
These results, said Vance, indicate that the particles detected in the air ended up on surfaces in the house—and in the human respiratory system. For a person spending the day outdoors during the HOMEChem test
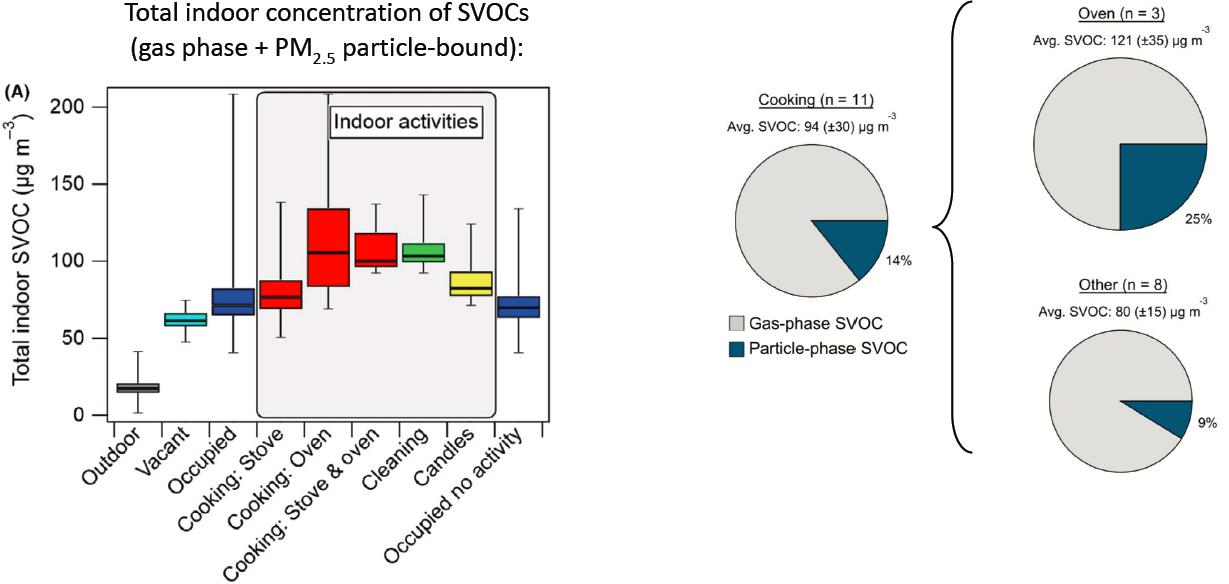
SOURCE: Vance slide 15, adapted from Kristensen et al. (2019) Figure 5A and Figure 7; reprinted with permission from John Wiley and Sons, Inc.
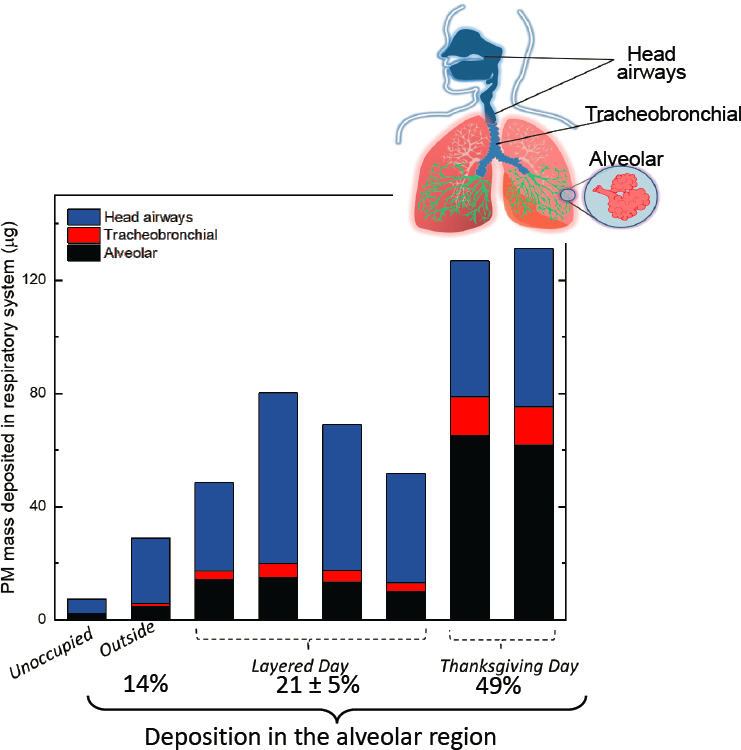
SOURCE: Vance slide 21, adapted from Patel et al. (2020) Figure 4.1
1https://pubs.acs.org/doi/10.1021/acs.est.0c00740; reprinted with permission from American Chemical Society Publications. Further permission related to the material excerpted should be directed to the American Chemical Society.
period, about 14 percent of the aerosol they inhaled would end up in the alveolar section of the respiratory system (Figure 3-4) (Patel et al., 2020). However, for someone participating in the Thanksgiving Day experiment “that would be about 50 percent, so half of the particles that they are inhaling would end up in the alveolar system,” she said. The reason for that increase, she noted, is because the average size distribution for a 24-hour period during HOMEChem was under 100 nanometers. She added that at
least one study has shown that the toxicity of inhaled particles can vary with the way food is cooked (Alves et al., 2021).
Despite the rich data generated by the HOMEChem study and others, there remain persistent knowledge gaps related to the particulate matter generated during indoor cooking. The literature indicates questions about commonalities or differences among cooking styles and ingredients, about how ventilation could affect the fate of particulate matter and exposure, and whether the chemical composition of cooking-generated particulate matter impacts its potential health effects. In terms of chemical composition and health effects, Vance remarked, questions remain about whether the bulk composition and size are important and what the influence of sorbed compounds is on health. More work is also needed to develop metrics for particle number versus mass and dose versus exposure time as they pertain to exposure and health effects. In addition, many questions remain about the contributions of indoor cooking activities to ambient air quality and the impact of socioeconomic disparities.
Vance concluded by noting that there is broad scientific consensus that for point sources of air pollution, it is critical to control emissions at their source. “For indoor cooking emissions in that context, what that really means is to electrify cooking, because cleaner fuels like the gaseous fuels are not necessarily clean fuels,” she said. For cooking, effective source control means operating a high-quality, externally vented over-the-stove hood every time cooking activities occur or using a well-positioned, properly selected portable air cleaner.
SECONDARY AEROSOL FORMATION OF FINE PARTICULATE MATTER IN THE INDOOR ENVIRONMENT
Organic aerosol, explained Michael Waring, comprises hundreds of thousands of types of molecules existing in a state of dynamic equilibrium between the gas and particle phases. Researchers classify organic aerosols based on whether they are primary aerosols produced by combustion or secondary aerosols that are the products of gas-phase reactions. Mass spectrometry is commonly used to categorize organic aerosols according to their emission type and character, with major categories including hydrocarbon-like, biomass burning, cooking, and both semivolatile oxygenated and low-volatility oxygenated (Sun et al., 2012; Zhang et al., 2011).
Most secondary organic aerosols formed indoors likely result from the reaction of ozone with an alkene such as terpene, which is emitted by building and consumer products (Kroll and Seinfeld, 2008). This reaction proceeds via a complex mechanism that generates many different products, including carbonyls, alcohols, carboxylic acids, peroxides, and organic
nitrates. These tend to be lower-volatility chemicals that partition to form secondary organic aerosols, Waring said. He noted that most studies on indoor secondary organic aerosol formation have been conducted in chambers or test rooms with intentional releases of reactants (Coleman et al., 2008; Destaillats et al., 2006; Sarwar et al., 2003; Waring, 2014; Weschler and Shields, 1999; Youssefi and Waring, 2012). Early studies relied on particle counters such as scanning mobility particle sizers; more recently aerosol mass spectrometers have yielded a major improvement in measurement capability and provided compositional information on aerosols that reveal chemical trends over time. Models of indoor secondary organic aerosols have also produced important insights.
Before discussing some of this modeling work, Waring provided an example of secondary organic aerosol formation in an experiment using an air freshener operated continuously at three air changes per hour in a chamber into which ozone was piped (Destaillats et al., 2006). Almost immediately, a strong burst of nucleation occurred in which new, small secondary organic aerosols formed. These grew into larger particles via gas-to-particle partitioning mechanisms before the size distribution stabilized.
Secondary Organic Aerosol Modeling
There are two approaches to modeling secondary organic aerosols: explicit production modeling, where products are tracked explicitly, assigned partitioning equations, and allowed to partition into an existing generic organic aerosol (Carslaw et al., 2012; Kruza et al., 2020); and lumped product modeling (Cummings et al., 2020; Cummings and Waring, 2019; Youssefi and Waring, 2012), which Waring uses. Lumped product modeling has particular strengths relative to explicit product modeling in that it can account for any arbitrary type of organic aerosol source, both indoor and outdoor; is not limited to secondary organic aerosols; and allows easy computation of bulk aerosol properties such as density or hygroscopicity.
After describing the parameters and equations his group uses in its models, Waring explained that this model can yield organic aerosol concentrations based on the volatility of organic mass and oxidation state. It shows that organic mass forms and ages via two processes: functionalization, in which a molecule gains oxygen-containing functional groups that enhance particle-phase concentration, and fragmentation, in which particle-phase molecules break into lighter fragments that can evaporate into the gas phase and reduce particle-phase concentration. He noted that organic mass can enter from outdoors along with ozone and the many volatile organic compounds that the model accounts for (terpenes plus other alkenes, alkanes, aromatics, and the like) or be emitted as primary
organic mass indoors along with the same VOCs and nitrous acid (HNO2 or HONO). Losses occur via deposition on surfaces or air exchange.
Waring and his collaborators have used the lumped product model to estimate the likely magnitude of secondary organic aerosol formation from VOC reactions with ozone or hydroxyl radicals in typical residences in the United States (Waring, 2014). These simulations predict that organic aerosols account for much of the indoor particulate matter and that primary organic aerosols, largely generated by cooking, dominate the organic aerosol content. While secondary organic aerosols are typically the smallest component of indoor organic aerosols, they can dominate both the aerosols and particulate matter when ozone and terpene levels are high and air exchange rates are low.
Research Needs
In the final portion of his presentation, Waring highlighted future research needs. The first concept that needs exploration is whether low-concentration, fast-reacting stealth reactants present in indoor air could have large effects on secondary organic aerosol formation. The origin of this idea was the surprising modeling result that, while d-limonene drives most secondary organic aerosol formation, the next most important driver was a-terpinene, and while the concentration of this compound is half that of d-limonene, it reacts ten times faster.
Another research need is to investigate whether the phase state of organic aerosol influences secondary organic aerosol partitioning and whether equilibrium assumptions about partitioning indoors are valid or secondary organic aerosol formation might be kinetically limited instead. The genesis of this question, said Waring, is modeling showing that most indoor organic aerosols are semisolid rather than liquid or amorphous solid, suggesting that it might be productive to use kinetic partitioning frameworks in models rather than equilibrium partitioning.
Waring then raised the question of whether nucleation or partitioning drives indoor secondary organic aerosol formation, as it is unclear which predominates based on modeling results. He also thought it important to know whether squalene present on human skin can react with ozone to form secondary organic aerosol particles, which a simple laboratory study suggested might be possible (Wang and Waring, 2014), and to identify effective mitigation strategies for indoor secondary organic aerosol formation. “I think we should be asking ourselves which terpenes are most important to remove from indoor formulations,” he said.
He also believes it would be useful to study whether standard practices are effective at removing secondary organic aerosols from indoor air, given
that they are dynamic mixtures. In other words, removing some of the organic aerosol content would just lead to the system readjusting.
The final research need that Waring identified was to better understand the influence of ozone and hydroxyl-producing technologies, such as ultraviolet lamps and bipolar ionization found in newer ventilation systems, on secondary organic aerosol formation.
THE EFFECT OF HUMIDITY ON THE CHEMISTRY AND BIOLOGY OF INDOOR AIR
Humidity is not a direct source of indoor particulate matter, but it does affect the chemistry and biology of airborne substances, said Linsey Marr. Absolute humidity (an expression of the concentration of water in the local atmosphere) can range from zero to 40 grams per cubic meter. Relative humidity is the amount of water vapor in the air compared to the maximum amount that the air can hold at a specific temperature. Absolute and relative humidity are strongly correlated indoors simply because the temperature varies within a narrow range (Marr et al., 2019).
Surprisingly, said Marr, there are few data on how indoor and outdoor humidity relate to one another, and studies that have examined the health effects of humidity have relied on outdoor data. One set of studies found that indoor and outdoor absolute humidity in temperate regions are correlated during the non–air conditioning season and that indoor relative humidity is better correlated with outdoor absolute humidity than outdoor relative humidity (Nguyen and Dockery, 2016; Nguyen et al., 2014). She noted that the pattern differs in tropical regions, where indoor absolute and relative humidity are linearly correlated with outdoor humidity in the absence of air conditioning, suggesting that outdoor absolute humidity may be a good proxy of indoor humidity in the absence of air conditioning (Pan et al., 2021).
Humidifiers can be a source of particulate matter indoors, noted Marr, particularly when using tap water, which contains dissolved minerals and even undissolved solids that can be aerosolized by nebulizing or ultrasonic humidifiers (Yao et al., 2019, 2020). Deionized water produces fairly low levels of particles, while water with dissolved solids produces a large number of particles. Water hardness does not seem to have much effect.
Humidity, Marr continued, has a large effect on the viability of bacteria and viruses in the air (Lin and Marr, 2020). Bacteria survive worst at low relative humidity, while virus survival is lowest at midrange levels of relative humidity, in both aerosols and stationary droplets. Relative humidity is important for chemistry, she explained, because it controls evaporation and condensation, and hence the size of airborne particles. For example, the
size of a particle containing physiologic levels of protein, salt, and influenza shrinks by more than half when relative humidity decreases from close to 100 percent to 20 percent, with most of the change taking place between near 100 to 80 percent (Marr et al., 2019; Mikhailov et al., 2004).
The weight of evaporating droplets depends on the rate of evaporation, which relative humidity controls. At low relative humidity, the weight of a droplet drops rapidly and solute2 concentrations can rise dramatically. At high relative humidity and slow evaporation, droplets lose far less of their weight and solute concentrations change only slightly (Lin and Marr, 2020). What Marr finds interesting is that at medium relative humidity, evaporation occurs more slowly and remains incomplete (Figure 3-5). She believes this occurs because the droplets form some sort of semisolid gel.
Mold, which thrives at higher humidity levels, is thought to be a problem in 18 to 50 percent of US buildings (Mendell et al., 2011), and concern about it accounted for some 90 percent of the calls and emails Marr received before the COVID-19 pandemic. Mold releases spores in the 2 to 100 micron size range, and they can act as allergens; exposure to mold is associated with upper respiratory tract symptoms, cough, wheeze, asthma, and other health problems (Fisk et al., 2007; Mendell et al., 2011), although Marr said there has been little research on the indoor emission rates of mold spores. Mold also releases microbial VOCs, and the chemistry and toxicity of these are also greatly underexplored, she added.
The pH of a droplet likely plays an important role in both the chemistry that takes place and microbial survival, but it is challenging to measure the pH of a 50 micron or smaller droplet. To address that problem, she and a colleague developed functionalized gold nanoparticles that they seed into a suspension that can then be aerosolized. The nanoparticles have a pH-sensitive dye that can be detected with surface-enhanced Raman spectroscopy (Freedman et al., 2019). In one experiment, Marr’s team found that the pH of droplets formed from water buffered at pH 7.3 was 10.5 ± 1.0, which would have a significant effect on the chemistry that could take place in those droplets. Further analysis found a spatial pH gradient in the droplets, with the highest pH values in the center of the droplet.
Marr noted that relative humidity and pH can have a significant effect on virus viability (Lin et al., 2020), demonstrating that relative humidity, which dynamically controls the water content of particulate matter and hence the concentration of the chemicals, bacteria, and viruses in those particles, affects both the chemistry and biology in aerosols.
___________________
2 A solute is a component of a solution, dissolved in a separate solution (the solvent).
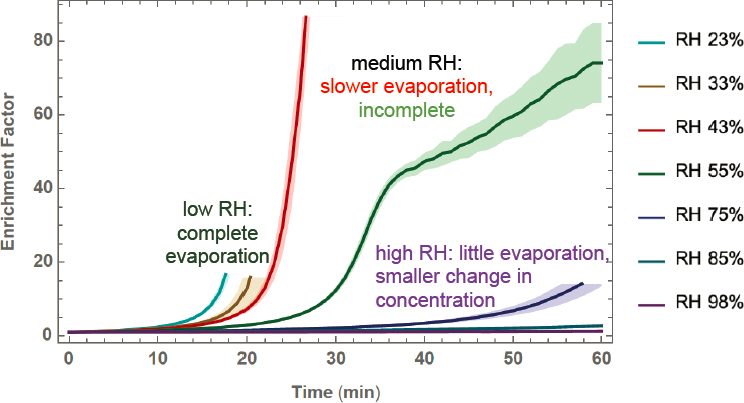
SOURCE: Marr slide 9, adapted and reprinted with permission from Lin and Marr (2020) Figure 3B; Copyright 2021 American Chemical Society Publications.
THE INFLUENCE OF SOURCES OF INDOOR FINE PARTICULATE MATTER ON THE CHARACTERIZATION OF EXPOSURE AND EVALUATION OF HEALTH EFFECTS
To start the day’s final presentation, Andrea Ferro highlighted a 1996 review paper on indoor particles that summarized studies in what was then a fairly new field (Wallace, 1996). At the time, the major known sources of indoor particulate matter were smoking, cooking, and “unexplained,” and the author noted that exposures in the “personal cloud” (an individual’s breathing zone) were higher than what was predicted from indoor monitoring. The paper also pointed out that personal PM2.5 exposures correlated poorly with outdoor PM2.5 levels, except when looking at individuals over long time periods.
Smoking and cooking are still considered the major sources of indoor particulate matter, and many of the “unexplained” sources are now known. Still, said Ferro, relevant research questions remain, such as the following:
- How does the indoor environment, including sources, occupants, building operation, and pollutant/microbial cycling, affect the level and composition of indoor PM2.5?
- How do acute and chronic exposure to indoor PM2.5 affect human health and how should health risks of indoor PM2.5 be assessed?
- What are the structural influences on disparate PM2.5 exposures across communities?
- What are the best approaches to mitigate indoor PM2.5 exposures?
Ferro pointed out that levels of indoor PM2.5 are often episodic, given the variety of sources; cooking, for example, is an episodic source, while a stove pilot light is a continuous source. And indoor and outdoor PM2.5 temporal patterns can be quite different. Indoor patterns change throughout the day and the week, reflecting the activities of the residents, while outdoor patterns are strongly associated with sources such as the level of vehicle traffic.
Given that short-term (<24-hour) increases in outdoor PM2.5 are associated with increased mortality (Lin et al., 2017; Lu et al., 2015) and that there is some evidence that short-term exposure to PM2.5 affects morbidity, Ferro wondered how indoor peaks in PM2.5 affect human health. Answering that question will require exposure estimates of indoor PM2.5 to assess acute health effects, such as myocardial infarction, that are known to be associated with peaks of PM2.5 concentrations, she said. In fact, indoor particulate matter may be more toxic than outdoor particulate matter. One study, for example, found that the inflammatory responses and allergic reactions triggered by PM10 collected at six schools in Munich were higher than for PM10 collected outdoors (Oeder et al., 2012). Studies using rodent macrophages found that indoor particles produced larger proinflammatory (Long et al., 2001) and cytotoxic effects (Happo et al., 2014) than outdoor particles.
The chemical content of indoor PM2.5 is enriched by bioorganic compounds such as squalene, cholesterol, and fatty acids, as well as compounds in chemical additives such as plasticizers, flame retardants, and surfactants. Each of these constituents, said Ferro, partitions between the gas and particle phases in a manner dependent on temperature and relative humidity, as well as chemical transformations including oxidation and acid-based and partitioning reactions. Indoor particulate matter also contains microbes that may originate from humans, plants, pets, plumbing systems, HVAC equipment, mold, resuspension of settled dust, and outdoor air (Prussin and Marr, 2015). Ferro mentioned a 2017 National Academies report that summarized research on the indoor microbiome and the built environment (NASEM, 2017), and noted that information on virus transmission through aerosols has increased substantially as a result of the COVID-19 pandemic.
In terms of source apportionment, techniques such as positive matrix factorization can determine the sources for human exposure, including indoor exposure. One study, for example, apportioned the particulate matter for two cohorts living in urban and suburban Beijing (Shang et al., 2019).
Ferro noted that low-cost monitors should prove invaluable for determining what particles come from outdoors and which originate indoors. Mass balance models are now used regularly for assessing indoor PM2.5 concentrations and human exposure to them.
Indoor Exposure Disparities
A 2011 framework developed by investigators at the Harvard T.H. Chan School of Public Health aimed to identify contributors to indoor exposure disparities (Figure 3-6) (Adamkiewicz et al., 2011). This framework acknowledges that there are disparities in the types of sources and their emissions depending on the specific community. Exposure factors, such as the built environment, number of people living in a residence, HVAC systems, air infiltration, and activity patterns, also vary by community. Ferro noted that looking at each of these factors systemically is important for addressing disparities, as is including the communities directly in this work.
Ferro raised the question of whether ambient air quality standards can be used to assess indoor air pollutants. In her opinion, the answer is no given that only some ambient particulate matter makes it indoors. As a result,
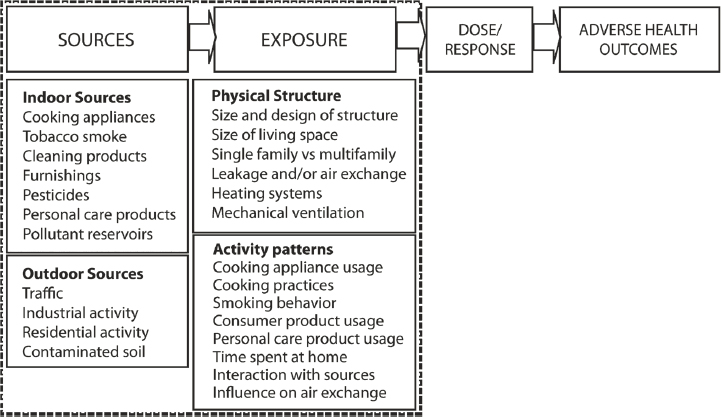
SOURCE: Ferro slide 15, from Figure 1 in the cited publication.1
1 Adamkiewicz G, Zota AR, Fabian MP, Chahine T, Julien R, Spengler JD, Levy JI. Moving environmental justice indoors: Understanding structural influences on residential exposure patterns in low-income communities. American Journal of Public Health, 101(S1):S238–45, 2011. Reprinted with permission from the American Journal of Public Health.
applying ambient health-based standards to assess indoor PM2.5 would underestimate risk. “We really need to separate the indoor-generated versus the outdoor-infiltrated particulate matter,” said Ferro. One approach might be to use disability-adjusted life years as a way to prioritize indoor sources for mitigation. She noted that the Global Burden of Disease study3 evaluates ambient PM2.5 and ozone pollution as well as household air pollution from use of solid fuels for cooking to assess the health risks associated with air pollution. She also discussed the modular mechanistic framework (Eichler et al., 2021) as useful for modeling semivolatile organic compounds.
Ferro called for research to separate indoor-generated from outdoor-infiltrated PM2.5 for epidemiological studies. She noted that it’s also important to update human activity pattern data—for example, by using new technology like GPS-enabled devices—rather than relying on data from 30 years ago. In addition, advanced metrics and approaches are needed to assess and communicate the health effects of indoor PM2.5 and to increase community-engaged research to better understand disparities in PM2.5 exposure and develop mitigation strategies.
DISCUSSION
Planning committee chair Richard Corsi opened the discussion by asking the panelists to put the issues they discussed in the context of possible disparities in underserved and underresourced communities. Vance responded that there is a huge knowledge gap about what to do to reduce exposure in terms of indoor cooking emissions for those who are economically disadvantaged. The most effective approach to reducing such exposure is a good, externally venting hood or portable air cleaner, but many people cannot afford or are not able to take those steps, particularly if they are renting. A first step is to develop additional knowledge about human activity patterns, determine how personal habits differ with socioeconomic status, culture, and even region of the country, and use that information to inform better experiments to understand realistic exposures.
Waring said that for secondary organic aerosols, there are three questions that if answered could start to yield a picture of disparities and secondary aerosol exposure among those who live in underserved or poorer communities:
- Are there differences in outdoor concentrations for underserved communities or are they in regions of higher ozone and organic or inorganic particulate matter concentrations, which can infiltrate and potentially drive secondary chemistry?
___________________
3 Information is available at http://ghdx.healthdata.org/gbd-2019.
- Are there differences in emissions of particular VOCs or precursors in housing types in underserved communities versus other communities, and are there different mixtures of VOCs present depending on the quality of the housing or the type of construction of the housing?
- Do individuals of different socioeconomic status use different consumer products that could generate more or fewer precursor chemicals?
Marr noted that she has been collaborating with a colleague on energy savings and building in underserved communities as these two factors pertain to indoor environmental quality. The residents of those communities, she said, are not concerned about ozone, volatile organic compounds, or plasticizers, but are very concerned about mold because they experience major asthma and allergy issues, which are more prominent in underserved communities. She wondered if the relatively sparse information in the literature on indoor exposure to mold has to do with the fact that this is predominantly a problem of underserved communities. Waring added that he was part of project in New York that measured mold in public housing, and it was everywhere. Elizabeth Matsui then noted that, while there are clear health effects associated with indoor PM2.5 exposure, they are smaller than those from exposure to biological contributors such as pest allergens that are more common in underserved communities and endotoxin concentrations that correlate with mouse allergens in the indoor air.
Both Ferro and Prather commented on the need to include occupations in these studies. For example, there are investigations being done on exposures for workers in nail salons and those who clean office buildings, as well as other occupations subject to higher or different exposures.
Marr raised the problem of funding research in this area given that it tends to fall into an “interagency hole” where it is not clearly in the purview of the National Institutes of Health or the National Science Foundation. Farmer traced the problem to the fact that there are so many factors that may influence indoor air quality and health, making it difficult to assemble the bigger picture that would lead to mitigation and policies. “There is a clear need, and I think all of the talks highlighted that…, to not only think about this complex indoor air environment and what we can do to improve it, particularly in underserved communities, but also to start having cross-disciplinary discussions,” said Farmer. Stephens added that resource constraints also limit what and who is studied, and Ivey stated that it boils down to money and the lower level of investment in the overall quality of life for people who are disproportionately exposed.
Planning committee member Seema Bhangar (WeWork) asked the panelists to discuss what they see as the biggest gaps in source control and if
policies or regulations that address source control would directly address the most vulnerable populations. Ivey replied that the two biggest gaps concern the current regulations on fossil fuel extraction and combustion. “If you look at domestic oil and gas extraction, where the wells are, how far away they are allowed to drill from people’s homes, there is a socioeconomic footprint and usually the burden is on communities of color.” She noted that environmental groups are calling for methane and ethane to be removed from exemption from volatile organic chemical classification, which would allow for more strict emission standards for methane and ethane, which disproportionately affect disadvantaged communities. Marr added that she would like to see a standard or at least a benchmark for mold exposure.
Prather asked the panelists if enough is known at this point to create a framework for establishing which exposures are bad and which ones benign. Vance said that more information is needed about the sources of particulate matter of different sizes and the health effects associated with particular exposures to identify what should be of greatest concern. Waring wondered how basing those decisions on levels of indoor concentrations would work, given the lack of knowledge in this area. Farmer cited the importance of understanding the fundamental processes at work—the actual drivers of particulate matter composition and chemistry—to avoid controlling the wrong source.
Chan offered a final question, asking where resources should be spent in schools, given what is known about indoor particulate matter. Waring said that the greatest need in the Philadelphia schools he is familiar with would be adequate ventilation.