3
COVID-19 Risks and Mitigation on Commercial Aircraft
A large part of the presentations and the discussion throughout the workshop centered on the twin questions of, “What are the COVID transmission risks related to flying?” and “What can be done to mitigate those risks so that air travel can get back to normal?” The air travel risks that have typically received the greatest attention are those associated with being on a commercial aircraft, and this chapter describes what was said during the workshop on that topic. But, as workshop participants agreed, it is important to take into account the other risks associated with air travel, especially those related to airports, and those discussions are described in Chapter 4. There is a certain amount of overlap between the two topics—wearing masks is an important risk mitigation measure in both aircraft and airports, for instance—and in those cases when speakers spoke about both, their comments are presented here and then briefly reviewed in the next chapter.
Several speakers pointed out that the risk of COVID-19 transmission in the airport or on an aircraft is not the only risk related to air travel. A significant concern is the direct transmission that can occur when an infected person from one part of the world carries the infection to another part of the world. However, beyond the discussions about how to prevent an infected person from getting on a plane in the first place, the workshop did not address the challenge of rapid transmission due to the increased mobility of the global population transmission or how to mitigate it.
COVID-19 TRANSMISSION ON COMMERCIAL FLIGHTS
In the early days of the COVID-19 pandemic, there were multiple anecdotal reports of passengers on commercial aircraft infecting fellow passengers, but a lack of concrete data makes it difficult to determine the reality behind the reports. Joshua Barocas, an assistant professor at the Boston University School of Medicine, discussed what is known about in-flight transmissions of the COVID-19 virus.
One study, for example, examined in-flight transmission of COVID-19 on 18 international flights into Greece between February 26 and March 9, 2020.1 On those 18 flights, there were 21 passengers, all but 6 of them asymptomatic, identified as index cases—that is, people who were potentially infected during the flights. Tracing
___________________
1 A. Pavli, P. Smeti, S. Hadjianastasiou, K. Theodoridou, A. Spilioti, K. Papadima, A. Andreopoulou, K. Gkolfinopoulou, S. Sapounas, N. Spanakis, A. Tsakris, and H.C. Maltezou, 2020, In-flight transmission of COVID-19 on flights to Greece: An epidemiological analysis, Travel Medicine and Infectious Disease 38:101882.
891 people from among the 2,224 passengers and 112 crew members on those flights, the researchers found only five who had been infected with COVID-19—four passengers and one crew member. All four of the passengers had been on the same flight and within two seats of two index cases who had symptoms during the flight.
A second study looked at a Vietnam Airlines flight from London to Vietnam with 16 crew members and 201 passengers, one of whom was symptomatic for COVID-19 during the flight. After the flight, 14 passengers and 1 crew member developed COVID, as confirmed by polymerase chain reaction (PCR) testing. Among the 14 passengers who became infected, 11 were seated within 2 meters of the index case.2
Yet another study examined transmission on a 15-hour flight from Boston to Hong Kong in early March 2020. Two passengers and two crew members were later found to have identical strains of the COVID-19 virus, and the researchers concluded that either one or both passengers, who were traveling together, had gotten the virus in North America and passed it on to the crew members during the flight. No other passengers or crew members were reported as testing positive.3
In a review, two researchers examined a number of flights where passengers flew with COVID-19. On three flights where there were two or more cases of in-flight transmission, a common variable was that masking was not required. By contrast, on five flights with a rigorously enforced masking requirement, there were no cases of COVID-19 transmission, despite the fact that the flights were all more than 8 hours in duration and that there were 58 passengers with PCR-confirmed COVID among the total of 1,500–2,000 passengers. On a sixth flight with 25 passengers with PCR-confirmed COVID and rigidly enforced masking, there were only two transmissions—one to a passenger who was sitting near five different people with COVID-19.4
The pattern that emerges from these studies, Barocas said, is that risk goes up sharply the closer an individual sits to a person with COVID-19, but the risk reduces significantly with mask wearing. However, because the studies are anecdotal, it is difficult concretely discern what the transmission risks are.
One recent study addressed that lack to some degree, he said. At the time of the workshop, the study was still in pre-print version. In that study, the authors modeled the risk of in-flight transmission on a 2-hour U.S. domestic flight with one case consisting of a completely full flight and another case where the middle seat remained unoccupied (Figure 3.1). The authors carried out 10,000 simulations to estimate the risks of COVID-19 transmission and concluded that a passenger on a flight with the middle seat remaining open had 1chance in 6,400 of contracting COVID-19, while a passenger on a flight with fully occupied seats had 1 chance in 3,900. Thus keeping the middle seat open cut the probability of infection almost in half. However, Barocas emphasized, the relative risk of becoming infected while flying is still high compared to the risk of a 2-hour walk down the street, which results in a 1 in 66,000 risk of contracting COVID-19. Flying carries significantly higher risks than staying on the ground, even though those risks can be reduced by taking certain measures, such as leaving the middle seat empty.
POSSIBLE MODES OF TRANSMISSION
Understanding the risk of COVID transmission—and finding ways to minimize that risk—begins with forming a clear understanding of the ways in which COVID or other infectious diseases can be transmitted from one person to another. Vicki Hertzberg, a professor at Emory University’s School of Nursing, provided a brief primer on infectious disease transmission, explaining that there are three major modes by which upper respiratory diseases are passed from one person to another—large droplets, aerosols, and fomites.
The definition of large droplets varies between sizes of 5 and 60 microns. The key attribute of large droplets, she emphasized, is that they contain both epithelial cells from the lining of the airways and immune cells. Additionally, they contain the electrolytes that are present in mucus and saliva as well as the infectious agents that reside in the upper respiratory systems—bacteria, fungi, and viruses. Large droplets are expelled from the mouth
___________________
2 N.C. Khanh, P.Q. Thai, H.-L. Quach, N.-A.H. Thi, P.C. Dinh, T.N. Duong, L.T.Q. Mai, et al., 2020, Transmission of SARS-CoV-2 during long-haul flight, Emerging Infectious Diseases 26(11):2617–2624.
3 E.M. Choi, D.K.W. Chu, P.K.C. Cheng, D.N.C. Tsang, M. Peiris, D.G. Bausch, L.L.M. Poon, and D. Watson-Jones, 2020, In-flight transmission of SARS-CoV-2, Emerging Infectious Diseases 26(11):2713–2716.
4 D.O. Freedman and A. Wilder-Smith, 2020, In-flight transmission of SARS-CoV-2: A review of the attack rates and available data on the efficacy of face masks, Journal of Travel Medicine 27(8):taaa178.
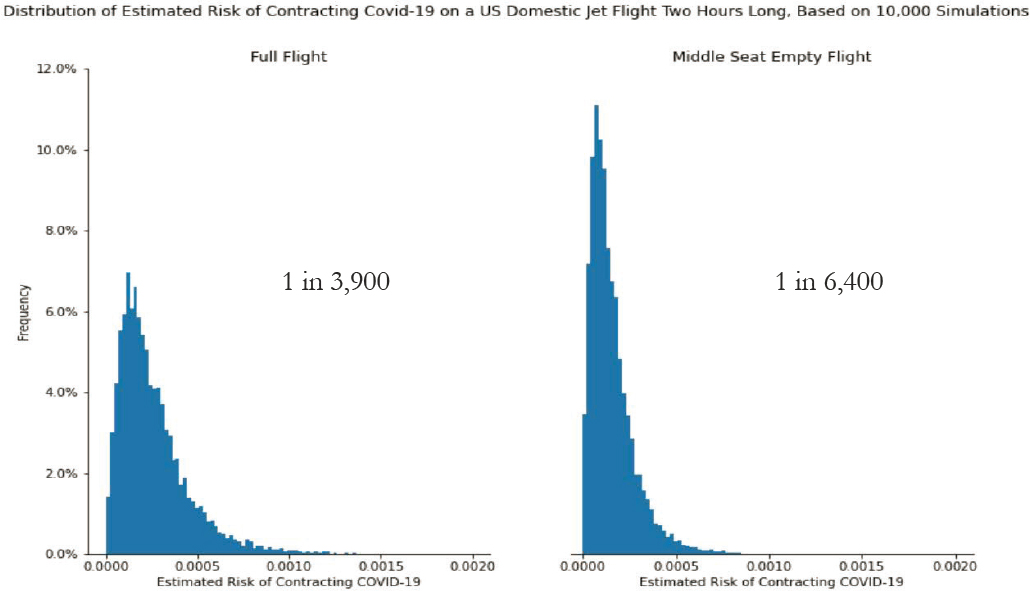
and the nose in multiple ways, including sneezing, coughing, talking, breathing, and singing. Once expelled, they fall quickly to the ground, generally within about 3 feet of their source.
Aerosols are generated in much the same manner as large droplets, Hertzberg said, but are smaller. Because aerosols also originate in the upper respiratory system, they have the same contents as large droplets. Their small size, however, allows them to remain suspended in the air for longer periods of time and thus travel much farther than 3 feet.
Finally, fomites, the third mode of transmission, are the surfaces on which infectious particles cling once deposited. The droplets and aerosols expelled from the upper respiratory tract can survive for hours or days once attached to a fomite, depending upon the microbe and the particular environment. Consequently, fomites facilitate indirect transmission from person to person. For example, she said, “if while I sneeze I cover my mouth, and then leave the room and shut the door with the door handle, I have deposited expelled matter from my upper respiratory tract on the door handle. You come along and you touch that door handle, and then later on you touch your hands to your face, and therefore you now can experience the disease, even though you and I were never in direct contact.”
Scientific Studies of Transmission Risks
Given the relative paucity of epidemiological data on in-flight transmission of the COVID-19 virus, a number of researchers have looked for other ways to get a clearer understanding of the risks of such transmission. Hertzberg described an observational and modeling study designed to elucidate passenger movements on planes and what those movements say about the risk to other passengers of sitting on a plane with a person with COVID. Joshua Santarpia, an associate professor of pathology and microbiology at the University of Nebraska Medical Center, described an
experimental study aimed at understanding how aerosols disperse in aircraft cabins. Charles Gerba, a professor of virology in the Department of Environmental Sciences at the University of Arizona, spoke about his work investigating the risk of viral transmission via fomites (i.e., surfaces with virus particles), describing what is known about the risks of transmitting the COVID-19 virus in flight and discussing some approaches to mitigating that risk.
FlyHealthy Study
Hertzberg described the FlyHealthy study, funded by Boeing and Delta, which was designed to explore how the coronavirus is transmitted inside commercial airplane cabins. The study’s results are applicable to other diseases as well, she said, noting that with billions of commercial flights taking place around the world each year, the spread of disease by air travel is a significant global health concern. In particular, she said, there have been many documented cases of diseases transmitted on airplanes, including tuberculosis, measles, influenza, severe acute respiratory syndrome (SARS), meningococcal infection, norovirus, cholera, and now, of course, COVID-19.
As an example, she showed a diagram of transmission of SARS on well-studied flights from Hong Kong to Beijing. The index case—the person who boarded the flight with SARS—sat in seat 14E, and 16 other passengers and crew members were later diagnosed as having SARS, with another 6 likely cases.5 While many of those infected were within two or three rows of the index case, others were not close. The goal of the FlyHealthy study was to address that question of how the transmission happens, she said. In particular, the study aimed to characterize the airplane cabin microbiome, to quantify transmission opportunities, and to create seat maps defining the risk of infectious disease transmission from an infected individual.
To gather data, a team of graduate students and postdoctoral fellows took flights—a total of 10 flights, or 5 round trips—between Atlanta and various destinations on the West Coast. During those flights, the students recorded the behaviors and movements of passengers and flight attendants when the plane was in its cruise phase, above 10,000 feet. Using those data, Hertzberg’s group reconstructed all movements of the passengers and flight attendants during the cruise phases of the 10 flights. The students also took environmental samples of the air and various surfaces common surfaces accessed by passengers and crew before, during, and after each flight. The team of graduate students and postdoctoral fellows later used these data to characterize the airplane cabin microbiome of each flight.
She described the microbiome findings first. The bacterial communities found on the planes were largely derived from human skin and oral commensal bacteria as well as environmental generalists, she said, and the team identified a core airplane cabin microbiome. They examined the collected environmental samples for viruses, but all tests were negative. There were very large flight-to-flight variations in the types of the bacteria identified, however, but there was no systematic pattern of change from pre- to post-flight microbiome. Finally, she said, the core microbiome found in the plane cabins demonstrated significant similarities with the core microbiome found in an earlier study of the Boston subway system done by Harvard School of Public Health.
Using the data collected on the behaviors and movements of the passengers on the 10 flights studied, Hertzberg and her students created what she called “fantasy flights”—models of flights in which one passenger was infected with a virus. The interactions the infected passenger had with other passengers and crew members were modeled according to probabilities derived from data taken by observing the passengers and crew on the flights studied. The team generated hundreds of thousands of such 4-hour fantasy flights to calculate the probabilities of the infected passenger passing the virus on to others on the plane. For the modeling, the team employed a transmission probability of 0.018 for 1 minute of close contact—a parameter published in a 1979 article in the American Journal of Epidemiology that examined an outbreak of influenza aboard a commercial airliner where about three-quarters of the passengers became ill after the airplane sat on the tarmac for several hours.6
___________________
5 S.J. Olsen, H.-L. Chang, T.Y.-Y. Cheung, A.F.-Y. Tang, T.L. Fisk, S.P.-L. Ooi, H.-W. Kuo, D.D.-S. Jiang, K.-T. Chen, J. Lando, K.-H. Hsu, T.-J. Chen, and S.F. Dowell, 2003, Transmission of the severe acute respiratory syndrome on aircraft, New England Journal of Medicine 349(25):2416–2422.
6 M.R. Moser, T.R. Bender, H.S. Margolis, G.R. Noble, A.P. Kendal, and D.G. Ritter, 1979, Outbreak of influenza aboard a commercial airliner, American Journal of Epidemiology 110(1):1–6.
From hundreds of thousands of simulations, Hertzberg’s group calculated the likelihood of infection for each person sitting on a plane if the index case sat in seat 14C, an aisle seat in the 14th row. The passengers in seats A, B, C, and D on the 13th, 14th, and 15th rows all had a high probability of being infected—greater than 80 percent—but for passengers seated elsewhere, the probability was small. In addition, the probability for a flight attendant to become infected was under 20 percent. Thus Hertzberg’s research identified a “zone of risk” for those passengers sitting very near the infected person, beyond which the odds of infection remained small.
Summing up the FlyHealthy study, Hertzberg said, there are a number of takeaways. First, however, she cautioned that the data were taken in the economy cabin on flights that were close to full at a time when there were no masks and no restrictions on moving around. Given that, she said that they found that passengers and flight attendants move around the plane a lot, and the longer the flight, the more they move around. The core microbiome consists mainly of things “that you would find in a place where a lot of people inhabit”—commensals and mutualists, mostly. And the main risk of infection from an index case lies with those passengers within one or two seats, but the odds are that at least one other person somewhere on the plane will also be infected.
The study had limitations; there remain a number of unknowns, she said. For one, the results apply only to flights on single-aisle planes that are about 5 to 6 hours long. “We don’t know what happens on long-haul flights, other than . . . people move around even more. We don’t know what happens in double-aisle planes in terms of how much movements are restricted to the aisle closest to the seat of a passenger.” Furthermore, the study does not address the potential contributions that aerosols and fomites might make to infectious disease transmission on planes. Finally there is no way to concretely identify the source of a viral infection transmitted on a plane, even when it is known which passengers are infected.
A lot of work has been done on aerosol exposures on airplanes, she said, but the work generally fails to account for either passenger movement or air movement. Furthermore, there is little known about the role of fomites in transmitting infections on a plane.
Given the relative dearth of data and a firm understanding of how virus transmission occurs on airplanes, Hertzberg had some simple advice for anyone who flies on a commercial plane: “Get a window seat, don’t move, and wash your hands and keep those hands away from your face.”
Aerosol Dispersion in Commercial Aircraft Cabins
Santarpia described the aerosol dispersion research his group conducted, sponsored by the U.S. Transportation Command, on the conditions of Patriot Express flights. Further assistance was provided by the Defense Advanced Research Projects Agency, Boeing, and United Airlines.
The main goal of the study, Santarpia said, was to understand the risk factors on the Patriot Express flights—offered to members of the U.S. Armed Forces and their families—in order to determine how many people could safely fit on a flight and to develop recommendations for safely operating the flights. The research focused on understanding aerosol transmission, not disease transmission, “because that’s more complicated than just aerosols,” he said. However, he went on to explain, the data from aerosol dispersal studies are valuable inputs in developing models of disease transmission.
The studies were fashioned with a manikin serving as the stand-in for an infected passenger. The manikin was placed in a seat on a plane, either a Boeing 767 or a Boeing 777, and an aerosol nebulizer in the manikin would release “breaths” containing aerosol particles. The breathing simulation involved expelling breath at 1.4 meters per second from the mouth of the manikin, with each breath lasting 2 seconds at an interval of 2 seconds. The speed of the exhalation was intended to simulate breathing, not coughing, speaking, or other modes of human respiration, Santarpia said. Some of the simulations were done with the manikin masked, and others were done with it unmasked.
An important point, he said, is that the amount of particulates released by the manikin was much larger than would be released by a single person, to make it easier to make the measurements. “Too small a number of particles, and you fool yourself,” he said, “because your limits of detection won’t be sufficient to see them.”
To track the movement of the aerosols around the plane, the team used 1-micron fluorescent tracer particles to facilitate particle detection around the cabin. The team also used DNA-tagged tracers that allowed exhalations
to be uniquely identifiable and, in particular, distinguishable from any other particles resident on the plane. The DNA tracers were used mainly to determine where the aerosol particles attached to surfaces around the plane. The DNA tracers were larger than the fluorescent tracers—3 microns instead of 1 micron—to simulate the larger aerosol particles more likely to end up as fomites on surfaces.
To carry out the tests, the team placed fluorescent particle detectors at various points around the cabin and had the manikin “breathe” for about 6 minutes while taking real-time measurements with the detectors. A total of 300 individual aerosol tests were carried out using 40 real-time sensors and 46 different positions around the 767 and 777 airframes.
An important limitation of the study, Santarpia commented, was the use of only two airframes, the 767 and 777, because of their relevance to the Patriot Express flights. Thus, in particular, the study did not include the more common Boeing 737 line of aircraft, which makes up a large portion of the domestic air travel fleet.
At the workshop, Santarpia focused on the results from the in-flight testing of the dispersion of the manikin’s breath throughout the cabin, but mentioned that the group also did some research on loading and unloading scenarios as well. He also focused on results obtained from the economy cabin rather than the first-class cabin, because the results from first class were similar. The one difference was that the aerosol penetration is generally lower in first-class because the seats are farther apart then in economy and often partially isolated with walls or other barriers.
Synopsizing the results, Santarpia said that, overall, the 767 and the 777 the mean particle reduction in the seats immediately surrounding the release point was 99.97 to 99.99, with the 767 coming in around 99.97 to 99.98 and the 777 from 99.98 to 99.99. There was also a slight difference between aft and mid aft in economy, he said, although it’s unlikely to be significant. There were also some extreme outliers in the measurements, which corresponded to the seats immediately next to the manikin. In other ways, the immediate neighbor of the person with the virus is the one most likely to be infected but also the one in which there was the most variability in the data (Figure 3.2).
Santarpia explained that the ventilation systems in aircraft are designed not to move air fore or aft but rather move it from the top of the cabin to the bottom. The data supported the design approach, except for the 777, where the analysis showed that particles also move slightly to the aft, indicating some general aft air flow in the 777. By contrast, the 767 had a slight aftward mixing in the rear of the plane and some forward mixing for releases toward the front.
The analysis showed that surface contamination by the aerosols released by the manikin were minimal, Santarpia said. Surface deposition was typically highest on the armrests and seats near the point of release. He cautioned that the analysis only examined surface deposition from aerosols, but did not address droplet transport direct contamination due to transmission by, for example, touching a hand rest after sneezing into a hand. But as far as aerosol deposition on surfaces is concerned, it is very localized to a small area close to the infected person.
There was relatively little difference in aerosol dispersion between masked and unmasked manikins, Santarpia said. In particular, his group found an average 15 percent drop across all sensors when the manikin was masked compared with unmasked, but the difference varied widely from sensor to sensor—some seeing an increase in aerosol particles and others seeing a decrease. A variation in sensor readings between the masked and unmasked conditions is not surprising; the presence of a mask is expected to redirect the manikin’s “breath.” When the rate of exhalation was changed to represent a cough—about 12 to 13 meters per second—the masks were much more efficient in reducing the flow because, he said, the increase in air flow due to the cough forced the particles through the mask rather than particles leaking around its edges, as happens in normal breathing. The study used the typical three-ply surgical masks being worn during the pandemic, he said.
Several key conclusions can be drawn from the study, Santarpia said. “The key thing is that when the plane is in flight and the heating, ventilation, and air conditioning (HVAC) is operating in an optimized fashion, the rapid dilution and mixing and purging of aerosol from around every person on the airplane and the airframe’s high exchange rate and downward ventilation design probably limit the transport of aerosol throughout the aircraft, which then minimizes aerosol exposure risks.” In short, a passenger’s risk of being exposed to aerosol produced by a nearby infected person is significantly lower because of the cabin airflow on the Boeing 767 and 777. The average breathing-zone penetration from nearby seats is just 0.0148 percent for the 767 and 0.0176 percent for
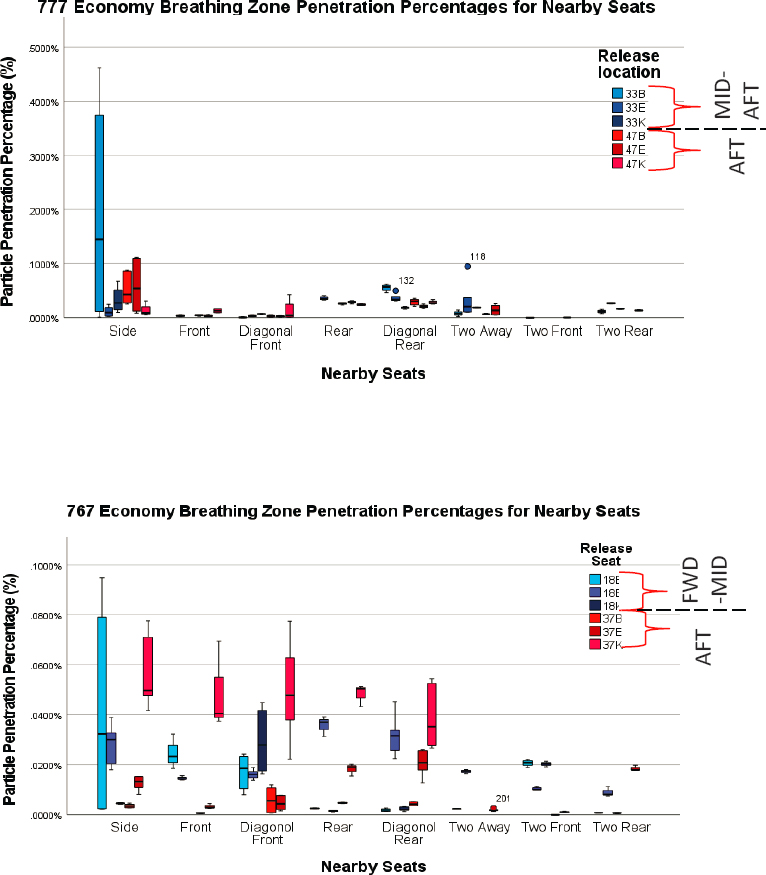
the 777, corresponding to about a 99.98 percent reduction in aerosol. The maximum aerosol penetration, which occurs for people sitting adjacent to the infected person, is still less than 0.5 percent.
An infected person wearing a mask does reduce the exposure risk for other passengers somewhat. The experiment did not measure the reduction achieved by those other passengers wearing masks. “The big picture of this,” Santarpia said, “is this does explain why we haven’t seen large-scale outbreaks on aircraft or related to air travel, at least none that have been reported.”
The study has several limitations, he said. It is not clear how well the conclusions apply to the Boeing 737 and other planes besides the 767 and 777, for instance. A key limitation is that it only studied disease transmission via the aerosol route—that is, small particles exhaled or coughed out by an infected person and inhaled into the lungs of someone else. Contact transmission and transmission via ballistic droplets were not part of the study, and
Santarpia and noted that seats with the highest aerosol penetration are also the ones most likely to be affected by these other routes. The study also assumes that an aircraft’s ventilation system is running constantly within the parameters that existed during data collection. If the aircraft HVAC system is turned off for any period of time, aerosol transport will be dramatically affected. In such cases, the airplane “goes from being a well-ventilated, enclosed space to being a closed-off, shiny metal tube,” he said.
An important constraint of the study that likely affected results was that that the manikin always faced forward, and there was minimal movement in the aisles. “We’ve done a little bit of limited follow up testing,” he said, “and turning the manikin’s head to the right as if someone were turning to talk to you dramatically changes that immediate impact.” The impact was especially true for the unmasked case because the person’s breath points directly toward the neighboring passenger. Furthermore, significant movement in the aisles disrupts the airflow and changes how the aerosols disperse. Thus the movement of crew members and passengers up and down the aisle, or when seated, turning to address a seat mate, can affect an individual’s aerosol exposure on these flights.
The Role of Fomites in the Spread of Viruses
Fomites, or surfaces that have been contaminated by the virus, are major virus transmission vectors. Especially now, viral transmission by fomites is very probable, Gerba said, “We share more common surfaces with more people than at any time before in history,” he said. “We’re working in larger buildings and larger stadiums, we go to larger malls and larger airplanes, so you’re touching more surfaces during a day than people have ever touched in history.” The result, he said, is “the perfect storm for the spread of COVID-19.”
Consequently, it is important to gain a better understanding of how viruses spread via fomites and, more importantly, the best ways to minimize the spread. Epidemiology does not provide particularly precise or accurate answers about the risks of fomite transmission, Gerba said, but epidemiological studies require large numbers of people who have been exposed to obtain statistically significant data. In addition, if there are multiple possible transmission vectors, epidemiological studies have difficulty separating the different transmission vectors. When the Environmental Protection Agency (EPA) faced a similar problem three decades ago, he said, they adapted a quantitative microbial risk assessment methodology to set standards for disinfectants for treating drinking water. The EPA’s approach “allows the expression of the risk in a quantitative fashion in terms of infection, illness, or mortality from microbial pathogens,” he said, and it has been adopted by World Health Organization to set standards for the treatment of food and water.
The quantitative microbial risk assessment approach has various components. One important component is obtaining dose-response data for the pathogen of concern. Unfortunately, dose-response data are not yet available for the COVID-19 virus, he said, but are available for both the 2003 outbreak of severe acute respiratory syndrome (SARS) and for coronavirus 229E, both of which are related to COVID-19. Furthermore, Gerba added, his colleagues have modeled what the dose response is likely to be for COVID-19, and it is similar to that of coronaviruses.
In addition to dose-response data, another component required to use the quantitative microbial risk assessment method is knowledge about the probability of infection by COVID-19 when a person is exposed to a certain level of the virus. Given the data on probability of infection, it is possible to use clinical data to estimate the probability that an infected person gets sick or dies. But the key is knowing what an individual is exposed to in the environment. If you know that, you can determine what your probability of getting infected is.
Figuring out what an individual is exposed to in a particular environment can be done in several ways, Gerba said. One way is to test the air and surfaces for the presence of the virus. Another is to release a tracer virus that doesn’t infect human beings and examine its levels in the air and on various surfaces. Gerba’s group has used that technique often to predict exposure in a certain environment, he said, and that work has been validated with epidemiology data on various outbreaks in the past that were linked to contaminants on surfaces and in air, water, and food, which has given him confidence that the approach is reliable.
Tracing studies have demonstrated how quickly and how far viruses on surfaces can propagate. Gerba said his group has done studies in which they released bacterial viruses similar to human viruses in office buildings to study transmission. “We put the bacterial virus on one person’s hand, or in another case, we planted the virus on the door knob of an office building with about 100 people,” he said, “within 4 hours, 50 percent of the surfaces are contaminated with the virus.” Experiments conducted in in hotels, conference settings, health care environments,
and nursing homes yielded similar results. People should not underestimate how quickly a virus can be transmitted from surface to surface, he said. “In fact, an individual’s exposure in an office building is much greater than an aerosol exposure, measured in the same time frame, because of the ongoing contact with common surfaces.”
A major factor in transmission risk from surfaces is how much of the virus a person gets on his or her finger. A number of finger-transfer studies have been done for non-envelope viruses, such as rotavirus, which causes diarrhea. Before the current pandemic, no similar studies had been conducted for coronaviruses. Since the beginning of the pandemic, Gerba’s group has been studying finger-transfer of COIVD-19. It is necessary, he said, for assessing how important fomites are in the transmission of the virus. A major finding from Gerba’s studies is that the likelihood of picking up a virus from a surface depends on the type of surface—but in a manner that differs from the pattern exhibited by the noroviruses. In the case of the COVID-19 virus, the likelihood of virus transmission is greater for porcelain surfaces than for Formica. The likelihood of COVID-19 transmission is also pretty low on stainless steel, he added, which is again a different pattern from what is seen with non-envelope viruses. Knowing which surfaces are most likely to lead to transmission of the COVID virus is useful, Gerba said, because it makes it possible to determine when risks are greater and which surfaces should be targeted for treatment.
MITIGATING THE RISK OF COVID-19 TRANSMISSION ON AIRCRAFT
Several presenters spoke about various ways of mitigating the risks of COVID-19 transmission onboard aircraft. Both Barocas and Gerba discussed mitigation as part of their talks. Caitlin Rivers, an assistant professor at the Bloomberg School of Public Health at Johns Hopkins University, spoke about the use of non-pharmaceutical interventions, such as screening and mask wearing, to prevent transmission in general settings, not necessarily only on aircraft. In particular, she emphasized the value of combining multiple interventions to decrease the risk of transmission. Joseph Allen, an associate professor at the T.H. Chan School of Public Health, Harvard University, reviewed the 2013 National Academies report Infectious Disease Mitigation in Airports and Airplanes7 and spoke about how the lessons from that report could be applied to reduce the spread of COVID-19.
There was general agreement among the workshop participants about the different techniques that can and should be applied to reduce the risk of COVID-19 transmission, so to avoid redundancy, not every recommendation by every participant is reproduced here.
Containing COVID-19: Reducing Risk Through Layered Non-Pharmaceutical Interventions
Rivers began by stating two key questions for assessing the risk of transmission in a particular setting, such as air travel. The first question, adapted for air travel is, “What is the probability that someone who boards an aircraft is infected?” The answer depends on several factors, she said. The most important one is community prevalence. The more that a particular virus is circulating in the community where people are traveling from, the more likely it is that one of the people who board the aircraft will be infected. This community prevalence factor can be modified to some degree by keeping people off the plane who have tested positive for the virus or who show symptoms, such as an elevated temperature. However, Rivers noted, that from the perspective of the air travel industry, the community prevalence factor is largely immutable. “We don’t have a lot of control over what’s happening in the community.”
It is also difficult to estimate the risk of virus introduction into a plane, she said, because the travelers are coming from so many different places, and the rates of infection often differ widely from place to place. It is much easier to estimate the risk of introduction in school, for instance, because the people who attend a school come from a particular community where there is likely to be a well-known level of infection. “But when you start to look at international air travel, people come from all over, and it’s really difficult to say what that exact mix is.”
The second component in assessing the risk of transmission in a particular setting is determining the risk of onward transmission. That is, if someone is to board a plane who is infected, what is the probability that this
___________________
7 National Academies of Sciences, Engineering, and Medicine (NASEM), 2013, Infectious Disease Mitigation in Airports and Airplanes, Washington, DC: The National Academies Press, https://doi.org/10.17226/22512.
person will infect someone else? Airlines can exert much more influence over this factor, Rivers said, using various non-pharmaceutical interventions, such as masks and social distancing. A key fact about these non-pharmaceutical interventions is that they work best when they are layered. No single intervention holds the key to preventing transmission, but if several are stacked, it is possible to sharply reduce the risks.
One other factor influences the risk of transmission on planes, Rivers said, but is an element that the air travel industry can exert less control over—compliance. “We can recommend things, we can even require things, but if people are not complying or choosing not to take those measures,” she said, “then obviously the effectiveness will be reduced.”
Next, Rivers went into each of the factors affecting the risk of transmission in greater detail. She first discussed estimating the risk of a plane having a passenger that is infected. She offered a simple example of passengers coming from one of three states, each with a different level of infection. Consider a flight with 100 people leaving with passengers from Hawaii, which at the time of the example had a measured infection rate of 7 cases per 100,000 people per day. Assuming that those numbers are accurate, there would be about a 7 percent chance that at least one of the 100 persons boarding the plane would be infected with COVID-19. In the case of New Mexico, where the infection rate was 32 cases per 100,000 people per day, the chances of a person bringing COVID-19 onto a 100-person flight would be about 27 percent. And for Texas, which at the time had the highest rate in the country, at 73 cases per 100,000 people per day, the odds would be 46 percent.
But, of course, there are always many cases of COVID-19 that go undiagnosed in a community, so these numbers are underestimates. Assuming that only one of every five cases of COVID-19 are actually recorded, so that the rates of incidence are five times higher, Rivers calculated a new set of odds of a passenger on a 100-person flight having COVID-19. In the case of Hawaii, there is a 31 percent chance, for New Mexico a 79 percent chance, and for Texas a 96 percent chance. This helps provide some idea about what to expect concerning the likelihood of passengers boarding a plane with COVID-19, she said.
That risk can be reduced in various ways, she added. One way would be to test people in order to prevent those with active infections from boarding a plane and traveling to other locations. Ideally, Rivers said, people would learn before beginning their trip that they are infected and would be able to stay home, but it could also be that people are denied boarding at the airport. Either way, it would be useful because not only would it protect other people who are traveling, but it also protects the people at the aircraft’s destination. Testing does have drawbacks, Rivers noted. For one, it does not identify people who have recently been exposed and are incubating the virus. This is less important for the people traveling with the exposed person, but it can be quite important for the people in the receiving community. Another weakness of testing, depending on the particular test used, is the presence of false negatives (such as for recently exposed people) and false positives. PCR tests, for example, may identify people as positive who have recovered from an infection and are no longer infectious.
The Centers for Disease Control and Prevention (CDC) require people entering the United States from another country via air travel to have had a test for COVID-19 within 3 days of departure, Rivers said, but there is currently no requirement for domestic travelers and no requirement for travelers crossing over-land borders.
In November, Rivers said, authors affiliated with CDC released a preprint in which they estimated the reduction in risk of infection produced by various interventions or combinations of interventions. Testing alone reduced the risk by up to 40 percent, depending on when the testing was done. Testing done more than 3 days in advance of travel did very little, and testing done 3 days before travel caught only about 10 percent of infections, but that number got better as the departure got closer, with testing on the day of departure identifying more than 40 percent of infected passengers. Adding symptom monitoring improved the results, from about 30 percent when the testing was done 3 days before departure to nearly 60 percent when done on the day of departure. “It’s not foolproof,” Rivers said, “but it can help to reduce the risk of introduction of a case onto the aircraft for the receiving community.”
Temperature or symptom screening by itself, while low cost, is not particularly effective, she continued. A 2015 paper in eLife8 reviewed the use of various airport screening methods—taking passengers’ temperatures and asking
___________________
8 K.M. Gostic, A.J. Kucharski, and J.O. Lloyd-Smith, 2015, Effectiveness of traveler screening for emerging pathogens is shaped by epidemiology and natural history of infection, eLife 4:e05564, doi:10.7554/eLife.05564.
about symptoms and possible exposure—that had been used in outbreaks of SARS, Ebola, and other diseases and found that they caught almost no cases of infected passengers planning to board planes. Such screening is likely to be of similarly low value with COVID-19, Rivers said, particularly because COVID-19 asymptomatic and presymptomatic infections account for a substantial fraction of onward transmission, probably around 40 percent. However, she added, because such screening is fairly inexpensive, she doesn’t “categorically recommend against it,” nor does she think that it can be relied on to reduce risk to any substantial degree.
Rivers next discussed the probability of onward retransmission; that is, the likelihood that an infected person on a plane passed the virus on to someone else, and she listed three key points on that topic. First, the probability of onward transmission is a function of mitigation measures, such as mask wearing and social distancing on the plane. “There is no one single strategy that is particularly effective at reducing onward transmission,” she said, “but when used in combination they can be quite effective,” which is the second key point: layering mitigation measures will improve effectiveness. Third, the marginal value of each mitigation measure is not clear. These values are difficult to determine because most of the data come from observational studies, which have little way to separate out the influences of each individual measure.
There are three primary modes of transmission for COVID-19, Rivers explained. “The first is droplets, which are the spit that we all produce when we’re talking or coughing or sneezing.” Next is aerosols, which are produced in the same way but which are much smaller and can stay in the air for much longer. And the third is fomites, which are surfaces that may be contaminated by the virus—a railing on stairs, for instance. Noting that it is difficult to figure out the relative contribution of each mode of transmission because infected people spread the virus through all three, she described the results of a paper recently published in the Proceedings of the National Academy of Sciences U.S.A. on which Joseph Allen, the speaker who followed Rivers in the workshop, was the senior author.9 Studying how the virus was transmitted on the cruise ship Diamond Princess, Allen and his co-authors concluded that airborne transmission was the major way the virus was spread on the ship and that aerosols in particular were a substantial contributor. Fomite transmission played a smaller role.
What this implies, Rivers said, is that preventive measures aimed at keeping droplets and aerosols from getting out and circulating are very important, while cleaning and disinfecting is of somewhat less importance, although certainly not unimportant.
Next Rivers discussed why the various non-pharmaceutical interventions are effective and their likely contribution to reducing the risk of onward retransmission. After some initial confusion as to whether members of the public needed to wear masks, it has become clear, she said, that masks are crucial to slowing the spread of the COVID-19 virus. They work both by keeping infected people from spreading the virus particles present in the air that they exhale and also providing protection for the wearer. N95 masks, which are worn by health care workers when dealing with COVID-19 patients, are very effective at preventing infection for the wearer, but surgical masks are also quite effective, and even lower-quality non-medical masks provide some protection for the wearer. The best masks are those with multiple layers, including a mostly impermeable layer, and having a charged layer that helps prevent infectious particles from passing through is also valuable. Cloth masks are less effective but still confer some protection.
Other non-pharmaceutical interventions include distancing, surface clearing, and improved ventilation and filtering. Social distancing is effective, Rivers said, but as she noted, there is fairly limited opportunity to do distancing on an aircraft. Surface cleaning is not thought to be of major importance because fomites are not thought to be a primary driver of transmission, but the situation may be somewhat different on aircraft, she said, because “there are extremely high-touch surfaces that hundreds of people can touch, maybe not in the airplane itself but in the airport. Furthermore, the cool dry air of airports and airplanes probably helps the virus to survive on surfaces longer. So frequent cleaning and disinfection is still sensible in air travel environments. Finally, variation ventilation strategies can reduce the risk of COVID-19 transmission, including increasing outdoor ventilation, creating directional airflow with fans, adding high-quality filters, and, potentially, using ultraviolet germicidal irradiation. The bottom line with ventilation, she said, is that the more air exchanges per hour, the faster the virus is cleared from the air.
___________________
9 P. Azimi, Z. Keshavarz, J.G.C. Laurent, B. Stephens, and J.G. Allen, 2021, Mechanistic transmission modeling of COVID-19 on the Diamond Princess cruise ship demonstrates the importance of aerosol transmission, Proceedings of the National Academy of Sciences U.S.A. 118(8):e2015482218.
A Physician’s View of Reducing Risk in Flight
“I think of airplanes as temporary congregate settings,” Barocas said, “and when we think about mitigation efforts in congregate settings, we use a framework called the hierarchy of control.” This framework starts with what can be done to mitigate risk on the level of an individual and moves all the way up to the facility level—in this case, the airplane.
Individual-level protection is the sort of personal protective equipment (PPE) that Rivers talked about—masks, gloves, and so on. “PPE, specifically masks, is essential to the protection of passengers and crew,” Barocas said. Masks should be worn at all times, and N95 masks are ideal for the flight crew. The cleaning crew should be wearing surgical, if not N95, masks. And passengers should be wearing masks even when in the lavatory.
The next level up is administrative controls, which includes such things as modifying how people board and move about the cabin. “There are ways that we can get people to board that maintain social distancing while boarding and can decrease the boarding time,” he said. Some of those methods involve back-to-front boarding, while others are very elaborate schemes that depend on the number of groups, the types of luggage coming on, and other factors. Other administrative controls include things like limiting unmasked time, staggering eating and drinking, and minimizing crew movement.
Then, Barocas discussed environmental controls. How can people be isolated from the virus if it is on board? The best way is with antigen testing to identify people who are infectious. Some antigen tests deliver their results in 15 to 20 minutes. “These are excellent ways to ensure that the virus doesn’t actually come on to the flight,” Barocas said, “or, if the virus is on the flight, that the person may not be actually infectious at that time.” And if an infectious person is on a flight, increasing ventilation and passing cabin air through HEPA filters is the best hope for restricting transmission. Noting that these things are already standard on aircraft, Barocas said they are “incredibly effective at decreasing the risk of transmission in the near vicinity.”
In closing, Barocas said that as a physician he wanted to make sure that people also think about the care of a symptomatic patient who might be on board. Because someone who is in respiratory distress is more likely to transmit the virus, it is important that as whoever is providing care to that patient do it in such a way that the people nearby are not exposed or at greater risk. Possible tactics include putting the symptomatic person in an isolation seat, one that has either been reserved on the plane or that is created by moving people away, providing N95 masks to people who are nearby, as well as to the patient and crew, and to have in-flight antigen testing to confirm the infection and, if necessary, lay the groundwork for contact tracing.
Insights from the National Academies of Sciences, Engineering, and Medicine Report Infectious Disease Mitigation in Airports and Airplanes
Following Rivers’s talk, Joseph Allen spoke about some of the findings in the 2013 Infectious Disease Mitigation in Airports and Airplanes10 report and discussed in particular parts of that report that are applicable to the current pandemic.
Concerning making airplanes safer, one issue that is often overlooked is gate-based ventilation. This was identified as a problem in the National Academies report, and it is likely still a problem, Allen said. That report recommended improving gate-based ventilation of aircraft in various ways, such as attaching gate-based ventilation systems to aircraft as soon as the aircraft is shut down and leaving them attached until shortly before aircraft startup. And while the report recognized that such ventilation is expensive, and thus routine use may not always be warranted, it specifically advised that such systems be available and used for all parked aircraft in case of a pandemic. Unfortunately, he said, “the reality is, this is not always happening.”
Referring to a study by his research team that looked at carbon dioxide levels in an aircraft cabin during different phases of a flight—boarding, ascent, cruise, descent, and deplaning—Allen showed that while the air quality is good during the cruise portion of the flight because there is outside air regularly being circulated into the plane, the carbon dioxide levels during boarding and deplaning indicate that the plane is under-ventilated during those
___________________
10 NASEM, 2013, Infectious Disease Mitigation in Airports and Airplanes.
phases when the plane’s ventilation system is not running.11 Indeed, according to that same study, aircraft regularly fail to meet the Federal Aviation Administration regulation for acceptable air quality.
Interesting enough, he said, with one recorded COVID-19 outbreak on a long-haul flight, the plane stopped for refueling, so the ventilation system was turned off during that 30-minute period. Even though many people have suggested that the flight was risky because it lasted so long, he said, “my assumption would be that the highest-risk point of that flight was refueling when the ventilation system was off.”
There are also benefits to good ventilation beyond decreasing the risk of COVID-19, Allen said. In particular, ventilation has been show to influence cognitive function in office workers and also to influence airline pilot performance. In a study that his group carried out, pilots were tested by being asked to carry out 21 different advanced maneuvers in an aircraft simulator where the cockpit of the simulator had different ventilation rates. The maneuvers included such things as responding to a fire in an engine at take-off. When the carbon dioxide levels were higher, Allen said, the pilots were less likely to perform the advanced flying maneuvers correctly. In short, ensuring good ventilation on planes can pay off in a number of ways.
The National Academies report also had recommendations for dealing with aircraft passengers in order to decrease the risk of disease transmission, and one of the recommendations was to carry out a healthy traveler campaign. As part of that campaign, the airline would distribute health traveler kits containing masks, gloves, hand sanitizer, and alcohol hand wipes. “Masks are absolutely the first line of defense, the most important line of defense,” he said.
Not all masks are created equal, though, he said. Their efficiency is dependent on their filtration and their fit. He offered a “cheat sheet” of recommended masks: Use an N95 if possible. If not, then the KF94 from South Korea or the FF2 from Europe. Next is the KN95, but only if it is from a manufacturer with a NIOSH certificate, because there have been reports of counterfeit KN95. Next would be double-masking with a good surgical mask for filtration covered by a cloth mask on top to improve the fit. After that, a blue surgical mask or a good three-layer cloth mask with a good filter would be recommended.
Further Tools
After his discussion about understanding the risks of fomites, Gerba described a number of tools that have been developed or are being developed to minimize transmission risks on aircraft. To find the most effective antiviral treatments, for example, Gerba’s group has been working with Boeing to develop what he called “a pandemic toolbox of environmental interventions.” They are examining, for example, anti-viral polymeric quats. Quats, or quaternary ammonium chloride compounds, are surfactants that have anti-viral properties. They can last anywhere from 48 hours to 90 days, Gerba said, which makes them useful for keeping surfaces free of viruses in between regular cleaning and disinfecting regimens.
His group has also been collaborating with Corning to develop new types of anti-viral paints. Some of these anti-viral paints contain copper, which can kill the COVID-19 virus within 1 minute after contact.
The group has been testing these surface interventions in actual airplanes, Gerba said, seeing what works on the different kinds of surfaces in an airplane because there are slight differences from surface to surface that can determine which is the right type of product to use.
Moving away from fomites for a brief time, Gerba said that his group has also been looking at interventions to prevent aerosol transmission of the COVID-19 virus. One technology of interest is bipolar ionization, which creates reactive ions in the air and can be used in a facility where people are present. One potential advantage of this technology over filters is that it is not necessary to wait for the air to be recirculated or go through a HEPA filter for it to have an effect. It may also decontaminate surfaces at the same time.
Gerba’s group is also evaluating the use of ultraviolet (UV) light in the 222-nanometer range. Like bipolar ionization, it can be used with people in the room and can be used in real time to kill the virus in the air and potentially on surfaces too.
___________________
11 X. Cao, C.D. Zevitas, J.D. Spengler, B. Coull, E. McNeely, B. Jones, S.M. Loo, P. MacNaughton, and J.G. Allen, 2019, The on-board carbon dioxide concentrations and ventilation performance in passenger cabins of U.S. domestic flights, Indoor and Built Environment 28(6):761–771.
An alternative to the use of sprays or UV radiation is heat inactivation of the COVID-19 virus. One complication is that the effect of heat on the virus depends on the relative humidity. It survives better on surfaces at low relative humidity than at higher relative humidity, which is important because the relative humidity tends to be kept low in aircraft. Gerba said that his group has been looking at temperatures that would be acceptable to passengers on the flight deck, and they have found parameters where heating devices could be used in the flight deck to inactivate the virus.
Ultimately, Gerba said, the goal is to use quantitative microbial risk assessment to evaluate and compare these different interventions, quantifying the potential reduction in risk from each of the technologies and determining which are most effective in which situations. For example, one study looked at the effect that hand sanitizers or disinfectant wipes for desks had in reducing the spread of a virus on the surfaces of an office building, and they showed it was possible to reduce the risk of surface-based transmission by 90 percent by using the right strategies and the right products. By quantifying the effects of the interventions, he concluded, this allows airlines to examine the cost–benefit of various technologies or combinations of technologies and maximize passenger protection.