3
Life-Cycle Assessment in a Low-Carbon Fuel Standard Policy
A low-carbon fuel standard (LCFS) refers to a policy intended to reduce the overall climate forcing that results from the production and use of transportation fuels by incentivizing the use of fuels with reduced emissions intensities. In this chapter, the term LCFS does not refer to any specific existing policy. Individual policies are specified differently; for example, the California LCFS is referred to as CA-LCFS. In general, an LCFS is predicated on three ideas:
- There are multiple energy sources (fuels) for accomplishing the same goal (transportation),
- The production and use of different transportation fuels will result in different climate forcing effects, driven by greenhouse gas (GHG) emissions and other climate forcers, and
- There is a mechanism for increasing the use of fuels with reduced contributions to climate change (referred to as low-carbon fuels) over others by regulating and incentivizing individual fuels based on their estimated ability to reduce GHG emissions and other climate forcing effects relative to an assumed baseline fuel.
In this context, the term “low-carbon” in “low-carbon fuel” refers not to the chemical composition of a fuel itself, but rather to the climate forcing, as estimated for use in the LCFS, associated with the production and use of a fuel relative to the impact of the use of other options. Climate forcing is typically accounted for based on the global warming potential of GHGs including carbon dioxide (CO2), methane (CH4), nitrous oxide (N2O), fluorinated gases, and other climate forcers–some of which may not be GHGs (i.e., aerosols, albedo changes, and other biophysical impacts). Because identical fuel molecules can be produced from different raw materials using different conversion methods, a specific set of material inputs (feedstocks) and conversion processes is referred to in many transportation fuel policies as a “pathway.”
As highlighted in Chapter 2, there is no single life-cycle assessment (LCA) method capable of answering all possible questions related to the climate impacts of a transportation fuel. Attributional LCA (ALCA) and consequential LCA (CLCA) both have important roles to play in understanding the climate impacts of transportation energy use, and each answers fundamentally different sets of questions. For each approach, there are methodological choices that will result in different outcomes. This chapter introduces some of the practical considerations in applying LCA methods to LCFS, both in terms of its direct integration into policy as well as its use in regulatory impact assessment (RIA). It summarizes the use of LCA in LCFS to date and discusses the appropriateness and tradeoffs of different approaches and methods for estimating life-cycle GHG emissions associated with transportation fuels for potential use in a national LCFS. The chapters in Parts II and III of this report explore technology-specific challenges and specific methodological issues in greater depth.
HISTORICAL CONTEXT
The use for LCA in regulating transportation-sector GHG emissions stems from the fact that emissions do not only occur at vehicle tailpipes. Raw material production, conversion to fuel, and the transportation of fuels to consumers can be important contributors to the total carbon footprint. Furthermore, many of those upstream emissions sources are not directly regulated. Regulating only vehicle tailpipe emissions, for example, would unfairly advantage technologies that have upstream impacts but zero tailpipe emissions, such as battery electric or hydrogen fuel cell vehicles. Biofuels were, and continue to be, a focus in the development of LCA methods for LCFS because there are GHG emission sources along biofuel supply chains (e.g., N2O from farmland and fossil CO2 emissions from combustion of diesel fuel
and natural gas in farm equipment and biorefineries). The LCFS concept was also conceived as a way to track and disincentivize the consumption of more emissions-intensive fossil resources.
In 2007, when federal and California policies were beginning to incorporate life-cycle GHG emissions, oil prices were increasing, stimulating increased production of tar sands, oil shale, and consideration of other emissions-intensive alternative production routes, such as coal-to-liquids. Some held the belief that this trend was unlikely to reverse and global “peak oil” (referring to conventional oil production) would soon be reached, implying that prevention of a rise in unconventional fossil fuel production was key to avoiding catastrophic climate change (Kharecha and Hansen, 2008). However, these alternative production routes were not being penalized for their emissions-intensive upstream activities and contributions to the GHG-intensity of transportation. As Farrell et al. noted in their 2007 policy analysis of the CA-LCFS, “[T]he LCFS is a response to this recarbonization of transportation fuels, as well as the many market failures blocking innovation and investments in low-carbon alternatives to petroleum” (Farrell et al., 2007, p. 3). In short, an LCFS attempts to capture both combustion and supply chain emissions by incorporating upstream GHG emissions sources that are otherwise not adequately regulated directly, such as power plants, farms, biorefineries, and hydrogen production facilities. This practice of regulating upstream emission sources can improve the comprehensiveness of an LCFS, but it also introduces additional complexity and uncertainty.
Challenges in the application of LCA for regulatory purposes have long been recognized (Hunt et al., 1996). For example, Lave et al. (1995, p. 420) stated with respect to one LCA method commonly used today (process-based), “Equally credible analyses can produce qualitatively different results, so the results of any particular life-cycle analysis cannot be defended scientifically.” More recently, Ekvall (2019, p. 1), noted:
“[I]t was clear almost from the start that results from different LCAs can contradict each other. This is still true, despite many attempts to harmonize, standardize, and regulate LCA. From history, we learn that it is not realistic to expect LCA to deliver a unique and objective result. It should not be regarded as a single unique method; it is more fruitful to consider it a family of methods.” Meta-analyses and harmonization efforts can adjust for differences in underlying assumptions and methodological choices that drive many of the contradictions across different LCAs, particularly for ALCAs (Heath and Mann, 2012). Despite the complexities of using LCA in a regulatory context, a desire to design policies that achieve GHG emissions reductions has motivated an increase in the use of LCA for policy development, particularly in the context of fuels
LOW-CARBON FUEL POLICIES IN THE UNITED STATES, EUROPE, AND BRAZIL
Although a comprehensive overview of international LCFS is outside the scope of this report, there are several examples of transportation fuel policies across the world that use LCA to varying degrees. This section summarizes a set of policies selected to provide a representative range of approaches to LCA implementation.
In the United States, there have been federal- and state-level policies that aim to reduce GHG emissions from transportation fuels in which LCA has been used. For the United States, the focus is on two groups of policies. The first is the Renewable Fuel Standard (RFS) (75 Fed. Reg. 14863), which was created by the Energy Policy Act of 2005; the life-cycle provisions were added when the program was amended by the Energy Independence and Security Act of 2007 (EISA). The U.S. Environmental Protection Agency (EPA) issued the final rule implementing EISA in 2010. A second set of policies targeting transportation fuels includes the CA-LCFS, which took effect in 2011, and a related policy called the Clean Fuel Program in Oregon, which took effect in 2016. Washington State also passed legislation authorizing a Clean Fuel Standard in 2021, which will go into effect in 2023. The California, Oregon and Washington programs are closely related and for simplicity, this chapter focuses primarily on the CA-LCFS because of its longer history and its similarity to the other more recently-crafted state policies.
Outside of the United States, one policy in the European Union (EU) and another in Brazil are explored. The EU’s 2009 Renewable Energy Directive (RED) and the updated 2018 Renewable Energy Directive (RED II) cover multiple sectors but have specific targets for the use of low-carbon fuels in transportation sector. The RenovaBio policy introduced in Brazil in 2017 sets carbon intensity (CI) targets for road transportation and assigns credits based on individual fuels’ contributions to achieving those targets.
The U. S. Renewable Fuel Standard
The RFS sets threshold GHG emissions reductions compared to a fixed baseline for petroleum-derived fuels that a renewable fuel must achieve to be eligible for the program. In addition to transportation fuels, liquid fuels intended for use as heating oil are also eligible. The legislation contains definitions for what types of feedstocks make a fuel eligible, independent of their GHG footprint. Generally speaking, biofuels, electricity generated using biomass as the fuel, and renewable compressed natural gas (biogas that has been cleaned and upgraded to pipeline quality methane) are currently, or could be, qualifying fuels in the RFS. Notably, the RFS excludes non-biomass sources of renewable electricity, such as solar and wind. Qualifying fuels are then awarded Renewable Identification Numbers (RINs), which serve as the “currency” of the RFS program. There are four categories of RINs, each with different GHG reduction thresholds that fuels must meet according to the specific LCA methods used by EPA:
- Conventional renewable fuel (RIN category D6), 20 percent;
- Advanced biofuel (RIN category D5), 50 percent;
- Biomass-based diesel (RIN category D4); and
- Cellulosic biofuel (RIN categories D3 andr D7), 60 percent
Fuels must be, according to the specific LCA methods used by EPA, 20 percent, 50 percent, 50 percent, and 60 percent less GHG intensive than petroleum-derived fuels, respectively, as assessed in the last year of statutory volumes (2022) (as shown in Figure 9-1, Chapter 9 of this report). The minimum 20 percent GHG reduction for D6 fuels was not strictly required for grandfathered facilities, including biorefineries that were existing, idled, or under-construction as of the November 2009 industry assessment, as well as coal-burning ethanol plants whose construction commenced before December 19, 2007. As of 2010, the EPA calculated that 14.8 billion gallons of ethanol production capacity could be grandfathered in regardless of whether they met the GHG reduction requirement, just short of the total 15 billion gallon cap for corn ethanol (EPA, 2010). There are additional eligibility criteria limiting the feedstocks from which the different fuel categories can be produced, including some safeguards against specific forms of domestic land conversion (EPA, 2010b).
As noted above, the RFS uses a combination of life-cycle emissions estimates and feedstock categorization to assign different alternative fuels to different compliance categories. Therefore, eligibility is based on both the definitions of qualifying fuels set forth in EISA and a fuel’s partial life-cycle GHG emissions relative to the baseline, fossil fuel life-cycle CI determined by EPA. As part of the RFS implementation, EPA has assessed the LCA of a variety of fuel pathways; new fuel pathways may be eligible for the RFS, but producers must first petition and submit data for EPA to conduct an LCA for final approval. EPA (2010) uses a combination of CLCA and ALCA (see Chapter 2) in its assessment of the lifecycle emissions for each fuel pathway. EPA (2010a) assessed the consequential impacts of RFS-induced biofuel demand on agricultural emissions and global land use, but not on fuel markets, for the regulatory impact analysis of the policy, supplemented with attributional emissions to estimate the impacts of biofuel production for four biofuel pathways.1
___________________
1 For a high-level summary of the regulatory impact assessment, see RIAs https://nepis.epa.gov/Exe/ZyPURL.cgi?Dockey=P1006DXP.txt.
An initial RIA (EPA, 2020) is supplemented by additional analysis for new pathways prior to their approval, based on a predominantly attributional analysis based on the energy and emissions associated with feedstock production and conversion. The hybrid ALCA and CLCA-based GHG footprints are used to determine, along with the feedstock type, in which RIN category a given fuel will be placed. RINs are generated when a qualifying fuel is produced and are retired when the fuel is blended. As can be seen above, the conventional renewable fuel (D6) category is the broadest and requires only a 20 percent life-cycle GHG reduction as assessed in 2022, in contrast; the cellulosic biofuel (D3 RIN and, for cellulosic diesel, D7 RIN) requires both a cellulosic feedstock and a 60 percent GHG reduction (although some types of biogas including landfill gas, once upgraded to renewable compressed natural gas, also qualify). EPA annually sets volume requirements based on statutory targets set out in EISA, with periodic adjustments based on various market factors. EPA mandates the blending of an overall quantity of renewable fuel annually, with separate volumetric sub-targets for certain compliance categories. RINs can be traded and carried over time. The four RIN categories are nested, meaning that some types of RINs can be used in compliance for other RINs. For example, biomass-based diesel (D4 RINs) can be used to satisfy advanced biofuel (D5) mandates for advanced biofuels or conventional renewable fuel (D6) for renewable fuels; see Figure 3-1.
Notably, the decision to incorporate CLCA in RFS decision-making was based on guidance in EISA, which included the following definition:
“LIFECYCLE GREENHOUSE GAS EMISSIONS.—The term ‘lifecycle greenhouse gas emissions’ means the aggregate quantity of greenhouse gas emissions (including direct emissions and significant indirect emissions such as significant emissions from land use changes), as determined by the Administrator, related to the full fuel lifecycle, including all stages of fuel and feedstock production and distribution, from feedstock generation or extraction through the distribution and delivery and use of the finished fuel to the ultimate consumer, where the mass values for all greenhouse gases are adjusted to account for their relative global warming potential.”
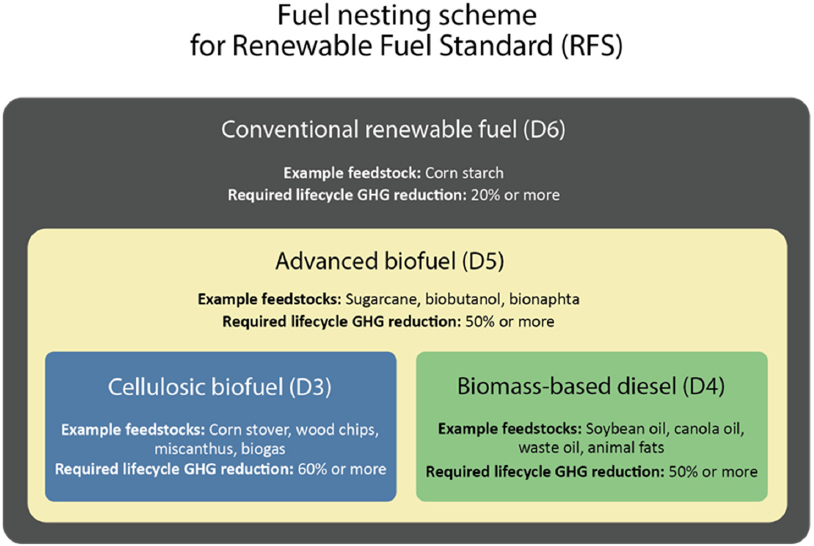
The EPA 2010 final rule acknowledged the uncertainty associated with estimating land use change (LUC) and presented results for emissions from domestic and international LUC as a mean value bracketed by low and high values. Because the RFS uses thresholds for GHG emissions reductions rather than directly tying incentives to CI scores, fuel producers are somewhat insulated from the uncertainty around the impacts of LUC. Once it is demonstrated that a fuel meets this threshold, more precise estimates of the lifecycle GHG emissions of the fuel have no impact on the administration of the policy. Furthermore, while EPA carried out a CLCA including indirect land use change (ILUC, also referred to as market-mediated LUC) for its RIA, it did not ultimately include other market-mediated effects such as potential changes in emissions from changes in fuel markets.
The California Low-Carbon Fuel Standard
The CA-LCFS is a performance-based standard that requires obligated parties—in this case, petroleum refiners and importers—to reduce the CI of the fuel mix intended for road transportation supplied to the road sector, as assessed and approved by the California Air Resources Board (CARB). Amended in 2018 to extend beyond 2020, it mandates a reduction of 20 percent by 2030 of the road sector fuel mix relative to 2010 levels (17 CCR § 95482), using CARB’s accounting methods. Deficits are generated from the production and import of fossil-derived gasoline and diesel fuel; credits may be generated through the use of alternative fuels based on their CI as approved by CARB relative to the benchmark CI assigned to each fuel type, which CARB updates annually. “Opt-in” credits may be generated for pathways such as aviation fuels and electricity, whose producers are not obligated parties under the CA-LCFS. In other words, petroleum-derived aviation fuels do not generate deficits, but alternative aviation fuels with a CI below the baseline jet aviation CI can generate credits.
Unlike the volumetric mandate of the federal RFS, credit generation in the CA-LCFS is based on the quantity of estimated GHG reductions from blended fuel rather than the volumes. Also in contrast with the RFS, non-biomass fuels such as electricity and hydrogen generated from wind and solar can be used for compliance. Similar CI-based standards have been implemented in British Columbia and Oregon and are in the process of implementation in the state of Washington and other provinces in Canada (British Columbia, 2008; Government of Canada, 2020; Oregon DEQ, 2021; Reducing Greenhouse Gas Emissions by Reducing the Carbon Intensity of Transportation Fuel, 2021).2
To be eligible under the CA-LCFS, every fuel producer must develop an LCA for each specific pathway and submit it for certification with CARB. The program includes pathway-specific life-cycle emissions estimates calculated using an ALCA approach, as well as a set of ILUC factors estimated for a selection of crop-based fuels using the Global Trade Analysis Project (GTAP)-BIO model (CARB, 2015). The ALCA portion of the assessment uses a modified version of Argonne National Laboratory’s Greenhouse Gases, Regulated Emissions, and Energy Use in Technologies (GREET) model (referred to as CA-GREET). For biofuel pathways that are well-characterized in the program (e.g., corn ethanol) users may submit a simplified (Tier 1) application that requires inputting facility-specific parameters into a calculator; novel pathways with unique operating considerations require a Tier 2 application that may include a modified CA-GREET model for certification. Pathway applications for new fuels require a combination of validation and verification by third-party verifiers as well as review by CARB.
In contrast with the RFS, the CA-LCFS is performance-based, so the precise CI value assigned to each fuel is integral to the implementation of the policy. The credits a fuel can earn under this policy are proportional to its estimated life-cycle GHG emissions. Accordingly, there is a strong incentive to demonstrate estimated life-cycle GHG emissions that are as low as possible. As noted previously, life-cycle GHG emissions of fuels under the CA-LCFS, excluding ILUC, are generally estimated using ALCA. However, CARB uses a CLCA approach to evaluate GHG emissions of ILUCs.
The policy paper developing the CA-LCFS concept in response to the California governor’s executive order S-01-07 recommended including some non-zero representation of LUC emissions, which
___________________
2 See Washington State’s 2021 Senate Bill 5231 at https://www.washingtonvotes.org/2021-SB-5231.
inherently requires a CLCA approach. However, the executive order acknowledged that these estimates were likely subject to change while methodologies were further developed. The executive order included the following recommendation (California Governor’s Executive Order S-01-07):
“Develop a non-zero estimate of the global warming impact of direct and indirect land use change for crop-based biofuels, and use this value for the first several years of the LCFS implementation. Participate in the development of an internationally accepted methodology for accounting for land use change, and adopt this methodology following an appropriate review.”
Methodologies to quantify the emissions from ILUCs caused by increased biofuels production and use have been developed and refined, as further described in Chapter 9, but no firm consensus methodology has emerged that has been universally adopted by regulators.
The European Union Renewable Energy Directive
The EU’s Renewable Energy Directive (RED) is a broad, cross-sectoral energy mandate with a sub-target for energy supplied to the road and rail sectors (Directive 2009/30/EC). As a directive, it provides high-level targets that shall be implemented with some flexibility by member states. RED II, updated in 2018, mandates a 14 percent transport sector energy target (Directive 2018/2001/EU). There are different GHG reduction requirements for fuels to qualify for RED II, depending on fuel type and what year a facility entered operation; fuels from facilities operating prior to October 2015 must generate at least 50 percent GHG savings as estimated by RED relative to the fossil fuel comparator; fuels from facilities entering operation in 2021 must generate at least 70 percent GHG savings. These emissions reduction thresholds are based only on the GHG emissions estimated with ALCA for each pathway they do not include emissions from ILUCs. Separate from the life-cycle GHG footprint estimates, fuels must comply with a set of sustainability criteria, including a prohibition on biofuels made from crops grown on high carbon stock land converted after 2008 to crop production. To verify that fuels counted toward the RED II are in compliance with the RED II, qualifying third-party verification schemes verify the supply chains of those fuels. These third-party certifiers at a minimum evaluate the feedstock source, land conversion, and life-cycle GHG savings from biofuels, as assessed by RED II, to ensure alignment with the sustainability criteria, and some schemes go further and also assess other environmental and social impacts. These schemes must be approved by the European Commission in order to be recognized.
RED II includes a variety of additional sub-targets, caps, and incentives for specific fuel categories, as well as consideing ILUC elements. The contribution of food-based biofuels is capped at 7 percent of transport sector energy or each member state’s 2020 production, whichever is lower. The cap drops to 3.8 percent in future years. RED II also includes a sub-target of 3.5 percent for advanced biofuels, which includes primarily lignocellulosic feedstocks, wastes, and residues — these fuels may be double-counted towards both the 3.5 percent sub-target and the overall 14 percent transport target. Other fuels, primarily waste oils, fats, and greases, also double count towards the 14 percent target, but their overall contribution is capped at 1.7 percent. The contribution of non-food sustainable aviation fuels and maritime fuels is adjusted to 1.2 times their energy value towards the 14 percent target.
Brazil’s RenovaBio Policy
Brazil’s RenovaBio policy, introduced in 2017, is a CI standard for biofuels (Presidência da República Secretaria-Geral Subchefia para Assuntos Jurídicos, 2017).3 RenovaBio establishes a 10-year time horizon for the reduction in the CI of road transport fuels consumed in Brazil, mandating a 10.2 percent CI reduction from 73.6 gCO2e/MJ to 66.1 gCO2e/MJ (USDA GAIN, 2021). Through the program’s biofuel
___________________
3 See the National Biofuel Policy (Law 13.576/2017 - RenovaBio). https://www.gov.br/mme/pt-br/assuntos/secretarias/petroleo-gas-natural-e-biocombustiveis/renovabio-1/renovabio-ingles.
certification scheme, individual CI scores will be attributed to each biofuel producer or importer based on the CI of fuel supplied. Fuels generate compliance credits on the basis of their CI relative to the fossil fuel baseline, as estimated using the policy’s RenovaCalc LCA model. Biofuel producers must hire inspection firms accredited by the National Petroleum, Natural Gas and Biofuels Agency in order to achieve certification and become eligible to generate compliance credits.
To summarize, the federal RFS and CA-LCFS policies both estimate fuel CIs and include emissions from ILUCs in the calculation of CI for biofuels. The EU RED II does not include those changes in its assessment of GHG savings for specific fuel pathways, but it does include limits on fuels with high emissions from ILUCs. The RenovaBio policy implemented in Brazil has defined CI standards, but it does not include emissions from ILUCs. None of these policies include emissions from changes in fuel markets that arise from changes in fuel production.
CONSIDERATIONS IN APPLYING ATTRIBUTIONAL LIFE-CYCLE ASSESSMENT AND CONSEQUENTIAL LIFE-CYCLE ASSESSMENT IN LOW-CARBON FUEL STANDARDS
Over the years, the use of LCA in the development of the RFS, CA-LCFS, and other policies across the United States and globally have been the subject of much discussion. The charge for this report is to “assess current methods for estimating lifecycle GHG emissions associated with transportation fuels (liquid and non-liquid) for potential use in a national low-carbon fuels program.” This section outlines three different specific applications of LCA in an LCFS and comments on the high-level methodological choices that must be made in each application, namely choosing between ALCA, CLCA, or some combination of both.
The process of estimating life-cycle emissions may be used for several purposes in an LCFS:
- Policy implementation: LCA methods have been used to assign CI scores to individual fuel pathways (production routes). Historically, this process has relied primarily on ALCA methods with a CLCA element added to account for emissions from LUCs, although this is not by any means the only viable approach to developing CI scores.
- Policy design: In addition to the use of CI scores for purposes of calculating credits and deficits used for LCFS policy implementation, LCA methods can also be used to guide other aspects of policy design. For example, the EU’s RED relies heavily on LUC modeling, and the results are used to establish limits and eligibility for particular feedstocks rather than modifying CI scores for a performance-based policy. This approach is meant to promote a policy that does not have market effects that decision-makers deem unacceptable.
- Regulatory impact assessment: Like policy design applications, RIAs are usually undertaken to understand the impacts of implementing or changing a policy and to avoid unintended negative impacts. A RIA would likely ask, among other things, how GHG emissions and other factors will change if the policy in question is enacted (relative to what would happen if the policy is not enacted), so a consequential LCA framing, with appropriate characterization of uncertainty, is well suited for this purpose: see Figure 3-2. The scope of a regulatory impact assessment may be much broader than the methods discussed at length in this report. For example, social LCA may be used to quantify the social and socio-economic impacts of implementing or altering an LCFS.
Of the three low-carbon fuel policies discussed previously, the CA-LCFS serves as a particularly interesting case study in applying LCA to policy. The CA-LCFS is performance-based and uses LCA in policy implementation through its use of CIs. California’s policy defines “life cycle greenhouse gas emissions” as:4
___________________
4 See https://ww2.arb.ca.gov/sites/default/files/2020-07/2020_lcfs_fro_oal-approved_unofficial_06302020.pdf.
“the aggregate quantity of GHG emissions (including direct emissions and significant indirect emissions, such as significant emissions from land use changes), as determined by the Executive Officer, related to the full fuel life cycle, including all stages of fuel and feedstock production and distribution, from feedstock generation or extraction through the distribution and delivery and use of the finished fuel to the ultimate consumer, where the mass values for all greenhouse gases are adjusted to account for their relative global warming potential.”
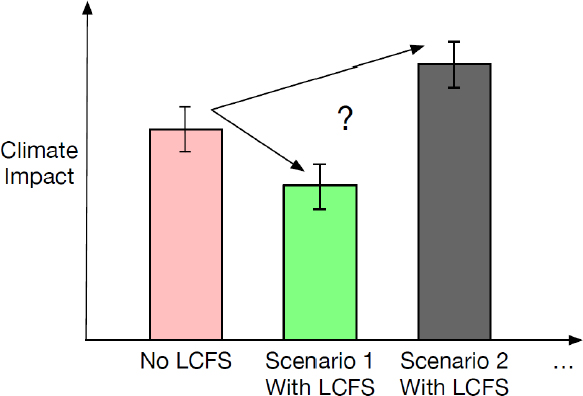
Although this wording does not explicitly require a particular approach, the phrase “related to the full fuel life cycle” has so far been interpreted as requesting a mostly ALCA: the task is to estimate emissions of processes “related to” fuel production and decide which of these emissions to allocate to fuel production and which to attribute to other co-products produced by the supply chain. There are a few caveats worth highlighting: CA-LCFS does assign offset/avoidance credits that are arguably more consistent with a CLCA approach. For example, fuel pathways that use dairy and swine manure as a feedstock for biogas production are awarded emissions avoidance credits relative to a counterfactual in which manure is stored in lagoons that emit methane to the atmosphere (CARB, 2022). It should be noted that these methane avoidance credits are the subject of debate, including a recent petition to exclude dairy and swine manure digesters from the LCFS; in January 2022 CARB declined to take immediate action on this petition but it promised to consider the issue in an LCFS rulemaking process in 2023 or sooner (Corey, 2022). The assessed CI of dairy biogas depends on the assumed counterfactual behavior in the absence of the LCFS; with binding methane regulations in place, it may no longer be appropriate to assume that the methane would have been released into the atmosphere if it were not converted into fuel.5 An additional example of offset credits in the CA-LCFS is the use of a co-product accounting method called system expansion, in which co-products are credited on the basis of the conventional product they displace in their respective markets. For example, dried distillers grains and solids are accounted for by subtracting the impacts of producing a functionally equivalent quantity of protein-containing animal feed (e.g., soybean meal). Similarly, electricity exported from fuel production facilities is accounted for by assigning an emissions offset credit consistent with the average grid mix in that region. Arguably, the application of system expansion to account for electricity exports is consistent with CLCA, but the use of average grid mixes for those credits is consistent with ALCA. CARB does not provide clear guidance for when to use ALCA or CLCA. An overview of ALCA models applied to transportation policies is provided in Box 3-1.
___________________
5 See https://ww2.arb.ca.gov/sites/default/files/classic//fuels/lcfs/lcfs_meetings/041717discussionpaper_livestock.pdf.
In contrast to the ALCA framing relied on in most of the CA-LCFS LCA, ILUC is modeled with a CLCA approach (the word “change” in ILUC itself implies a consequential framing). These analyses estimate how land use will change if a fuel is produced in higher quantities, and then translate that change in land use to a net change in GHG emissions. This type of CLCA, in which market-mediated effects are captured, is not applied for any other element of the analysis underpinning the CA-LCFS. For example, the potential rebound effect associated with increased supply of alternative fuels is not modeled or accounted for (CARB, n.d.).
There are some drawbacks to mixing ALCA and CLCA in the manner that CARB has done in the CA-LCFS. Combining ALCA and CLCA approaches limits the interpretability of the CIs because some parts of the underlying analysis answer one question and other parts answer a different question. In other words, CIs in the CA-LCFS do not reflect the average emissions associated with a fuel nor the emissions implications of changing fuel use, per the modeling methods described by CARB (CARB, n.d.). However, it is worth acknowledging that there are practical reasons why CARB chose not to employ a purely CLCA approach. The average behavior of a system may change relatively slowly (e.g., the electricity grid will not double or halve its carbon footprint in a single year), but the behavior at the margin can evolve more quickly depending on a host of economic, geopolitical, climate, and other factors well outside the control of any entity being directly regulated. Cross-sectoral policy coordination to reduce the chances or understand the implications of policy interaction and the risk of double-booking benefits may be beneficial in these cases. However, these conditions may not always be applicable. Marginal changes in emissions averaged over a range of conditions can also be stable, so incorporating multiple scenarios can help to address this concern.
More broadly, there are challenges associated with the choice to include or exclude market-mediated effects in policy implementation of an LCFS. Some issues with including market-mediated and other consequential emissions in policy implementation for LCFS are that the estimates rely on modeling assumptions that can be difficult to validate empirically and can be sensitive to a wide range of factors such as commodity prices, consumer behavior, market conditions, the presence of other policies and other actors, and future market trends. As noted in the discussion above of CA-LCFS, consequential impacts (occurring on the margin) can vary on a shorter timescale than do most average impacts, so there is a tradeoff between capturing accurate, up-to-date impacts and providing stability and predictability in how an LCFS is implemented.
On the other side, some issues with excluding market-mediated emissions consequences from policy implementation are that ignoring market-mediated effects does not eliminate these effects, nor the uncertainty surrounding them. Regulating fuels based on only a selected portion of each fuel’s emission consequences can create misaligned incentives, favor some fuels over others because of which factors are
excluded, or potentially lead to regulations that intend to reduce GHG emissions as measured by metrics such as CIs but end up increasing total GHG emissions, by some estimates (see Figure 3-3) (Holland et al., 2009, 2015; Lark et al. 2022; Plevin et al., 2017; Rajagopal and Plevin, 2013).
Existing policies have so far addressed the challenges of incorporating market-mediated effects, and the drawbacks of excluding them, by mixing ALCA and CLCA approaches. In an LCFS, the mixing of ALCA and CLCA makes the resulting CI estimates methodologically inconsistent in that they do not fully answer a common question with a common frame. The research community broadly, and members of this committee in particular, have different views about the best path forward for weighing these tradeoffs, particularly between ALCA and CLCA, when designing policy.
CONCLUSIONS AND RECOMMENDATION ON THE USE OF DIFFERENT LIFE-CYCLE ASSESSMENT APPROACHES IN LOW-CARBON FUEL STANDARDS
This committee is in agreement that there is an important distinction between using LCA for policy implementation, policy design, and RIA. In all cases, a rigorous accounting of process-level emissions (e.g., fossil fuel combustion emissions at a refinery, N2O emissions on farms, and CH4 leakage at compressed natural gas production facilities) is essential. Both ALCA and CLCA approaches rely on accounting of direct emissions in foreground systems in this case, each major stage in the primary fuel supply chain, from raw materials through fuel production and distribution. However, in some cases ALCA estimates and allocates average supply chain emissions and CLCA estimates marginal supply chain emissions, the data inputs and results are likely to differ. For example, electricity used as a transportation fuel in a battery electric vehicle may be assigned the average grid mix in an ALCA and the marginal grid mix in a CLCA.
The committee also agrees that any policy proposal intending to reduce GHG emissions should be able to show through a RIA that the likely consequences of enacting that policy will be to reduce total GHG emissions. CLCA is best suited to answer the fundamental question posed in an RIA: What will the net impact of this policy be on GHG emissions compared with a counterfactual in which no policy is implemented? A rigorous RIA is essential, and it is likely necessary to periodically conduct updated RIA to ensure a policy is continuing to have the intended effects on GHG emissions.
In terms of applying LCA approaches to policy design and policy implementation, opinions differ among committee members regarding the appropriate uses of ALCA and CLCA. Some members hold the opinion that ALCA frameworks are appropriate for use in implementation of an LCFS policy via CI scores for each fuel because the use of straightforward GHG accounting, tied to decisions within the control of fuel producers and primary entities in their supply chain, incentivize fuel producers to reduce their own process emissions and select suppliers with lower GHG footprints. In essence, these members recommend the use of ALCA because it allows for relatively more effective externality management by participants in the policy. However, these members are also of the opinion that CLCA analysis may be used to augment ALCA in some instances, but it should also inform policy design by highlighting risks that can be mitigated through other policy mechanisms (including verification; see Chapter 5), policy “guardrails,” and other complementary non-LCFS policies) to manage effects that are not otherwise included in CIs (Khanna et. al., 2017). For impacts that are outside the control of fuel producers and other entities in their primary supply chain, it may be defensible to use “guardrails” and other complementary policy mechanisms to guard against substantial unintended effects. When using multiple interacting policy mechanisms like this, the full committee agrees that such interacting policies should be designed with care to avoid unintended consequences, and the full impacts should be estimated through a RIA.
Other members of the committee are of the opinion that LCFS policy should focus on using CLCA estimates. They argue that it is problematic that ALCA estimates of CIs do not model the policy-relevant factor: the GHG consequences of changing fuel use. These members are concerned about the effects of LCFS policy on competition among fuels: they believe that CIs based on ALCA favor some fuels over others because of modeling boundary choices rather than the GHG emissions consequences of fuel use, creating distorted incentives that, together with other policy factors, can potentially lead to a LCFS policy that increases net GHG emissions (e.g., Holland et al., 2009, 2015; Plevin et al., 2017; Rajagopal and Plevin,
2013). This group is of the opinion that asking how an LCFS affects climate change is a more important aspect of an LCFS than focusing on ALCA-based CIs that may be easier to quantify or verify.
To summarize the key findings in this chapter:
- In existing state LCFS policies, ALCA and CLCA approaches are combined to produce a single CI score. The choice to combine these different approaches was motivated in part, but not exclusively, by a decision to incorporate ILUC GHG emissions into low-carbon fuel policies. Combining results from different LCA modeling approaches in this manner can complicate the interpretation and use of the CI score.
- LCA for RIA is a consequential analysis, estimating how GHG emissions and other factors will change if a policy is enacted. More work is needed to develop national, open-source, transparent CLCA models for use by policymakers and industry experts.
- For policy design, the LCA approach used may depend on a variety of factors including coordination of multiple policy mechanisms, practical issues, limits of regulatory authority, politics, uncertainty, transparency, stakeholder buy-in, avoiding unintended consequences and perverse incentives, and goals and implications beyond GHGs.
- ALCA does not capture market-mediated effects, including the consequences of competition for feedstocks (e.g., electricity, biomass), fuel markets, and land use. ALCA provides insights into choices in the conversion of a feedstock to a fuel pertaining to choice of technology. ALCA may also be used for screening pathways for viability under a particular policy framework.
- Because ALCA and CLCA answer different questions, using both LCA approaches to inform the policy in different ways, together with verification techniques (see Chapter 5), has the potential to strengthen policy design and implementation. As discussed above, there are different views among the committee members on whether or not ALCA and CLCA should be combined in the same analysis.
Conclusion 3-1: The carbon intensities of fuels used in an LCFS are not necessarily equivalent to the full climate consequences of their adoption. Increased use of a fuel with a low carbon intensity, as defined in an LCFS, could potentially decrease or increase carbon emissions relative to the baseline, depending on policy design and other factors. Regulatory impact assessments that use CLCA to project the consequences of policy can help assess the extent to which a given policy design with particular carbon intensity estimates will result in reduced GHG emissions.
Recommendation 3-1: When some emissions consequences of fuel use are excluded from carbon intensity values in an LCFS, the rationale, justification, and implications for these exclusions should be documented.
Conclusion 3-2: More research is needed to evaluate effective methods to collectively leverage the strengths of CLCA, ALCA, and verification methods in achieving LCFS objectives.
Recommendation 3-2: Public policy design based on LCA should ensure through regulatory impact assessment that, at a minimum, the consequential life-cycle impact of the proposed policy is likely to reduce net GHG emissions and increase net benefits to society. Regulatory impact assessments should consider changes in production and use of multiple fuel types (e.g., gasoline, electricity, biofuels, hydrogen).
Recommendation 3-3: LCA practitioners who choose to combine attributional and consequential LCA estimates should transparently document these choices and clearly identify the implications of combining these different types of estimates for the given application, scope and research question.
RECOMMENDATIONS FOR RESEARCH
This chapter concludes with a set of recommendations regarding a research agenda for LCA as it is developed for and applied to fuels. There are many entities that could support and participate in this research. The National Science Foundation supports research to develop and apply LCA methodologies. EPA has developed and applied LCA in transportation fuel policy and is often responsible for developing and implementing policies that use LCA. The U.S. Department of Energy has supported the development and use of LCA for transportation fuels at multiple national laboratories. Finally, because biofuels are a consideration in transportation policy, the U.S. Department of Agriculture, which has a history of supporting and developing biofuel LCAs, is another potential sponsor of LCA research. The Department of Transportation also has a pivotal role in transportation policy and itself implements policies that affect GHG emissions from transportation.
Beyond federal agencies, state environmental and natural resource departments that have or are considering an LCFS may want to support LCA research. Furthermore, non-profit organizations interested in mitigating transportation’s effects on global warming may be interested in providing support. The researchers who carry out this work will likely be a comprehensive network of experts from universities, national laboratories, federal and state agencies, non-profit organizations, and industry. It is critical to obtain diverse perspectives and expertise in carrying out the committee’s recommended research agenda.
Recommendation 3-4: Research programs should be created to advance key theoretical, computational, and modeling needs in LCA, especially as it pertains to the evaluation of transportation fuels. Research needs include:
- Further development of robust methods to evaluate the GHG emissions from development and adoption of low-carbon transportation fuels, and development or integration of process-based, economic input-output, hybrid, and CLCA methodologies
- Products could include the following:
- development of national, open-source, transparent CLCA models for use in LCFS development and assessment
- continued development of national, open-source ALCA models from new or existing models
- evaluation of different approaches to creating, using, or combining ALCA, CLCA, and verification for evaluation of policy outcomes
- quantification of variation between marginal and average GHG emissions for various feedstock-to-fuel pathways; and
- quantification and characterization of the implications of approximations and proxies in LCA, such as comparisons of marginal and average emissions.
Research goals include a comprehensive understanding of the implications of including or excluding various attributional and consequential emissions sources in LCFS CIs on the policy’s resulting overall effect on net emissions and social welfare, as well as identification of opportunities to apply ALCA and/or CLCA to design policies that are well-aligned with their intended outcome.
REFERENCES
Argonne National Laboratory. 2021. Greenhouse gases, Regulated Emissions and Energy use in Transportation (GREET) model. 2021 release. https://greet.es.anl.gov/.
British Columbia. 2008. Renewable and Low Carbon Fuel Requirements Regulation, BC Reg 394/2008. https://www.canlii.org/en/bc/laws/regu/bc-reg-394-2008/latest/bc-reg-394-2008.html.
CARB (California Air Resources Board). 2015. Detailed Analysis for Indirect Land Use Change. https://ww2.arb.ca.gov/sites/default/files/classic/fuels/lcfs/iluc_assessment/iluc_analysis.pdf.
Corey, R. W. 2022. Petition for Rulemaking to Exclude All Fuels Derived from Biomethane from Dairy and Swine Manure from the Low Carbon Fuel Standard Program. https://ww2.arb.ca.gov/sites/default/files/2022-01/LCFS%20Petition%20Response%202021.pdf.
Ekvall, T. 2019. Attributional and consequential life cycle assessment. In Sustainability Assessment at the 21st Century, M. J. Bastante-Ceca, J. L. Luis Fuentes-Bargues, L. Hufnagel, F-C. Mihai, and C. Iatu, eds. London: IntechOpen. https://www.intechopen.com/chapters/69212.
EPA (Environmental Protection Agency). 2010a. Renewable Fuel Standard Program (RFS2) Regulatory Impact Analysis. https://nepis.epa.gov/Exe/ZyPURL.cgi?Dockey=P1006DXP.txt.
EPA. 2010b. Regulation of Fuels and Fuel Additives: Changes to Renewable Fuel Standard Program; Final Rule. 40 CFR Part 80.
EPA. n.d. Renewable Fuel Annual Standards. https://www.epa.gov/renewable-fuel-standard-program/renewable-fuel-annual-standards.
Farrell, A. E., D. Sperling, A. Brandt, A. Eggert, A. Farrell, B. Haya, J. Hughes, B. M. Jenkins, A. D. Jones, D. M. Kammen, C. R. Knittel, M. W. Melaina, M. O’Hare, R. J. Plevin, and D. Sperling. 2007. A Low-Carbon Fuel Standard for California Part 2: Policy Analysis. UC Berkeley: Transportation Sustainability Research Center. https://escholarship.org/uc/item/1hm6k089.
Government of Canada. 2020. Clean Fuel Regulations. https://pollution-waste.canada.ca/environmental-protection-registry/regulations/view?Id=1170.
Heath, G. A. and M. K. Mann. 2012. Background and Reflections on the Life Cycle Assessment Harmonization Project. Journal of Industrial Ecology 16(s1). https://doi.org/10.1111/j.1530-9290.2012.00478.x.
Holland, S. P., J. E. Hughes, and C. R. Knittel. 2009. Greenhouse gas reductions under low carbon fuel standards? American Economic Journal: Economic Policy 1(1):106-146. https://www.aeaweb.org/articles?id=10.1257/pol.1.1.106.
Holland, S. P., J. R. Hughes, C. R. Knittel, and N. C. Parker. 2015. Unintended consequences of carbon policies: Transportation fuels, land-use, emissions, and innovation. The Energy Journal 36. https://www.iaee.org/en/publications/ejarticle.aspx?id=2626.
Hunt, R. G., W. E. Franklin, and R. G. Hunt. 1996. LCA—how it came about. The International Journal of Life Cycle Assessment 1(1):4-7.
Kharecha, P. A., and J. E. Hansen. 2008. Implications of “peak oil” for atmospheric CO2 and climate. Global Biogeochemical Cycles 22. https://doi.org/10.1029/2007GB003142.
Lave, L. B., E. Cobas-Flores, C. T. Hendrickson, and F. McMichael. 1995. Life cycle assessment: Using input-output analysis to estimate economy-wide discharges. Environmental Science and Technology 29:420A–426A.
Lark, T. J., N. P. Hendricks, A. Smith, N. Pates, S. A. Spawn-Lee, M. Bougie, E. G. Booth, C. J. Kucharik, and H. K. Gibbs. 2022. Environmental outcomes of the US Renewable Fuel Standard. Proceedings of the National Academy of Sciences 119(9). https://doi.org/10.1073/pnas.2101084119.
Oregon DEQ. 2020. Oregon Clean Fuels Program. https://www.oregon.gov/deq/ghgp/cfp/Pages/default.aspx.
Plevin, R. J., M. A. Delucchi, and M. A. O’Hare. 2017. Fuel carbon intensity standards may not mitigate climate change. Energy Policy 105:93-97. https://doi.org/10.1016/j.enpol.2017.02.037.
Rajagopal, D., and R. J. Plevin. 2013. Implications of market-mediated emissions and uncertainty for biofuel policies. Energy Policy 56:75-82. https://doi.org/10.1016/j.enpol.2012.09.076.
U.S. Department of Agriculture. n.d. USDA Global Agricultural Information Network (GAIN) Database. Greenhouse Gas Reduction (Renewable and Low Carbon Fuel Requirements) Act. https://gain.fas.usda.gov/#/home.