Summary
One essential component of maintaining a safe, stable climate is a global transition to a net-zero carbon dioxide (CO2) emissions system, with no new net accumulation of CO2 in the atmosphere. In 2019, CO2 emissions in the United States totaled 5.26 gigatonnes (Gt), out of a total of 33.4 Gt CO2 emissions globally. Among the highest priority actions to mitigate these emissions are decarbonizing the electric grid and transitioning energy end uses to decarbonized electricity, which will eliminate the majority of sources of emissions from fossil-fuel combustion. This long-term transition to net-zero emissions likely will eliminate the largest current uses of carbon-based products and processes, such as gasoline or diesel fuel for light-duty transportation and natural gas for heating in residential and commercial buildings. Mitigation of the remaining CO2 emissions will occur via a number of different strategies, collectively termed carbon management. Large volumes of CO2 will require long-term removal from the atmosphere, for instance, by storage in geologic formations underground or by sequestration in durable, long-lived products such as concrete, aggregates, some polymers, and nanostructured forms of carbon, such as carbon fiber. Short-lived carbon-based products, including fuels for some heavy-duty transportation applications, some polymers, and commodity chemicals, still will be needed and will have to be produced and used in a circular manner with no net emissions on a life cycle basis. Currently, the vast majority of embodied carbon in chemicals and materials (84 percent globally in 2020) is fossil derived, but in a circular carbon economy, this fossil carbon will need to be replaced with sustainable carbon from waste plastics and other carbon-based products, biomass-based carbon feedstocks, and CO2. This report focuses on CO2 utilization, in which CO2 captured from flue gas streams, the atmosphere, or bodies of water is chemically transformed into a marketable product. The positioning of CO2 utilization within the larger carbon management objective is illustrated in Figure S-1.
In the Energy Act of 2020, the U.S. Congress mandated that the U.S. Department of Energy (DOE) contract with the National Academies of Sciences, Engineering, and Medicine to analyze opportunities and challenges to advance CO2 utilization technologies, develop the associated infrastructure, and establish markets for CO2-derived products, considering a future in which carbon wastes participate in a circular carbon economy. For this first of two reports from the study, the committee was tasked with assessing the state of the infrastructure for CO2 transportation, use, and storage as of early 2022 and identifying priority opportunities to improve and expand on that infrastructure to enable future CO2 utilization development. The following paragraphs summarize the committee’s approach to the study and its main findings, conclusions, and recommendations.
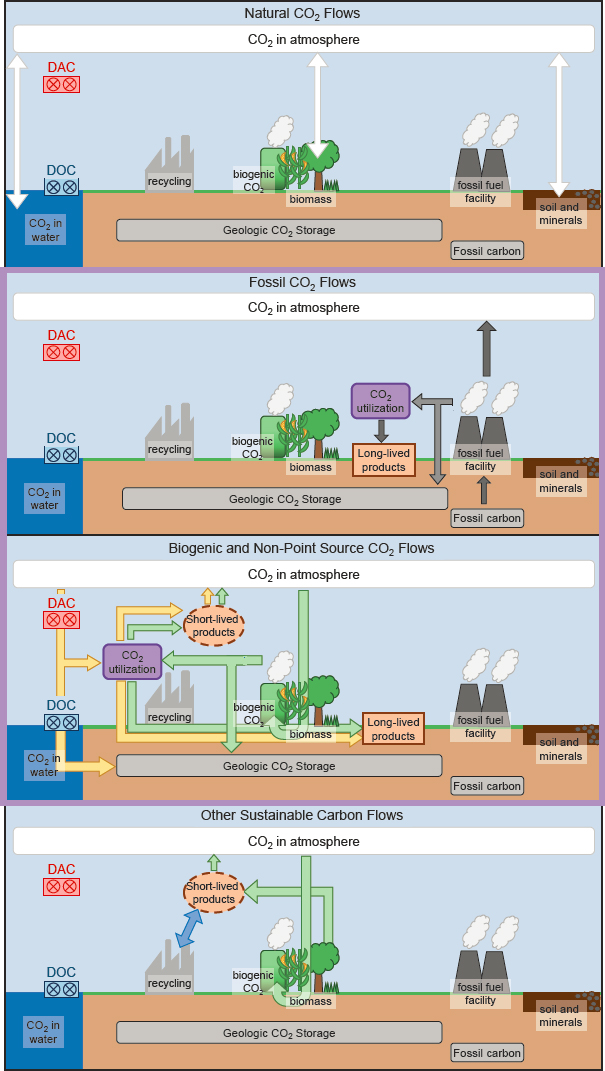
NOTE: DAC = direct air capture; DOC = direct ocean capture.
The committee first identified existing infrastructure for CO2 capture, transport, conversion, and geologic storage, as well as infrastructure for enablers of CO2 utilization processes such as clean electricity, clean hydrogen, water, land use, and energy storage. The largest volume chemical utilization of CO2 today is in urea synthesis; other products made on an industrial scale from CO2 include organic carbonates, methanol, salicylic acid, and inorganic carbonates embedded in concrete. Pilot-scale efforts are under way to generate additional products from CO2; however, the inherently higher energy requirements to use CO2 as a feedstock in place of fossil carbon for hydrocarbon-based products and the lack of incentives to produce net-zero-emission products currently limit commercialization opportunities for CO2 utilization. Furthermore, the committee found limited opportunities to leverage current CO2 capture and transport infrastructure for future CO2 utilization projects, since the majority of the existing infrastructure has been developed for enhanced oil recovery (EOR) and connects geologic or fossil-based sources of CO2 with depleted oil reservoirs, routes that generally do not align with sustainable CO2 utilization opportunities in a net-zero-emissions future. Nonetheless, the infrastructure expected to be developed for CO2 capture and geologic storage (CCS) over the next decade also may be able to serve utilization projects. Therefore, the committee recommends that CCS developers design flexibility into their project plans to allow some of the CO2 captured to be directed to utilization facilities (Recommendation 6.2). Given that many CO2 utilization processes require significant amounts of clean electricity, clean hydrogen, and water, project developers also will need to consider the availability and accessibility of these inputs when designing CO2 utilization infrastructure (Recommendations 4.4, 4.5, 4.6, and 6.3). DOE similarly should consider the availability and accessibility of geologic CO2 storage, CO2 transportation and product offtake infrastructure, clean electricity, and water when evaluating the design and siting of direct air capture and hydrogen hubs authorized in the Infrastructure Investment and Jobs Act (Recommendations 6.3 and 6.4).
To assess opportunities and challenges for future CO2 utilization infrastructure development, the committee identified priority products that could be synthesized from CO2 in a future circular carbon economy, separating these products into two classes, or “tracks,” distinguished by their lifetime, and hence their ability to sequester carbon for long periods, as illustrated in Figure S-2. Track 1 products, with lifetimes greater than 100 years,1 include concrete, aggregates, some polymers, and solid carbon materials that durably store CO2. Track 2 products, with lifetimes less than 100 years, include chemicals, some polymers, and fuels that decay to CO2 and re-emit it to the atmosphere on relatively short timescales, unable to store carbon for long periods. Importantly, these Track 1 and Track 2 products have different requirements for sustainable production consistent with net-zero-emissions goals. Track 1 products derived from non-fossil CO2 sources, such as direct air capture or biogenic carbon, are net-negative-emissions compatible, while those derived from fossil CO2 point sources are net-zero-emissions compatible. On the other hand, Track 2 products must be derived from non-fossil CO2 sources to be net-zero-emissions compatible. In addition to considering the CO2 source, assessments of net-negative- or net-zero-emissions compatibility require taking into account emissions associated with all other inputs (e.g., electricity, hydrogen, and heat), processes, product fates, and any co-products or waste generated. Such sustainability considerations influence the type and location of infrastructure, as discussed further below.
When developing infrastructure for CO2 utilization, the entire value chain of CO2 capture, purification, transportation, and conversion needs to be considered. Commercial technologies exist for purifying CO2 to achieve the different requirements for capture, transport, utilization, and storage, but add cost to CO2 utilization processes. Mineralization and biological CO2 conversion are the most resilient to impurities of the CO2 feedstock, and catalytic electro- and thermochemical CO2 conversion are the most sensitive. Once captured (and purified, if required), CO2 can be transported by a variety of means including pipeline, rail, truck, ship, and barge. In many cases, some combination of these transportation methods will be required. Multimodal transport infrastructure will be particularly beneficial for connecting small-scale emitters and capturers located far from main pipelines with larger industrial hubs containing utilization facilities, and for connecting large- or small-scale emitters and capturers with distributed small-scale users (e.g., concrete manufacturers). When accessible, CO2 pipelines are the most cost-effective transportation option, as they can move the largest volumes of CO2 and therefore benefit from
___________________
1 The 100-year lifetime is chosen as the cutoff between Track 1 and Track 2 products to align with the United Nations Framework Convention on Climate Change.
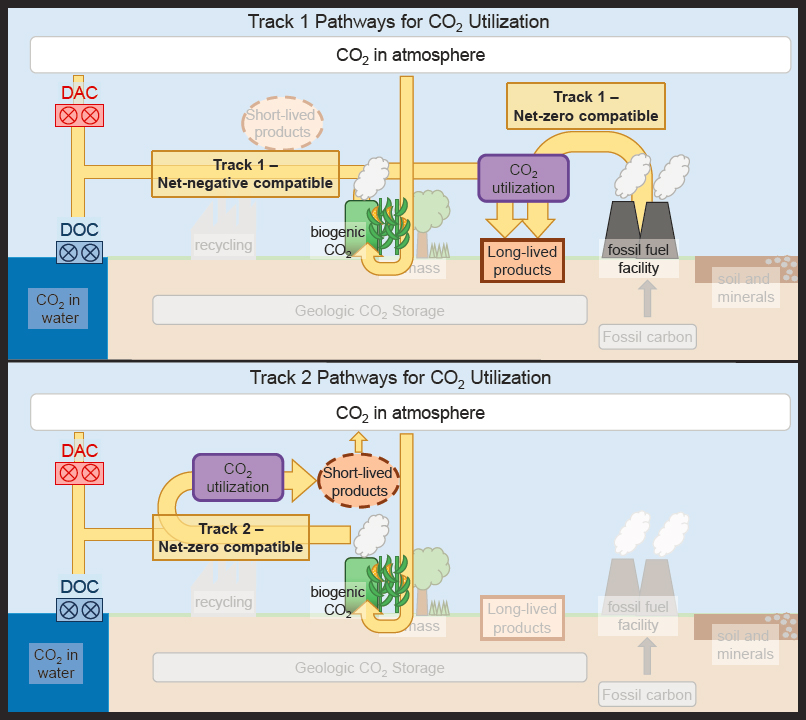
NOTE: DAC = direct air capture; DOC = direct ocean capture.
economies of scale. Of paramount importance in any transportation scenario is safety; the primary risks from CO2 are physical damage due to rupture of pressurized equipment and asphyxiation. There is a considerable level of understanding regarding the risks associated with pipeline transportation of CO2, both dense-phase and gaseous. One option under consideration for CO2 transport is to repurpose natural gas pipelines, which could simplify acquisition of rights-of-way and potentially decrease capital costs. However, pipeline integrity and operational issues make this challenging. Each individual project would require rigorous techno-economic and cost-benefit analyses to determine its feasibility.
Infrastructure development decisions for CO2 utilization depend not only on CO2 capture, purification, and transportation requirements but also on the availability of enabling infrastructure for inputs such as clean electricity, clean hydrogen, and water and for transportation of the CO2-derived product. Many CO2 utilization processes require significant amounts of clean electricity and clean hydrogen. Increases in demand for zero-carbon-emissions electricity, including that required for CO2 utilization, will influence transmission and distribution planning and load management. In the long term, post-2050, it is expected that the grid will need to accommodate all power demand with a reliable supply of net-zero-emissions electricity for all users, but in the short term, CO2 utilization processes may require deployment of clean power generation and energy storage to enable the 24/7 operation likely required for economic viability. Given the complexities of transporting and storing hydrogen, the committee
recommends that project designers co-locate facilities for hydrogen production and CO2 utilization when feasible (Recommendation 4.5). Another required input for some CO2 utilization processes is water. Though not expected to have a significant impact on water demand at a national scale, water requirements for CO2 utilization could put stress on local water resources. Water will be an input to biological and chemical utilization processes, as a reaction medium, a reactant, or for process cooling. The committee therefore recommends siting CO2 utilization facilities near a sustainable water source to support both short- and long-term needs and analyzing the effect of CO2 utilization and the required enabling inputs (e.g., hydrogen) on local water demands, taking into consideration local environmental and justice impacts (Recommendation 4.6). Some CO2 utilization processes, such as concrete production and biological CO2 conversion to alcohols and hydrocarbons, are well suited for small-scale, distributed operations, with co-located CO2 capture and conversion followed by transport of the CO2-derived product. Optimizing the CO2 transportation network to meet the needs of different utilization processes requires a systems-level analysis considering technological, environmental, economic, and societal factors (Recommendation 6.5).
In addition to developing technology and infrastructure, enabling and expanding CO2 utilization requires consideration of policies, regulations, equity, and environmental justice. The most effective and efficient policies to enable CO2 utilization would ensure that externalities from greenhouse gas emissions from all sources are captured in the full cost of using the technology and incentivize knowledge creation and reduce costs and risks for early adopters rather than subsidize specific technologies. In all cases, policies should support the CO2 utilization industry toward societal goals, such as net-zero emissions, in a technology-agnostic manner. Policy makers should also take care that the policy regime does not create perverse incentives or excessively difficult regulatory environments (Recommendation 5.1). Establishing policies that overly incentivize CO2 capture, for example, may create an unwanted incentive to produce emissions. Navigating the requirements to obtain permits from many different entities or managing the uncertainty in policy stability over the long term may decrease or deter investments in CO2 utilization. The committee recommends that a single entity be responsible for guiding CO2 utilization project developers through the permitting process, given the large number of organizations involved in granting permissions (Recommendation 5.4). Finally, the committee recognizes that widespread deployment of CO2 utilization may have significant environmental, economic, and societal impacts. Total costs and benefits to society and their distribution should be assessed for each individual project using cost-benefit analyses. In particular, the equity and justice implications of CO2 utilization projects should be examined and addressed. Furthermore, CO2 utilization projects should involve early and sustained community engagement and address inequality, which may include terminating a project that does not receive local buy-in or for which regulators determine that the equity implications are unacceptable (Recommendation 5.6).
Given all of the above considerations, the committee identified two primary near-term opportunities for CO2 utilization infrastructure investment: (1) using CO2 sourced from bioethanol plants to make sustainable synthetic aviation fuel and (2) mineralization using fossil or non-fossil CO2 sources to generate aggregates for construction materials, including concrete. In both cases, locating the CO2 utilization operation in close proximity to enabling infrastructure (e.g., clean electricity and clean hydrogen) could prove cost-effective. While the committee deemed these two opportunities to be priorities in the near term given current knowledge and technologies and their potential scale of deployment, they are by no means the only promising CO2 utilization opportunities. Consequently, and recognizing that infrastructure to capture and transport CO2 for storage will likely be developed over the next decade, the committee recommends designing flexibility into that infrastructure such that it could be used to meet longer-term CO2 utilization needs (Recommendation 6.2). One example would be developing industrial clusters that co-locate capture, utilization, and storage of CO2 and incorporate relevant industries such as hydrogen production, chemical and fuel manufacturing, and low-carbon power generation. Specifically, the committee recommends that the Department of Energy consider co-locating at least one each of the hydrogen and direct air capture hubs authorized in the Infrastructure Investment and Jobs Act of 2021 (Recommendation 6.4). The best deployment and investment opportunities for CO2 utilization should be identified using techno-economic, life cycle, and integrated systems analyses, while considering relevant regulatory and policy frameworks and factors that may influence societal acceptance via cost-benefit analyses (Recommendation 6.1). All infrastructure siting and development decisions should include best practices for substantive community engagement to ensure equitable outcomes.
Net-zero or net-negative CO2 utilization can support the decarbonization transition by providing pathways for sustainable synthesis of carbon-based chemicals and materials in products, and carbon storage in durable, Track 1 products. However, few commercial examples of companies making net-zero or net-negative products via CO2 utilization exist today, and for CO2 utilization to meet its potential will require overcoming limitations and challenges related to technologies, processes, infrastructure, and business opportunities. These limitations and challenges include inherent inefficiencies that result in high costs; needs for discovery of and improvement in processes and technologies; requirements for large amounts of additional inputs, such as land, hydrogen, water, and electricity; elimination of the emissions associated with the entire life cycle of a CO2 utilization process and product; limited existing CO2 infrastructure; and societal concerns about infrastructure deployment, especially from environmental justice communities. Considering these challenges and opportunities, this report evaluates near-term needs for CO2 utilization to play a role in a net-zero future.