3
Large-Scale Interactions Among the Upper Ocean, Atmosphere, and Land
SPATIAL VARIABILITY
Tropics
It is generally accepted that the tropical atmosphere is primarily thermally driven by the latent heat released in deep convective clouds. A glance at any monthly or annually averaged precipitation chart (for example, Figure 3-1) indicates that these convective clouds tend to be clustered together in large agglomerations, which are referred to here as ''heat sources.''
There are three major localized heat sources: one is centered over the Amazon basin; one is over tropical Africa; and the largest one is over the "maritime continent," which comprises Indonesia, Malaysia, the surrounding islands, and adjacent oceanic regions. In addition to these localized heat sources, there are also the somewhat rectilinear-shaped sources characteristic of the Intertropical Convergence Zones (ITCZs) in each ocean, the northwest-to-southeast-oriented South Pacific Convergence Zone (SPCZ), and the similarly oriented South Atlantic Convergence Zone (SACZ).
The three major localized heat sources move seasonally, generally being most north and west in northern summer, and south and east in southern summer. The ITCZs are generally north of the equator, most northward in late northern fall and most equatorward in northern spring. The Amazon basin and African heat sources are
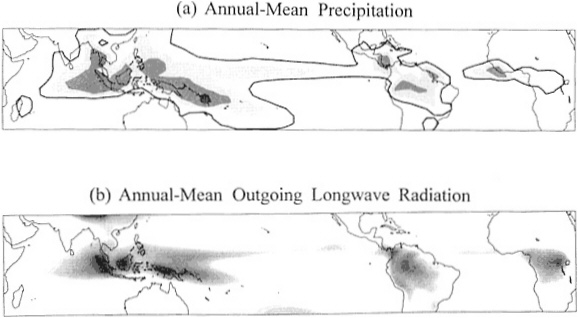
Figure 3-1 (a) Annual-mean precipitation over the tropics, after Jaeger (1976). The heavy contour encloses regions with amounts in excess of 12 cm per month; and light, intermediate, and dark shading correspond to amounts in excess of 16, 20, and 30 cm per month, respectively. (b) Annual-mean outgoing longwave radiation for the period 1974–1991. Values below 240 Wm-2, indicated by the shading, are associated with regions of deep convective clouds.
primarily over land. The maritime-continent heat source responds strongly to SST changes. Indeed, it is the interannual motion of this heat source that can be identified with the ENSO phenomenon. During warm phases of ENSO, the maritime-continent heat source expands eastward into the anomalously warm tropical Pacific; during cold phases, it contracts back into the warm western Pacific. During a warm phase of ENSO, while the maritime-continent heat source is in its easternmost position, anomalously high rainfalls occur over the central Pacific and anomalously low rainfalls over Australia, Indonesia, and the Indian subcontinent. During the cold phases of ENSO, the opposite occurs.
It is known that the major heat sources interact. The problem of drought over the northeastern region of Brazil, for example, has features that have been correlated with the location of the ITCZ and SST anomalies in the Atlantic as well as the SST anomalies in the Pacific (Hastenrath, 1991, secs. 8.6 and 9.7). During the warm phases of ENSO in the Pacific, anomalies observed in the upper-level winds over the Atlantic can only be explained by anomalous modulations of the Amazon-basin heat source or long-range effects of the maritime-continent heat source. It is also known that the maritime-continent heat source is influenced by processes in and around the Indian Ocean as well as by SST changes in the tropical Pacific (Barnett, 1983, 1984a,b). The convergence of moisture into this maritime-continent heat source is partly due to the mean easterlies over the Pacific and partly due to the mean westerlies over the Indian Ocean.
The progress in understanding atmosphere-ocean interactions in the tropical Pacific can probably be attributed to:
-
The relative unimportance of land, which makes the maritime-continent heat source respond primarily to SST anomalies;
-
The tight coupling between SST and the regions of persistent precipitation over the Pacific (on monthly time scales the regions of persistent precipitation tend to follow the warmest water); and
-
The essentially linear nature of equatorial thermocline adjustment in the Pacific, making the problem of non-locally induced SST variability comparatively simple.
While the thermocline adjustment problem has similar dynamics in other tropical oceans, the tight relationship between heating and SST in the Pacific does not necessarily hold more generally. For example, April is the warmest month for SST in the northern Indian Ocean, but

Figure 3-2 Climatological mean sea-surface temperature (SST), surface winds, and outgoing longwave radiation (OLR) over the Indian Ocean for the months of April and July. Contour interval of 1K for SST; OLR values below 240 Wm-2, indicated by the shading, are associated with regions of enhanced deep convective clouds. SST and surface winds after Sadler et al. (1987).
it is not the time of maximum precipitation (see Figure 3-2). The time of maximum precipitation there is during the summer monsoons, when the northwest extension of the maritime-continent heat source lies over Southeast Asia and extends into the Bay of Bengal. There is clear influence of the land bordering the Indian Ocean during the northern summer (Webster, 1987). Indeed, the large amount of rainfall over the Indian subcontinent can be associated with the extension of the maritime-continent heat source into this region due to the effects of land. Thus, the motion of the maritime-continent heat source in the tropical Pacific due to SST variations in the tropical Pacific is only part of the puzzle. In general, climate variations in the tropics can be viewed as modulations and movements of the tropical heat sources, each affected by its underlying ocean, by combinations of ocean and land, or by land alone, and by interactions with other heat sources. To understand fully the motion and associated wind and precipitation patterns of the maritime-continent heat source, it is necessary to understand the influence of the conditions in and around the Indian Ocean on the convergence patterns of moisture, and to understand the interactions of the other heat sources with the maritime-continent heat source.
It is clear that thermal forcing in the tropics must be considered as a whole. In modeling the tropical thermal forcing, all the heat sources and sinks need to be included. The consequence of those heat sources that lie over the ocean (the maritime-continent heat source, ITCZs, SPCZ, and SACZ) will be determined primarily by atmosphere-ocean processes and will be directly affected by local evaporation, although modification will occur in some relation to their proximity to land. Over land (the Amazon Basin and African heat sources), the nature of the vegetation and soil moisture determine the local evaporation and surface temperature. It should be emphasized that when all the sources and sinks of tropical thermal forcing and their variability are taken into consideration, these land processes must also be considered.
Tropical-Extratropical Interactions
The interactions between the tropics and the extratropics occur over a wide range of spatial and temporal scales. It is believed that, in general, the tropics affect the midlatitudes on slower time scales (monthly or longer), while the midlatitudes influence the tropics on faster time scales (synoptic to monthly). On the "intermediate" time scale (a month or two), neither the tropics nor the extratropics dominates the mutual interaction. On these intermediate time scales the interac-
tions between the tropics and extratropics are the most complex, representing an interplay between the chaotic midlatitude flow and the more regular tropical flow (see, for example, N.-C. Lau et al., 1992; Molteni et al., 1993). There is clear evidence that tropical variations affect higher latitudes on interannual time scales (Horel and Wallace, 1981; Ropelewski and Halpert, 1987, 1989), but the mechanisms for this influence are still in question.
The Hadley circulation (the zonally averaged atmospheric meridional circulation) is driven by the zonally averaged tropical heating. It extends from inside the deep tropics all the way to the subtropics and is essentially responsible for the existence and maintenance of the subtropical jet (Schneider, 1977).
In addition to the midlatitude response of zonally symmetric heating in the tropics, teleconnection patterns forced by zonally asymmetric tropical heating sources are also an important source of midlatitude variability (Hoskins and Karoly, 1981; Branstator, 1985). The teleconnection pattern depends not only on the distribution of tropical heating but also on the three-dimensional distribution of the basic state climatological flow. Because the basic flow may be dependent on the tropical forcing and transients may play a role in the forced teleconnection patterns, the influence of the tropics on the midlatitudes may involve many different factors and may be very complex. In spite of these difficulties, the influence of the tropics on the midlatitudes has been demonstrated by numerical model experiments that show that the more realistic the simulation of tropical heat sources, the more realistic the simulation of midlatitude circulation.
The western boundary ocean currents (the Gulf Stream, for example) bring heat and salt from the tropics into midlatitudes and, as extensions of the boundary currents, into higher latitudes. Both the Hadley circulation and the western boundary currents can influence the middle and higher latitudes on time scales of seasons to a year or more. Eastern boundary Kelvin waves are also known to affect the higher latitudes on the time scales of interest to the GOALS program. For example, during warm phases of ENSO, the water off the western coast of the United States is usually warm and is a northward extension of the warm event taking place in the equatorial Pacific.
The influence of the midlatitudes on the tropics is most pronounced during the northern winter season and occurs on synoptic to intraseasonal time scales. The aggregate of these effects may significantly influence the evolution on longer time scales. One of the most noted examples of the midlatitudes influencing the tropics is the occurrence of cold surges associated with the Northern Hemisphere winter mon-
soon. Cold surges are midlatitude baroclinic disturbances that form over the east coast of China, over Japan, and in the East China Sea. They are often accompanied by strong surface northeasterlies that penetrate the equatorial regions. In addition, prolonged periods of high-frequency surface westerly wind fluctuations in the warm-pool region of the western Pacific have been linked, in part, to the excitation of equatorially trapped wave modes by lateral forcing from the extratropics—in particular, cold surges from the east Asian monsoon (K.-M. Lau et al., 1989). These westerly wind bursts and their accompanying rainfall are known to have significant impact on the salinity changes and, hence, on the dynamic and thermodynamic structure of the upper ocean on daily-to-weekly time scales.
The Extratropics
Due to large day-to-day atmospheric variability in the extratropics, possible influences of boundary forcings are difficult to discern in the winter season. However, variations in the land-surface conditions (soil moisture, vegetation, and so forth) can produce significant changes in the summer season's extratropical atmospheric circulation and monthly averaged temperatures over land (Shukla and Mintz, 1982; Manabe and Wetherald, 1987). The nature and degree of this interaction between the land surface and the atmosphere depend on the character of the dynamical circulation regime where land-surface changes are taking place. It is expected that the specification of initial land-surface conditions can lead to enhanced predictability during summer seasons. The land's influence depends on the interaction of the soil moisture and vegetation of the land surface with the overlying circulation. A sufficiently accurate treatment of atmosphere-land processes therefore provides a potential for prediction of seasonal mean temperatures, and possibly of rainfall, over continental midlatitude regions.
A large number of sensitivity studies using GCMs have shown that the simulation and prediction of the monthly and seasonal surface temperature and rainfall over land and those of the mean diurnal cycle are quite sensitive to the specification of the initial land moisture (Cook and Gnanadesikan, 1991; Fennessy and Shukla, 1992). Adequate initialization and assimilation procedures to specify the initial land-surface properties, including soil moisture and snow cover, and realistic parameterizations of energy and momentum exchanges between the land surface and atmosphere need to be developed for this potential predictability to be fully understood and utilized.
Finally, the question arises as to whether anomalies in midlatitude
SST on seasonal-to-interannual time scales affect the midlatitude atmosphere. Here the evidence is less conclusive. According to Wallace and Jiang (1987) and Wallace et al. (1990), there is no question about there being strong correlations between midlatitude SST anomalies and midlatitude atmospheric anomaly patterns. These papers point out that the simultaneous correlations between midlatitude atmospheric anomalies and midlatitude SST anomalies are even stronger than the simultaneous correlations between midlatitude atmospheric anomalies and tropical SST anomalies. The strong correlations are between the most dominant mode of SST variability and the most dominant mode of atmospheric variability for both the midlatitude Atlantic and the Pacific. The modeling studies of Lau and Nath (1990), using a 30-year record of observed global SST to force a low-resolution atmospheric GCM, found similar patterns of correlations.
For strong simultaneous correlations, one would naturally assume that there is a strong coupling between the midlatitude SSTs and the midlatitude atmosphere. The lag correlations indicate, however, that the correlation is much stronger when the atmosphere leads the ocean than when the ocean leads the atmosphere, indicating that in large part the atmosphere is forcing the ocean (Hasselmann, 1976). The existence of predictability of the coupled midlatitude atmosphere-ocean system needs to be much more intensively studied.
TEMPORAL VARIABILITY
Climate variability has been documented on all time scales, from a few tens of days to the age of our planet. The GOALS program would concentrate on the seasonal-to-interannual band. The following discussion concentrates on the temporal variability of climate and its relation to the seasonal-to-interannual band.
Subseasonal Variability
Short-term climate variability is often obscured by fluctuations on the subseasonal time scale. Of particular importance in this regard are the eastward-propagating planetary waves with periods on the order of 40 to 60 days, which modulate convection and perturb the surface wind field over the tropical Indian and western Pacific sectors (Madden and Julian, 1971). These planetary waves influence the timing of the onsets and cessations of the rainy seasons over much of Australasia, and they appear to be responsible for at least some of the well-defined breaks between extended episodes of monsoon rainfall. Their signature is evident in the thermal structure of the upper
ocean all the way across the equatorial Pacific, as illustrated in Figure 3-3. At times it is difficult to determine whether large month-to-month changes in the various indicators of the state of the coupled tropical atmosphere-ocean system over the Pacific sector are signaling a major swing of the ENSO cycle or whether they are merely responding to the passage of one of these waves. It is conceivable that at certain critical junctures in the ENSO cycle, the passage of a wave might have important implications for the evolution of the coupled system over the course of the next few seasons. The extratropical circulation also exhibits large month-to-month variability which appears to be associated with teleconnections related to the 40-to-60-day wave (Weickmann et al., 1985) and the 20-day wave (Branstator, 1987; Kushnir, 1987).
Higher-frequency variability is also of interest in GOALS, to the extent that it mediates processes that determine the mean state and the evolution of short-term climate variability. In certain situations, analysis of the high-frequency variability can yield important insights into physical processes that operate across the full range of time scales. For example, Figure 3-4 shows time series of surface wind speed, insolation, and subsurface temperature at one of the TOGA TAO moorings over a 10-day period. It is evident that on the days when the winds were relatively strong, the diurnal cycle penetrated downward to at least 30 meters, with nearly neutral stratification during the nighttime hours. However, during the 3-day period of relatively weak winds, the diurnal cycle was confined to the topmost few meters; below 10 meters the water column was stratified throughout the 3-day period. By examining such sequences, it is possible to make inferences concerning the role of wind-driven entrainment in maintaining the nearly neutral stratification of the mixed layer and the time scale over which the ocean equilibrates to changes in the atmospheric forcing.
The diurnal cycle, which was evident in the preceding example, also plays a critical role in mediating vertical mixing and deep cumulus convection over land. Examples of other high-frequency phenomena of interest in GOALS are 20-to-30-day period tropical instability waves along the equatorial front in the eastern Pacific Ocean, which produce strong meridional transports of heat and zonal momentum; and 4-to-5-day period easterly waves in the atmosphere, which modulate the intensity of rainfall along the Atlantic and Pacific ITCZs.
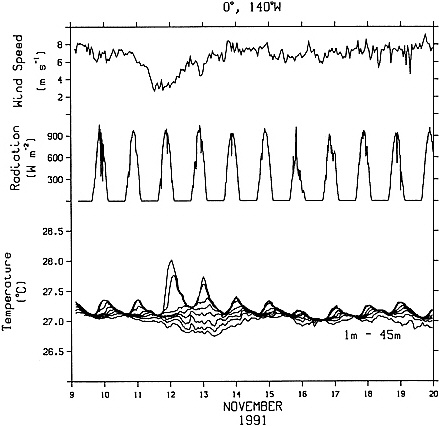
Figure 3-4 Time series of surface wind speed, insolation, and subsurface temperature at the TOGA TAO mooring on the equator at 140°W over an 11-day period. The subsurface time series are for depths of (proceeding from top to bottom) 1, 3, 10, 17, 24, 30, 36, and 45 m.
The Annual Cycle
The short-term variability that is the focus of GOALS occurs against a background signal of enormous importance, the annual cycle. An understanding of and ability to predict the interannual fluctuations that are of interest to GOALS depend on the ability to simulate and explain the annual cycle. The circulation changes associated with short-term climate variability represent relatively small distortions in the climatological mean annual cycle. For example, a modest shift in the wintertime planetary-wave configuration can bring below-
normal temperatures and more frequent snowstorms to an area the size of the northeastern United States. A small displacement of the convection associated with the summer monsoon can bring floods or droughts to the semi-arid regions that lie along the margins of the monsoon rain belts. Subtle changes in summertime circulation patterns can also shift hurricane tracks. To predict the anomaly patterns as they evolve from one season to the next, it is necessary to understand and be able to model the annual cycle in the coupled ocean–atmosphere–land system.
Although the annual cycle is global, the processes that determine its character vary considerably from region to region. Therefore, a study of this cycle can conveniently be subdivided into components that focus on different regions. For example, the annual cycle of the eastern tropical Pacific is strikingly different from that of the Indian Ocean; each can be studied separately before turning to the links between them. The eastern tropical Pacific has a marked asymmetry relative to the equator; the warmest surface-waters and the mean position of the ITCZ are north of the equator. Interactions between the ocean and atmosphere are of prime importance in this region, and processes involving the land play a secondary role. In the Indian Ocean, on the other hand, the monsoons are strongly dependent on land processes.
The annual cycle has many other manifestations throughout the globe. In the equatorial Pacific, the cycle is more clearly evident in SST at the far eastern end, while it is evident in large monsoonal changes in winds and small changes in SST in the western warm-pool. Over all oceans it is manifested in the annual progression of SST, and over land in the annual progression of air temperature, sea-level pressure, vegetation amount, and soil moisture.
Gross features of the climatological mean annual cycle include, for example, the rainy seasons over the tropical continents that occur around the time of the equinoxes along the equator, shifting to midsummer as one moves away from the equator. Many such features can be understood as a direct response to the annual cycle in insolation, but some of the other features are no less difficult to explain than the ENSO cycle. For example, the observed meridional shifts in the ITCZs, which account for the single pronounced rainy season around March over much of equatorial South America, appear to be a consequence of complex interactions involving upwelling in the equatorial cold tongues, vertical mixing and evaporation, changes in the overlying stratus clouds, and changes in surface winds induced by the continental monsoons. Another example is the suddenness of the onsets of the climatological mean rainy seasons in some areas,
TABLE 3-1 Climatological mean precipitation (in cm) for selected stations, showing the onset of the rainy season.
STATION |
|
APR |
MAY |
JUNE |
JULY |
AUG |
SEPT |
OCT |
Tucson |
(32°N, 111°W) |
0.8 |
0.4 |
0.6 |
6.1 |
5.4 |
3.4 |
2.2 |
Mazatlan |
(23°N, 106°W) |
0.1 |
0.2 |
3.4 |
16.3 |
20.1 |
21.6 |
6.5 |
Bombay |
(19°N, 73°E) |
0.0 |
1.8 |
60.1 |
93.5 |
61.3 |
34.4 |
10.4 |
which would be difficult to account for on the basis of the slowly varying insolation associated with the annual cycle. (See Table 3-1.)
The annual cycle modulates the amplitude, structure, and month-to-month persistence of temperature and circulation anomalies throughout the atmosphere and upper ocean, including those associated with the ENSO cycle. Pronounced seasonality is also evident in the predictability of these anomalies, as explained in more detail in Chapter 4. Indeed, it is difficult to conceive of a well-designed study of short-term climate variability that is not framed within the context of the annual cycle.
Interannual Variability
The ENSO cycle is the dominant mode of interannual variability in and over the tropical Pacific; understanding it and being able to predict it served as the focus of the TOGA program. It is now recognized that the ENSO cycle is due to interactions between the atmosphere and the upper ocean. The accompanying slow evolution of SST anomalies in the eastern Pacific is due to an atmosphere–ocean instability modulated by the effects of slowly propagating equatorial ocean waves and equilibrated by nonlinear processes in the atmosphere and ocean. ENSO manifests itself through the expansion of warm SSTs and heavy rainfall eastward into the Pacific, thereby modulating the annual cycle in the eastern part of the basin.
Throughout the ENSO cycle, there exist higher-frequency modulations due to short time-scale variability, particularly in convection, and due to 40-to-60-day waves. The ENSO cycle is in turn perhaps affected by longer time-scale processes, particularly in the ocean. The mean state of the upper ocean is dominated by the existence of a thermocline that is maintained by the slow (time scale on the order of hundreds of years) thermohaline circulation of the ocean.
Important interannual variability exists in other regions of the globe. In the Atlantic, the annual cycle dominates the SST variations, but small interannual changes in the location of the ITCZ affect the
rainfall over northeast Brazil (Hastenrath and Heller, 1977). Over the North Pacific, strong interannual variability of SST and atmospheric circulation has been correlated with the ENSO cycle in the tropical Pacific (Horel and Wallace, 1981). Considerable interannual variability exists at midlatitudes in surface temperature, pressure, and precipitation (see, for example, Walsh and Mostek, 1980); in snow cover and soil moisture (for example, Walsh et al., 1985; Karl et al., 1993); and at higher latitudes in snowcover and sea ice (Chapman and Walsh, 1993). Over continents, soil moisture increases the time scale of atmospheric variability and leads to interannual variability of summer rainfall (Delworth and Manabe, 1989). Understanding this interannual variability and how much of it is predictable is one of the major objectives of GOALS.
Other manifestations of interannual variability include a tendency for phase locking between ENSO and the annual cycle resulting in an enhanced biennial variability (Rasmusson et al., 1990; Ropelewski et al., 1992), which shows up mainly as a biennially varying low-level circulation over the western Pacific and eastern Indian oceans. The possibility that the alternation of strong and weak Asian monsoons is related to interaction of the atmosphere with the surrounding oceanic regions is suggested by lag correlations between SST and monsoon rainfall (Yasunari, 1990). Observations of biennial variability of the Mindanao Current have been presented by Lukas (1988).
Decadal Variability
Interannual and interdecadal climate variations are not always clearly separable, even in retrospect, because of the absence of a spectral gap in the frequency domain. For example, warm episodes of the ENSO cycle are usually marked by cyclonic circulation anomalies over the central North Pacific, which induce negative SST anomalies in that region. Anomalies of that polarity were set up in association with the 1976–1977 warm episode, and they persisted throughout most of the 1980s. Warm wintertime surface air temperature anomalies downstream over western Canada that occurred in association with the same atmospheric teleconnection pattern contributed to the observed warmth of the Northern Hemisphere during the 1980s relative to the previous three decades (Trenberth, 1990; Trenberth and Hurrell, 1994). It is not clear whether this long-lived ''regime shift'' is rooted in the ENSO cycle itself or whether it is of extratropical origin (Trenberth, 1990). This example serves to illustrate how the assessment of interdecadal variability in the state of the climate system requires judgment as to whether the observed climate changes over the past few years should
be interpreted as manifestations of interannual or longer-term variability.
A number of the important signals in the climate record exhibit variability on both interannual and interdecadal time scales. Examples include rainfall over areas such as the Sahel (Folland et al., 1986) and the U.S. Great Plains, hurricane and typhoon frequency and tracks, salinity anomalies over the North Atlantic (Dickson et al., 1988), and variations in sea-ice extent (Walsh and Johnson, 1979). Investigations of the interannual component of these signals, conducted within the context of the GOALS program, will contribute to the understanding of some of the processes that operate on longer time scales. Such processes include desertification, variability of the eastern and western boundary currents, the gyre circulations and their associated poleward heat fluxes, and relationships between the atmospheric circulation, sea-ice extent, salinity, and the production of bottom water in the marginal ice zones.