2
Low-Dose Radiation Exposures and Health Effects
This chapter addresses the first charge of the Statement of Task, which calls for defining the health and safety issues that need to be guided by an improved understanding of low-dose and low-dose-rate radiation health effects. To address this charge, the committee identified seven low-dose exposure sources relevant to members of the U.S. population. These are natural radiation sources, medical applications, occupational exposures, nuclear power routine operations and accidents, nuclear or radiological incidents, exposures from the nuclear weapons program, and nuclear waste. These sources are important in terms of low-dose and low-dose-rate exposures either because they contribute a large portion to the average annual dose to the U.S. general population or to specific populations (e.g., medical applications), or they are of concern to members of the public and impacted communities (e.g., nuclear waste management) or to current and future U.S. policies and plans (e.g., nuclear power operations). The relative contribution of these sources is displayed in Figure 2.1.
The seven low-dose exposure sources described in this chapter involve different types of ionizing radiation (e.g., alpha, beta, and gamma radiation), routes of exposure (internal and external), and durations of exposure (acute or protracted), as summarized in Table 2.1. For example, the type of radiation, route of exposure, and dose rate that are involved in releases from routine nuclear power plant operations are different from those from a medical computed tomography (CT) scan; therefore, the biological consequences of these two exposure sources may differ. Some members of the public may receive combined exposures from these different sources, both internally and externally, and over differing periods of time.
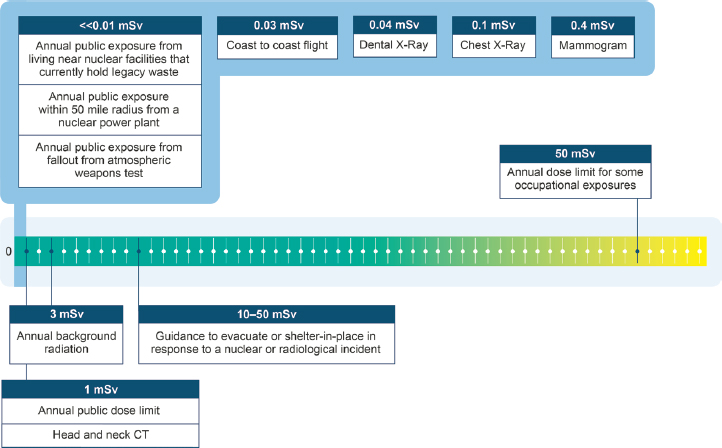
NOTE: CT = computed tomography; mSv = millisievert.
TABLE 2.1 Exposures to the General U.S. Population, Communities, Patients, or Workers, Following Different Exposure Sources
Exposure Source (exposed population) | Approximate Size of Exposed Population | Approximate Annual Effective Dose Today | Route of Exposurea |
---|---|---|---|
Natural Sources (general U.S. population) | |||
Terrestrial | Entire U.S. population | 2.49 mSvb | Internal (inhalation, ingestion, absorption) and external |
Today, in the absence of a mechanistic understanding of different low-dose and low-dose-rate radiation sources and their direct health effects, estimation of risks and decisions related to exposures from substantially different sources often are made using the same generic approach—by relying on risks derived from higher, acute, external exposures to radiation, largely from the Japanese atomic bombing survivors, and applying appropriate correction factors in risk models.
In Section 2.2, the committee discusses current epidemiological evidence on health effects that are associated with low doses of radiation and identifies several knowledge gaps, particularly for health outcomes other than cancer, that are now being observed at lower doses than in the past.
2.1 LOW-DOSE RADIATION SOURCES AND EXPOSURES
The following sections illustrate common low-dose radiation sources and exposures to the U.S. population. The committee does not attempt to provide a complete review of the issues involved with these sources and exposures, but instead it uses some key references to the existing literature to indicate the current state of knowledge.
Types of Radiationa | Duration of Exposure (acute or protracted) | Typical Radioisotopes of Exposurea (not exhaustive list) | Radiation Protection and/or Policy Implications of New Low-Dose Radiation Information |
---|---|---|---|
Alpha, gamma | Protracted | Potassium-40; uranium-238 and -234 and the decay products of uranium, such as thorium-232, -230, and -228, radium-224 and -226, and radon-220 and -222 | Decisions about housing and remediation; government radon mitigation programs; informing dose calculators for members of the public; decisions about air travel, space travel, and need for monitoring |
Protons, neutrons, higher Z elements | Protracted | — |
Exposure Source (exposed population) | Approximate Size of Exposed Population | Approximate Annual Effective Dose Today | Route of Exposurea |
---|---|---|---|
Diagnostic Medical Exposures (patients) | |||
CT | 74 millionc | 1.4–1.5 mSvc | External |
Radiography and Fluoroscopy | 271 millionc | 0.22 mSvc | External or internal (absorption) |
Cardiac interventional fluoroscopy | 3.6–4.1 millionc | 0.13 mSvc | External or internal (absorption) |
Noncardiac interventional fluoroscopy | 4 millionc | 0.13 mSvc | External |
Nuclear medicine | 13.5 millionc | 0.32 mSvc | Internal (injection, inhalation, ingestion, absorption) |
Occupational Exposures | |||
Medical personnel, commercial nuclear workers, DOE facilities; industrial radiographers; mining and milling; other | 3.86 milliond | 0.6–3.1 mSvd | External, internal (inhalation, ingestion, and absorption) |
Aircrew | 170,000d | 3 mSvd | External |
Types of Radiationa | Duration of Exposure (acute or protracted) | Typical Radioisotopes of Exposurea (not exhaustive list) | Radiation Protection and/or Policy Implications of New Low-Dose Radiation Information |
---|---|---|---|
X-ray | Acute | — | Benefit-risk balance for a procedure; need for regulation of patient doses or tracking of exposures; communications |
X-ray | Acute | — | |
X-ray | Acute | — | |
X-ray | Acute | — | |
Alpha, beta, gamma | Acute | Technetium-99; iodine-123, -125, -129, and -131; xenon-133; iridium-192; actinium-225; astatine-211; fluorine-18; gallium-67; yttrium-90; radium-223, -224, and -225; ruthenium-106; lutetium-177 | |
X-ray; alpha, beta, neutron, and gamma | Protracted | Potassium-40; uranium-238, -235, and -234; and the decay products of uranium, such as thorium-232, -230, and -228; radium-224 and -226; and radon-220 and -222 | Revision of exposure limits for radiation workers; informing dose calculators for aircrews; setting exposure limits for aircrew; radiation compensation policies; communications |
Galactic cosmic; solar particle events | Acute or protracted | — |
Exposure Source (exposed population) | Approximate Size of Exposed Population | Approximate Annual Effective Dose Today | Route of Exposurea |
---|---|---|---|
Nuclear Power Under Routine Operations (communities in vicinity) | 1 million within 5 miles; more than 45 million within 30e miles | <<0.01 mSv | Internal (inhalation, ingestion) and external |
Nuclear or Radiological Incident (impacted communities) | Varies depending on incident ranging from a few people to millions of people | Varies depending on incident | Internal (inhalation, ingestion, and absorption) and external |
Nuclear Weapons Program (1942–1991; impacted communities) | All areas of the United States received fallout but larger amounts over some parts of Utah, Colorado, Idaho, Nevada, and Montanag | <<0.01 mSv | Internal (inhalation, ingestion, and absorption) and external |
Types of Radiationa | Duration of Exposure (acute or protracted) | Typical Radioisotopes of Exposurea (not exhaustive list) | Radiation Protection and/or Policy Implications of New Low-Dose Radiation Information |
---|---|---|---|
Alpha, beta, gamma | Protracted | Carbon-14; cobalt-60; tritium; iodine-129 and -131; krypton-85; xenon-135; cesium-137 | Acceptability of nuclear power and current and new reactor technologies; decommissioning; implementation of permanent solution for nuclear waste storage and disposal; emergency planning zoning; communications |
Alpha, beta, gamma | Acute and protracted depending on radiological incident | Incident-dependent but could involve americium-241; cesium-134 and -137; cobalt-60; iodine-129 and -131; plutonium-238, -239, and -240; polonium-210; strontium-90; uranium fission products; activation productsf | Appropriate protective actions; remediation and reoccupation activities; communications |
Alpha, beta, neutron, gamma | Acute and protracted | Uranium-238, -235, and -234; plutonium-238, -239, and -240; iodine-129 and -131; americium-241; strontium-90; tritium; cesium-137; carbon-14; fission products; activation products | Dose reconstruction; radiation compensation policies; communications |
Exposure Source (exposed population) | Approximate Size of Exposed Population | Approximate Annual Effective Dose Today | Route of Exposurea |
---|---|---|---|
DOE Nuclear Waste Sites (communities in vicinity)h | 3.8 millioni | <<0.01 mSv | Internal (inhalation, ingestion, and absorption) and external |
a Some information from https://www.epa.gov/radiation/radionuclides.
b See https://www.epa.gov/radiation/radiation-sources-and-doses.
f See https://www.nrc.gov/docs/ML0531/ML053130250.pdf.
g See https://www.cancer.gov/about-cancer/causes-prevention/risk/radiation/stateandcountyexposure.
h See U.S. NRC (2012).
i Based on population sizes in counties where the remaining 16 active sites are located.
NOTE: CT = computed tomography; DOE = Department of Energy; mSv = millisievert.
2.1.1 Natural Sources
The exposure of humans to radiation from natural sources is unavoidable. For the U.S. population, approximately half of the average annual dose (about 3 millisieverts [mSv]) is due to external exposure from terrestrial gamma and cosmic radiation and approximately half is due to internal exposure by inhalation of radon progeny and ingestion of radionuclides.1 Radiation exposure from natural sources varies globally and within a country depending on the geology and altitude where people live. For example, in the United States, background radiation (excluding radon) can range from less than 0.1 mSv in low-elevation towns along the Gulf Coast to about 2 mSv in high-elevation towns in the Colorado Plateau.2 Areas around the world where the annual
___________________
1 Ingestion of radionuclides such as radioactive potassium-40 and radium-226 (present in plants and animals) or uranium and thorium (present in water) contribute to a dose of about 0.3 mSv to the average person in the United States.
2 See https://www.epa.gov/radiation/calculate-your-radiation-dose.
Types of Radiationa | Duration of Exposure (acute or protracted) | Typical Radioisotopes of Exposurea (not exhaustive list) | Radiation Protection and/or Policy Implications of New Low-Dose Radiation Information |
---|---|---|---|
Alpha, beta, neutron, gamma | Protracted | Cesium-137; strontium-90; technetium-99; tritium; plutonium-238, -239, -240; curium-242; fission products; activation products (nickel-63) | Cleanup activities; communications |
effective dose to the populations due to background radiation is above 20 mSv are considered high background areas (Hendry et al., 2009).
High Background Areas
Studies of the health of populations living in areas with high levels of background radiation conducted during the past 25 years are a potential source of information on the effects of low-dose protracted exposures that are also relevant to the U.S. population. These include descriptive studies in Guarapari (Brazil), Kerala (India), Ramsar (Iran), and Yangjiang (China), where levels of background radiation are high due to high concentrations of radioactive minerals in the soil. Overall, these studies have not found any associations between background radiation and cancer risk or other health problems arising from these high background radiation levels (Dobrzyński et al., 2015; Nair et al., 2009; Tao et al., 2012) although one study found that a higher level of background radiation was associated with a higher
incidence of chromosomal aberrations (Hayata et al., 2004). These studies of high background areas have several design limitations including lack of individual estimates of doses to specific organs from internal and external exposures, lack of consideration of high-risk populations (e.g., children), which would increase statistical power to detect health effects, and lack of well-documented health statistics, in particular, organ-specific cancer rates (Hendry et al., 2009). Better designed nationwide registry-based studies of background radiation in the United Kingdom, France, Germany, and Switzerland have resulted in conflicting results with some showing an association of childhood leukemia and gamma radiation exposure (Kendall et al., 2013; Mazzei-Abba et al., 2021)3 and others not showing an association (Berlivet et al., 2020; Spix et al., 2017). The German study found an association with incidence of central nervous system tumors in exploratory analyses (Spix et al., 2017). These studies also lacked information on children’s individual exposure to radiation from natural and other sources. Participants of an international scientific workshop aimed to evaluate how epidemiological studies of background radiation and childhood cancer can best improve current understanding of the effects of low-dose protracted radiation. They determined that in the absence of individual cumulative doses, it is difficult to draw firm conclusions about risks. (For a summary of the discussions of the workshop, see Mazzei-Abba et al., 2020.)
Residential Radon
Initial information regarding cancer risks from radon came from observations following occupational exposures of underground miners in the 1950s and 1960s (Holaday and Doyle, 1964), when the average concentrations of radon progeny in uranium mines were high—approximately 92,000 becquerels per cubic meter (Bq/m3) or approximately 2,500 picocuries per liter (pCi/L) due to poorly ventilated underground mines. These studies, conducted in Germany (Kreuzer et al., 2018), France (Rage et al., 2015), Canada (Navaranjan et al., 2016), Czech Republic (Tomásek, 2012), and the United States (Samet et al., 1991; Schubauer-Berigan et al., 2009), consistently showed a positive dose-response relationship between occupational exposure to radon decay products and lung cancer risk. These studies also demonstrated that radon and cigarette smoking have a synergistic effect on lung cancer rates, so that smokers are at higher risk from radon exposure (Grosche et al., 2006). Although early in the debate about radon-related risks it was questioned whether findings from high occupational exposures to radon could be used to understand risks from lower exposures
___________________
3 These studies found a 4–12 percent excess relative risk of childhood leukemia per millisievert of cumulative red bone marrow dose from gamma radiation.
to radon in the home, studies on residential radon exposures (Cheng et al., 2021; Darby et al., 2005; Field et al., 2000; Krewski et al., 2006; Lorenzo-Gonzalez et al., 2019; Turner et al., 2011) provided consistent evidence that radon exposure presents an important environmental health hazard.
The Environmental Protection Agency (EPA) estimates that the average indoor radon concentration for homes is about 1.3 pCi/L, and nearly 1 out of every 15 homes in the United States is estimated to have an elevated radon level (4 pCi/L or more; EPA, 2018a); that is, more than 7 million houses need mitigation. Of those only about 2 million had radon mitigation systems installed, implying that about 5 million houses still have high indoor radon concentrations.4 Based on risk models developed by the National Research Council’s Biological Effects of Ionizing Radiation VI committee (NRC, 1999a), EPA estimates that radon is responsible for about 21,000 lung cancer deaths every year, making it the second-leading cause of lung cancer after smoking (EPA, 2003). Several programs and initiatives carried out by EPA and partners aim to reduce radon-induced lung cancer deaths by mitigating elevated indoor radon levels and adopting radon-resistant construction techniques.5
The effects of exposure to the low levels of radon decay products and health outcomes other than cancer are not well understood. One study of residential exposure suggested an association of radon with stroke (Kim et al., 2020). The Pooled Uranium Miner Analysis (PUMA) study is anticipated to provide additional insights on exposure to low levels of radon decay products and health outcomes other than cancer. PUMA is the largest study of uranium miners conducted to date that combines information from cohorts of uranium miners from North America and Europe. PUMA was designed to identify health effects of contemporary uranium miners employed in settings with mechanical ventilation where average concentrations are typically held below the range 500–1,500 Bq/m3 (Rage et al., 2020). Comparisons of mortality rates in the PUMA cohort with those of the general population showed elevated risks for cancers of the lung, stomach, larynx, liver, and gallbladder but not for cardiovascular disease (Richardson et al., 2021). Analyses on the exposure-response relationships for different disease outcomes are not yet available.
Rare Earth Element and Lithium Mining
New natural radiation exposure sources are becoming of concern for populations in the United States, notably the possible pollutants or
___________________
4 See https://cfpub.epa.gov/roe/indicator.cfm?i=27. The cost for radon mitigation depends on the size, layout, and foundation of the house. An average price is more than $1,000.
contaminants that could result from rare earth element (REE)6 and lithium7 mining. REEs have become increasingly important resources for modern technological applications including those that support clean energy transition such as cellular telephones, computer hard drives, electric and hybrid vehicles, flat-screen monitors and televisions, and permanent magnets used for motors and wind farm operations. Similarly, demand for lithium, a key component of batteries used in hybrid and electric cars, is also increasing (Graham, 2021). Availability of REEs and lithium is a high priority for green energy and long-term energy policies in the United States (Tracy, 2020).
China had been the major supplier of REEs until supply issues related to export restrictions arose in the past decade (Tracy, 2020), leading the United States and other countries to explore alternative economic REE deposits. The Mountain Pass deposit in California has produced most of the REEs mined in the United States since the late 1960s and resumed mine production in 2017 following a period of inactivity. Other potential domestic resources for REEs are located in Alaska, Nebraska, Texas, and Wyoming (Tracy, 2020). For lithium, roughly 70 percent of the world’s deposits are located in salt flat regions in Chile, Bolivia, and Argentina. Chemetall in Nevada is currently practicing lithium extraction from brine, and Salton Sea in California is being explored as a possible lithium mining resource. Despite the benefits of mining REEs and lithium in support of clean energy transition, under normal operations, these mining activities may have adverse social and environmental impacts similar to other mining activities related to thorium present in REE deposits and chemicals used in the extraction process (Cheminfo Services Inc., 2012) which results in technologically enhanced naturally occurring radioactive material (TENORM wastes,8 see Section 2.1.3). These mining activities also raise concerns related to contamination of groundwater and drinking water with radionuclides, during both normal operations and accidents (Cone, 1997; Penn and Lipton, 2021).
Space Radiation
Space radiation consists of high-energy charged particles of extraterrestrial origin that constantly bombard Earth and contribute a natural source of background radiation (about 0.4 mSv annually). Some of these particles come from the sun (solar particle events [SPEs]), but most are from deep
___________________
6 There are 17 REEs, most of which have unique magnetic and optical properties and are not rare, as the name implies. These are lanthanum, cerium, praseodymium, neodymium, promethium, samarium, europium, gadolinium, dysprosium, holmium, terbium, thulium, ytterbium, lutetium, scandium, and yttrium.
7 Lithium is a naturally occurring metal found in pegmatitic minerals, ocean water, and some brines and clays.
8 See https://www.epa.gov/radiation/tenorm-rare-earths-mining-wastes.
space (galactic cosmic radiation [GCR]). Earth’s atmosphere and magnetic shield protect against cosmic radiation, and only a low dose of low-energy ionizing radiation eventually makes it to sea level. Exposure to space radiation from SPEs becomes more relevant to airplane passengers, especially at higher altitudes and latitudes. Long-distance air travel can lead to low radiation exposures—about 0.03 mSv for a roundtrip airplane flight from New York to Los Angeles. Most people do not fly frequently enough to be exposed to high levels of radiation from space, but aircrew and frequent flyers could add a significant amount to their total radiation dose because of how often they fly. (For more information on aircrew exposures, see Section 2.1.3.) There is currently no radiation protection guidance for members of the public who are frequent flyers.
Space tourism (i.e., space travel for recreational or leisure purposes) became a reality in 2021 with three commercial companies commencing suborbital flights (Howell, 2021). Space travel is likely to expand in the current year and beyond, with additional suborbital flights and trips to the International Space Station initially and potential future space tourism experiences with destinations such as the moon, planets, and asteroids (Bushnell, 2020). The Federal Aviation Administration estimates that the risk to crewmembers on suborbital commercial spaceflights due to radiation exposure is comparable to that of air-carrier aircrews (average 3 mSv per year; NCRP, 2009a) but likely lower until space tourism flights become frequent and have durations similar to those of commercial aviation. There is currently no radiation protection guidance for members of the public who engage in space tourism.
2.1.2 Medical Exposures
Medical procedures that use ionizing radiation such as CT diagnostic examinations, nuclear medicine, and fluoroscopy-guided intervention have revolutionized medicine and improved medical decision-making and patient care. Their use contributes to diagnosis of disease and injury, informs appropriate care, and often allows patients to avoid more invasive procedures (Rubin, 2014). Between the 1980s and 2006, the average exposure of the U.S. population to radiation from medical diagnostic procedures increased sevenfold, primarily due to increased use of CT and nuclear medicine (NCRP, 2009a), making it the biggest human-made source for radiation exposure to the U.S. population. That increase in exposure plateaued in the last decade (NCRP, 2019), but exposure from medical diagnostic procedures remains the biggest human-made radiation source, contributing an estimated dose to the U.S. population of 3 mSv. Today, CT diagnostic procedures account for about 60 percent of the dose received by the U.S. population from medical diagnostic procedures (see Figure 2.2), with about 74 million CT procedures taking place in 2016 (Mettler et al., 2020).
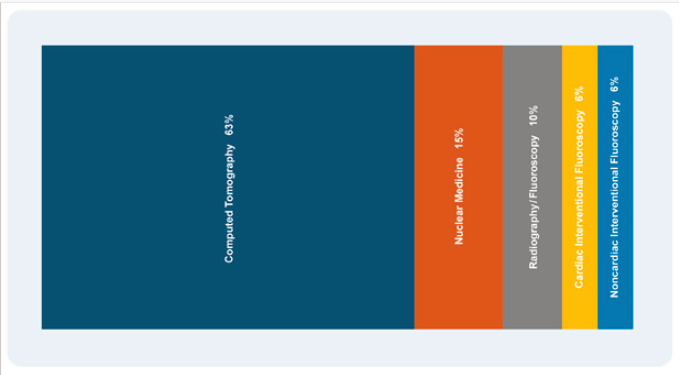
Effective radiation doses have been estimated for a number of typical diagnostic medical procedures, and they can range from about 1 mSv for a head and neck CT to 9 mSv for cardiac CT (NCRP, 2019). Although a single medical diagnostic procedure is within the low-dose range, some patients undergo multiple procedures, and their cumulative dose can exceed the low-dose range. Using data from multiple institutions in the United States, Rehani et al. (2020) found that more than 1 percent of patients were exposed to cumulative effective doses above 100 mSv, with a median cumulative effective dose of 130 mSv and a maximum effective dose of about 1,200 mSv. Patients who received these cumulative doses were typically older (>50 years of age), but a significant fraction (about 20 percent) was younger (Rehani et al., 2020). According to a different study, 80 percent of CT examinations in patients receiving cumulative effective doses above 100 mSv were related to evaluation or follow-up of a malignancy (Jeukens et al., 2021). Globally, it was estimated that 1 million patients per year are exposed to cumulative effective doses of more than 100 mSv due to medical diagnostic procedures (Brambilla et al., 2020).
Effective doses from diagnostic X-rays are much lower than from CT or nuclear medicine procedures, on the order of 0.1 mSv for a chest X-ray, and in the case of mammography on the order of 0.4 mSv (NCRP, 2019). Radiation therapy is another important medical use of radiation that benefits treatment of about half of the patients with cancer (Tyldesley et al., 2011). The intent of radiation therapy is to destroy cancer cells, and therefore the doses and dose rates utilized are much higher—on the order of hundreds or thousands of times higher than in the diagnostic procedures. Radiation
therapy becomes relevant to the issues discussed in this report because of the lower doses to tissues outside the treatment field, some within the low-dose range (Lee et al., 2014).
A recent review noted that “the recurrent application of medical imaging procedures involving ionizing radiation are of concern, from the viewpoint of radiological protection” (Rühm et al., 2022). With the exception of mammography, radiation doses to patients are not regulated and limits do not apply. There are two guiding principles for the safe use of radiation in medicine: justification and optimization (ICRP, 1996). For justification, the expected benefit of the diagnostic procedure should exceed any expected harm. For optimization, exams should use the lowest dose possible to achieve the desired clinical outcome. Despite these principles and several campaigns that reinforce them, including the Image Wisely and Image Gently campaigns,9 there are concerns about overutilization of medical diagnostic procedures such as CT scans. Approximately 20 to 50 percent of imaging exams are thought to yield little or no clinically useful information (Cremer et al., 2014; Litkowski et al., 2016), and some studies have shown highly variable and, in some cases, higher-than-needed doses to patients (Smith-Bindman et al., 2014, 2015). Another study found that use of CT during pregnancy increased almost fourfold during the period 1996 to 2016 in the participating medical sites in the United States and Canada (Kwan et al., 2019).
As older medical equipment is replaced with more modern equipment and post-imaging software for image quality improves, the radiation dose to the patient from diagnostic procedures is expected to decrease. However, both overutilization and lack of optimization may result in unnecessary and preventable radiation risks, particularly in potentially sensitive subpopulations such as children and pregnant women and the small percentage of the population with cancer predisposition syndromes (Brodeur et al., 2017; Reid and States, 2018).
Recent studies in pediatric populations have reported cumulative absorbed organ doses of about 50 milligrays (mGy) delivered with CT were associated with triple the risk of leukemia compared to doses below 0.5 mGy. Similarly, cumulative absorbed organ doses of 60 mGy delivered by pediatric CT were associated with triple the risk of brain cancer (Abalo et al., 2021; Bernier et al., 2019; Berrington de González et al., 2021; Hauptmann et al., 2020; Pearce et al., 2012), although confounding by some other factor or reverse causation10 are arguments against concluding that this association is causal. However, systematic examination of this
___________________
9 See https://www.imagegently.org and https://www.imagewisely.org.
10 The CT scan may have taken place because of preexisting cancer and therefore not have been a cause (see Little et al., 2022a).
issue in key studies did not identify other factors (e.g., cancer predisposing conditions) that could account for the observed associations (Berrington de González et al., 2021). EPI-CT is a retrospective European multinational cohort of children and young adults subjected to CT from the 1970s to 2014. The study aims to include more than 1 million individuals, is facilitated by electronic record linkage, and uses an improved and standardized dosimetric approach; therefore, it has the potential to provide some insight on the impact of confounding by indication in medically exposed populations (Thierry-Chef et al., 2021). Results from EPI-CT are expected in the near term.
Large-scale studies of medically exposed populations facilitated by electronic record linkage have not yet been possible in the United States owing to the lack of a centralized health care system and fragmentation of patient services across multiple health care providers (NASEM, 2019c), although record linkage studies within single health care systems are common. At least three experts who briefed the committee noted that the lack of studies of medically exposed populations in the United States is a missed opportunity11 and called for a national effort to collect the data needed for such a study. This effort would require long-term buy-in and commitment from both the research and medical communities. Currently there is a general resistance among medical professional organizations in the United States to track radiation exposures from medical diagnostic procedures for the purposes of patient dose and risk assessments (AAPM, 2021). The International Atomic Energy Agency’s Safety Standard document, cosponsored by eight international organizations including the World Health Organization (IAEA, 2018), and a more recent publication (IAEA, 2021) state that tracking individual patient exposures can support the process of justification and appropriateness of a procedure and provide information on collective dose to population and trends. The dilemma today appears to be that there is enough knowledge to be concerned that medical diagnostic procedures contribute significantly to the annual average dose to the U.S. population but not enough about the actual risk to act decisively. The debate of tracking exposures from medical diagnostic procedures to inform a patient’s dose and risk assessment is likely to continue until risks at low doses are better understood.
___________________
11 Armin Ansari, Centers for Disease Control and Prevention, presentation to the committee on September 24, 2021; Rebecca Smith-Bindman, University of California, San Francisco, presentation to the committee on October 26, 2021; and Jonine Bernstein, Memorial Sloan Kettering, presentation to the committee on November 17, 2021.
2.1.3 Occupational Exposures
Workers in a variety of occupations in the United States have potential occupational exposure to low doses of radiation. These occupations include, but are not limited to, physicians and dentists, radiology and nuclear medicine technologists, workers employed in the nuclear power and nuclear fuel cycle industry, industrial radiographers, and aircrews. In addition, countries with nuclear weapons programs such as the United States, United Kingdom, and France employed hundreds of thousands of workers over the past years who were exposed to radiation due to nuclear weapons production and testing activities. In general, occupational radiation exposures have declined over the years due to several radiation protection measures adopted in medical practices and in nuclear and other industries.
Ionizing radiation has been recognized as an occupational hazard for more than a century and as an occupational carcinogen for decades. Case reports of occupational injury related to work with ionizing radiation began to appear shortly after the discovery of X-rays, and included both prompt effects of burns as well as descriptions of malignant skin tumors caused by occupational external ionizing radiation exposure. By the 1950s, there was evidence of radiation-related excess mortality among radiologists in the United Kingdom and the United States (Court-Brown and Doll, 1957; Seltser and Sartwell, 1965). Major industrial cohort studies of radiation-exposed workers began after the Manhattan Project in the United States and have permitted quantitative analysis of health effects associated with occupational external radiation exposures that had been measured by personal dosimeters worn by employees on the job. These studies have been periodically updated, expanded, and refined since the 1970s, and national cohorts have been assembled that aggregate information on nuclear industry workers as well as workers in other industries and professions that require radiation monitoring and for whom registries of radiation workers have been assembled, such as medical radiology technicians, industrial radiographers, and commercial nuclear power workers. Table 2.2 lists some of the studies on occupationally exposed cohorts and cancer risks. Outcomes other than cancer following occupational exposures are discussed in Section 2.2. A brief discussion of health effects among uranium miners can be found in Section 2.1.1.
These studies are important because the doses received have been accumulated as many low doses of radiation over protracted periods, often many years (Rühm et al., 2022), and therefore are more relevant to public exposures compared to, for example, the higher and acute doses received by the Japanese atomic bombing survivors.
Occupational exposures to radiation also occur from TENORM, which includes radioactive materials that are found naturally but have been
TABLE 2.2 Summary of Some Occupational Cohort Studies and Cancer Risks
Study | Outcome | Sample Size | Cancer Cases | Mean Dose | ERR per 100 mGy | 95% CI (90% CI) | Reference |
---|---|---|---|---|---|---|---|
Korean workers | Cancer mortality | 79,679 | 134 | 6 mSv | 0.72 | −0.5 to 2.1 | Ahn et al., 2008 |
Korean nuclear workers | Cancer incidence | 16,236 | 203 | 20 mSv | 0.21 | −0.19 to 0.9 | Jeong et al., 2010 |
Rocketdyne employees | Cancer mortality | 46,970 | 647 | 14 mSv | 0.02 | −0.18 to 0.17 | Boice et al., 2011 |
Japanese workers | Cancer mortality | 200,583 | 2,636 | 12 mSv | 0.13 | −0.03 to 0.30 | Akiba and Mizuno, 2012 |
Canadian workers | Cancer mortality | 45,316 | 437 | 22 mSv | 0.18 | −0.04 to 0.53 | Zablotska et al., 2014a |
German nuclear workers | Cancer mortality | 8,972 | 115 | 20 mSv | −0.1 | −0.4 to 0.1 | Merzenich et al., 2014 |
U.S. radiology technologists | Breast cancer | 66,915 | 1,922 | 37 mGy | 0.07 | −0.005 to 0.19 | Preston et al., 2016 |
U.S. nuclear workers | Cancer mortality | 119,195 | 10,877 | 20 mSv | 0.01 | −0.02 to 0.05 | Schubauer-Berigan et al., 2015 |
UK national registry of radiation workers | Cancer incidence | 167,003 | 13,985 | 25 mSv | 0.012 | 0.005 to 0.02 | Haylock et al., 2018 |
French nuclear workers | Cancer mortality | 59,004 | 2,536 | 26 mSv | 0.04 | −0.04 to 0.13 | Fournier et al., 2016 |
INWORKS | Cancer mortality | 308,297 | 17,957 | 21 mGy | 0.05 | 0.018 to 0.079 | Richardson et al., 2015 |
Million Person Study (analysis restricted to subpopulation of nuclear power plant workers) | Cancer mortality | 135,193 | 8,445 | 52.6 mGy | 0.01 | –0.03 to 0.05 | Boice et al., 2021 |
NOTE: CI = confidence interval; ERR = excess relative risk; INWORKS = International Nuclear Workers Study of nuclear industry workers in France, the United Kingdom, and the United States; mGy = milligray; mSv = millisievert.
SOURCE: Adapted and updated from NASEM (2019c).
concentrated or exposed to the accessible environment as a result of human activities such as manufacturing, mineral extraction, or water processing (EPA, 2008). Concentration of radioactive materials in the oil industry on the inside of pipes or in sludge has been well documented to lead to higher worker exposures and to wastes with relatively high radioactivity levels.12 Enhanced exposure to radioactivity has recently been observed in unconventional shale gas drilling workers and to occur downwind of hydraulic fracturing (Li et al., 2020). The use of the term TENORM is generally not applied to activities specifically regulated under the Atomic Energy Act of 1946 such as nuclear energy or military uses.
Exposures to aircrews differ from the occupational exposures described thus far and are primarily due to galactic cosmic radiation and solar particle events. Studies of cancer mortality among aircrew members are inconsistent and generally complicated by the effects of occupational exposures other than radiation as well as circadian rhythm disruption from crossing multiple time zones. Although increased risk of malignant melanoma, brain cancer, and leukemia has been reported in some studies (Hammer et al., 2009; Sigurdson and Ron, 2004) and female flight attendants were more likely to be diagnosed with breast cancer (Lynge, 1996; Pukkala et al., 1995), several studies have observed statistically significant lower mortality from cancer and cardiovascular diseases among aircrew members compared with the general population (Blettner et al., 2003; Yong et al., 2014; Zeeb et al., 2010, 2012). These results likely reflect the healthy worker effect of the members of this occupationally exposed group, who, due to job requirements, are also under continuing medical surveillance to maintain fitness and health qualifications to fly (Sykes et al., 2012). The literature is sparse with respect to radiation health effects on flight crew and other health outcomes. A study found that exposure during the first trimester to cosmic radiation as low as 0.36 mSv may be linked to increased risk of miscarriage (Grajewski et al., 2015).
2.1.4 Nuclear Power Operations
The nuclear energy industry produces energy by initiating and controlling a sustained nuclear chain reaction. Radioactive sources from the life cycle of nuclear power plants stem from uranium mining, milling and mill tailings, power plant operation, and nuclear waste.13 Nuclear energy facilities are subject to and must comply with multiple engineering, regulatory,
___________________
13 Other (non-nuclear) industries of electricity production are also a source of radioactive exposure. For example, waste from coal-fired power plants and from oil and gas drilling contain radioactive material. Exposures to radiation from these other industries are not generally monitored or systematically reported (UNSCEAR, 2016). See also discussion on TENORM in Section 2.1.3.
12 See https://www.epa.gov/radiation/tenorm-oil-and-gas-production-wastes.
and administrative measures to minimize unintentional releases and to ensure minimal exposures to the neighboring communities. Under normal power plant operations, only a small amount of radioactivity (a few curies to a few hundred curies) is typically released to the environment each year, contributing to a small portion of the average dose to the U.S. population. In the United States, effluent releases in airborne and liquid forms from nuclear plants must be controlled, monitored, and reported to the U.S. Nuclear Regulatory Commission (U.S. NRC) and other federal and state regulatory agencies. EPA sets the permissible annual dose equivalent to individual members of the public from a nuclear power plant at 0.25 mSv in a year but the U.S. NRC estimates that the average dose to members of the public living within a 50-mile radius of a nuclear power plant is much lower, about 0.0001 mSv.14
Populations living near nuclear facilities have expressed concerns about risks to their health from releases during routine operations of these facilities. To address these concerns, since 1985, several countries, including the United States, carried out epidemiological studies of cancer risks in populations near nuclear facilities. The majority of these studies investigated rates of cancer deaths or cancer occurrence in populations living in various-size geographic areas including counties and municipalities, zones of increasing distance, or zones based on models of dispersion of releases from the nuclear facilities (NRC, 2012). These studies have come to different conclusions, with some suggesting no associations (Heinävaara et al., 2010; Nuclear Safety Council and the Carlos III Institute of Health, 2009; White-Koning et al., 2004) and others suggesting positive associations between leukemia in children living in proximity to a nuclear facility (COMARE, 2016; Kaatsch et al., 2008; Sermage-Faure et al., 2012; Spix et al., 2008). Even studies that found positive associations have been unable to attribute them to radioactive releases from the facilities (Sermage-Faure et al., 2012).
In the United States, studies on risks to populations living near nuclear facilities are more than 30 years old. In 1991, the National Cancer Institute (NCI) compared cancer mortality rates in counties that contained nuclear facilities with rates in counties that were similar to the study counties in terms of population size, income, education, and other socioeconomic factors but did not contain nuclear facilities and found no differences in cancer rates (Jablon et al., 1991). The U.S. NRC requested that the National Academies reexamine risks around nuclear facilities and update the 1991 findings. The U.S. NRC request led to two National Academies studies that provided recommendations for the appropriate methods for assessing risks to populations around nuclear facilities (NRC, 2012) and information on
___________________
14 See https://www.nrc.gov/about-nrc/radiation/related-info/faq.html.
piloting the study to validate the recommended methods (NRC, 2014). In 2015 the U.S. NRC decided to terminate the National Academies efforts due to budgetary constraints (U.S. NRC, 2015b). In the fiscal year 2022 Appropriations Bill, Congress “encourages” the Department of Health and Human Services to contract with the National Academies to carry out the pilot study.
In addition to concerns about health effects from nuclear power plant operations that need to be addressed, uncertainties about risks from low doses such as those resulting from routine nuclear power plant operations create major challenges to the U.S. government about the acceptability of nuclear power and its future in the country’s energy mix. The risk and benefit perceptions regarding nuclear power have been dynamic, responding to changing context such as the cost and availability of clean energy and in relation to accidents that result in radiation releases (Gupta et al., 2019; see Figure 2.3). The 2011 Fukushima accident in Japan, triggered by the Great East Japan Earthquake and tsunami, revived the debate about the future of nuclear power globally and risks from unintentional releases of radiation. There were no immediate fatalities due to radiation releases from the accident and the average dose to a member of the public from releases from the accident was low (about 6 mSv to adult evacuees and 1–15 mGy to the thyroid of children; UNSCEAR, 2020b). Leukemia and thyroid cancers can manifest a few years after exposure, and other types of cancer can develop decades later. The Fukushima Prefectural Government offered thyroid ultrasound examination to children age 18 or younger. The examination revealed an unexpectedly large number of thyroid cancers among children screened that could not be explained by the relatively low doses received (NASEM, 2019b). The screening findings caused public anxiety about the health effects of radiation and raised concerns within the scientific and medical community about over-diagnosis following thyroid screening (IARC, 2018; NASEM, 2019b).
Evacuees and residents of Fukushima (Suzuki et al., 2015, 2018), similar to those from areas affected by the 1986 Chernobyl accident (Bromet, 2011, 2012, 2014; Bromet and Litcher-Kelly 2002), demonstrated high prevalence of psychological effects due to loss of life and social ties, homes, and employment; relocations; and the perceived health risk due to radiation exposure. The perceived risk and scientific uncertainty about the health effects of low-dose radiation exposures also fostered social problems. Many workers engaging in recovery operations following the Fukushima accident were likely to suffer from public discrimination resulting in general psychological distress and post-trauma. In addition, there are reports of school bullying of affected children and adolescents (Sawano et al., 2018), and many young women in Fukushima were afraid that they might be viewed negatively due to possible effects of radiation on future pregnancy
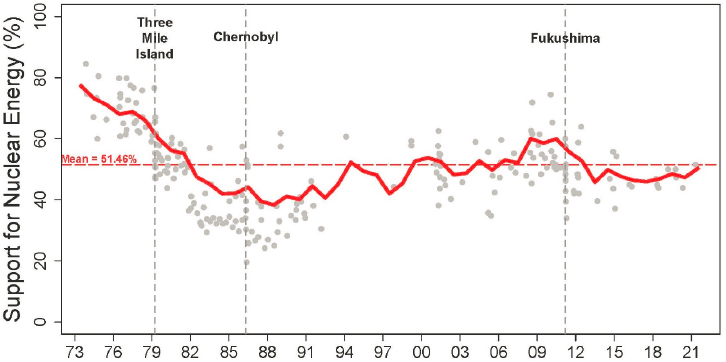
or transgenerational effects (Glionna, 2012; Save the Children, 2012). Social stigma and self-stigma are well-documented consequences of radiation exposure among survivors of the Fukushima accident as well as among the Japanese atomic bombing survivors (Amano et al., 2021) and those affected by the Chernobyl nuclear power plant accident in the former Soviet Union (Trichopoulos et al., 1987).
Scientific uncertainty about the health effects of low-dose radiation exposures also complicates government decision-making about decontamination of the plant and how to handle releases of radiation to the environment. In April 2021, the Japanese government approved the release of more than 1 million tonnes of contaminated water from the plant into the sea. According to an analysis, the resulting radioactivity in the sea will be below national regulatory standards and international guidelines (WNN, 2021). The release of the contaminated water into the sea was perceived unfavorably by environmental groups, fisheries organizations, and neighboring countries due to concerns about the possible environmental impact of the discharge (Normile, 2021).
Public attention to the Fukushima nuclear power plant accident and subsequent cleanup continues to influence the perceived balance of risk and benefit for nuclear energy (Jenkins-Smith et al., 2017). A main argument for the need for nuclear power in the world’s energy mix is its contribution toward clean energy.15 However, the operating nuclear power reactors in the United States are aging, and the number is declining. Barriers to a
___________________
15 Nuclear power results in no direct CO2 emissions; therefore, some see it as a promising energy production option for climate change mitigation. See, for example, Johnson (2019).
nuclear energy renaissance exist, among them high costs to build new reactor systems, safety concerns, and capacity for renewable energy sources to provide energy (Johnson, 2019). According to some, the general stringency in nuclear power regulation and the lack of a permanent solution for disposing of the accumulating volumes of high-level waste from more than six decades of nuclear power plant operations16 are also affecting the extent to which nuclear power can contribute in the world’s energy mix (Cohen, 1987; Cuttler and Pollycove, 2009; see also Batkins, 2016). Implementation of both clean energy and waste disposal solutions are affected by uncertainties in low-dose radiation health effects to the surrounding populations and future generations.
The Department of Energy’s (DOE’s) Office of Nuclear Energy, the office responsible for nuclear power sustainability, has announced large investments over the next few years to support the industry to further develop and deploy advanced reactors, including small modular reactors (DOE, 2020b).17 Improved knowledge of low-dose radiation health effects can impact acceptability of these new technologies, safety assessments for reactor siting, and decisions about emergency planning zones for these new technologies (Smith et al., 2021; U.S. NRC, 2020a).
2.1.5 Nuclear or Radiological Incidents
The dose to affected populations and potential health impacts of a nuclear or radiological incident depend on a number of variables including the type of incident (i.e., a nuclear power plant accident, an unintentional radiation release from a radioactive source, or attack by terrorists using a radiological dispersal device [RDD] or an improvised nuclear device [IND]); form of the radioactive material; size of the exposed population; meteorological conditions; and protective and mitigation response actions. An RDD detonation, for example, is likely to occur in highly populated areas with the intent to cause disruption and widespread panic and fear of radiation. With possible exceptions, the doses to members of the public are expected to be low (NASEM, 2019b). Similarly, doses to members of the public from a nuclear power plant accident could be reduced if appropriate protective actions such as evacuations are taken. An IND, whether it involves a surface detonation or high-altitude burst, would have severe consequences in terms of immediate casualties from physical trauma and radiation and
___________________
16 In the absence of a permanent repository for nuclear waste, federal facilities and nuclear power plants across the country store highly radioactive waste on site.
17 Developers of small modular reactors claim that they provide scalability and flexibility and can be deployed quickly and cost-effectively. This type of reactor has not yet been deployed in the United States or elsewhere.
would expose large populations to moderate to low doses of radiation from the fallout (NASEM, 2019b). Emergency workers and workers involved in long-term cleanup activities are exposed to doses higher than those of the members of the public. For example, over the first 12 months after the Fukushima accident, emergency and cleanup workers received effective doses of about 13 mSv, but a small percentage of the workforce—less than 1 percent corresponding to fewer than 200 individuals—received effective doses higher than 100 mSv with a maximum effective dose of about 680 mSv (UNSCEAR, 2020b).
In the United States, several federal agencies have responsibilities for preparedness and response to a nuclear or radiological incident, and their roles and authorities depend on the type of incident (DHS, 2016). All federal agencies involved have published plans and guidance documents to assist with a coordinated response (see CDC, 2020, for a listing). Federal plans and guidance documents for a response to a nuclear or radiological incident are based on available scientific evidence for radiation risks and are informed by recommendations from national and international bodies such as the National Council on Radiation Protection and Measurements and the International Commission on Radiological Protection (ICRP). Because many incidents are likely to involve exposures to populations within the low-dose range, knowing what the risks are from these low-dose exposures is important for responders to make the appropriate risk-benefit evaluation before they make decisions (e.g., to evacuate populations from the area). Evacuations, although they can reduce the dose of radiation to affected communities, can also cause harm, particularly when made without adequate planning, as evidenced most recently by evacuations during the Fukushima nuclear power plant accident. In the absence of direct knowledge of risks at low doses, it is not possible to optimally balance the risks and benefits of evacuations.
Vulnerable populations such as residents of nursing homes are often the most affected by evacuations, as was the case following the Fukushima accident (Nomura et al., 2013). In the Fukushima Prefecture, about 165,000 people who lived near the nuclear power plant were evacuated at the directive of the Japanese government because the annual cumulative doses would be highly likely to exceed 20 mSv after the accident, which is the standard limit for radiological protection in emergency exposure situations. Murakami et al. (2015) used the loss-of-life-expectancy measure to compare the risks of evacuation with the risks of radiation exposure caused by staying among nursing home residents and the staff members who cared for them following the Fukushima accident. They showed that the mortality risk associated with rapid evacuation without sufficient preparation was about 400 times higher than that of radiation exposure associated with staying in the nursing home for 3 months after the disaster and concluded that evacuation planning needs to be balanced with the tradeoffs against radiation-related risks (Murakami
et al., 2015). The risks of staying in the contaminated area due to damaged infrastructure, interruptions in food and medical supplies, human assistance, and other factors were not considered in this analysis.
Evacuations following the Fukushima accident also resulted in long-term displacement of large populations. Today, in Fukushima Prefecture, decontamination of prefectural land has been completed in all areas except for the zone referred to as the “difficult-to-return zone,” where annual doses are higher than 50 mSv. Still, out of the about 165,000 people who evacuated, about 35,000 remain under evacuation orders and about an equal number of evacuees has chosen not to return due to concerns about risks from radiation.18 Although the Government of Japan aims to lift the remaining evacuation restrictions by 2023 (FPG, 2021), it is likely that a considerable number of evacuees will never return because they have relocated permanently elsewhere (Budgen, 2021).
Recent radiological incidents that involved small releases of radiation and low risks to affected people, because protective actions were taken promptly, resulted in complex response to the incidents and in significant economic costs to achieve remediation at the applicable standards. One such incident is the release of a small amount of cesium, estimated to be about 1 curie (Ci) (37 gigabecquerels), during the recovery of a sealed cesium-137 source from the Harborview Research and Training Facility in Seattle, Washington, in 2019. The incident resulted in contamination (internal and external) of 13 individuals who received effective doses no greater than 0.55 mSv. Contamination of the facility forced more than 200 researchers and laboratory staff to relocate until recovery operations were complete, with direct effects on funded research programs. The projected costs for response, recovery, remediation, and reconstruction will exceed $100 million (NASEM, 2021b).
Effective communications and engagement with the impacted communities at all stages of the response to a nuclear or radiological incident are recognized by the U.S. government to be crucial as they can build credibility, inspire confidence, and ultimately contribute to an effective response. Similar to other aspects of preparing for a nuclear or radiological incident, a series of documents and tools is available.19 Although different incidents present their
___________________
18 Letter from Hisako Sakiyama, Kuniko Takagi, Yoshiyuki Segawa, Akiko Okumura, Yasuko Nagasoe, Noriko Nonaka, Tomio Negishi, and Akiko Ohashi to the committee, on February 12, 2022. According to the letter, the Government of Japan raised the public dose limit in the Fukushima Prefecture from 1 mSv per year to 20 mSv per year and stopped providing housing support to evacuees who refuse to return. The evacuees, however, especially those with small children, are concerned about risks associated with radiation exposures. The letter also states that several lawsuits have been filed by evacuees against the Japanese government claiming legitimacy of their evacuation and compensation for damages.
19 Communication resources from different federal agencies are listed on EPA’s website. See https://19january2021snapshot.epa.gov/radiation/pag-public-communication-resources_.html.
own communication challenges, questions about radiation risks (immediate and long term) from radioactive releases are expected to be central to all scenarios. Disagreement within the radiation community about health risks at low doses of radiation and the generally complicated terms and units used by experts to describe exposure may give the appearance of inconsistent public messaging and ultimately lead to loss of trust in those who deliver it (NASEM, 2019b). Communication about radiation risks with first responders, who typically only have basic training and understanding of radiation health effects, is also complicated. The Department of Homeland Security representative who briefed the committee noted that first responders are risk averse when it comes to radiation, and this aversion appears most often to derive from a lack of understanding or preconceptions about radiation effects and risks.20
2.1.6 Nuclear Weapons Program
The Manhattan Project (1942–1946) and the Cold War (1947–1991) involved activities related to nuclear weapons production and testing conducted under the auspices of the Atomic Energy Commission (AEC) and later DOE. Nuclear weapon production activities were carried out with a sense of urgency and under policies that demanded immense secrecy. At Hanford Nuclear Site, a site built in the 1940s to produce plutonium for nuclear weapons, volatile gases from fuel processing were vented directly into the atmosphere, and reactor cooling water contaminated with chemicals, metals, and radioactive materials was discharged into subsurface soil, groundwater, and the Columbia River, exposing populations in the surrounding areas to radiation. It was not until later that DOE released previously unavailable or classified documents about past operations. For example, in the mid-1980s, as a result of repeated Freedom of Information Act public requests and media stories, DOE released information on past operations at the Hanford Site. The released information showed that large amounts of iodine-131 (about 740,000 Ci or 2.73 × 1016 Bq; see Heeb et al., 1996; Napier, 2002) and other radioactive materials were released into the air from Hanford from 1944 through 1957.
In July 1945, the United States conducted the world’s first atomic bomb test at the Trinity site south of Los Alamos, New Mexico. Soon after that, U.S. bombers dropped atomic bombs on Hiroshima and Nagasaki as part of a strategy to end World War II. After the war’s end, the Cold War led to new national defense priorities and prompted the government to initiate a program of nuclear weapons testing. More than 1,000 atomic weapons tests took place between 1945 and 1992, the majority of which were conducted at the Nevada Test Site. Tests were also conducted in other places
___________________
20 Jonathan Gill, Department of Homeland Security, presentation to the committee on October 27, 2021.
within the United States and the Marshall Islands, primarily on Bikini and Enewetak atolls, which were then designated as United Nations Trust Territory governed by the United States. Although most tests were underground tests, some were atmospheric tests in which the atomic weapons exploded at or above ground level, resulting in radioactive material being released into the atmosphere, some of which reached ground as nuclear fallout. In contrast to nuclear weapons production operations, nuclear tests were generally not a secret. The tests were considered events or spectacles announced in advance, and the public and test-site workers observed the events often with encouragement to do so at the expense of personal safety or protection (NRC, 2005). In 1955, the AEC distributed a booklet outlining the testing procedures (AEC, 1955) and generally underplayed the risks to the exposed populations. All areas of the United States received fallout from at least one nuclear weapons test, but larger amounts of I-131 from the Nevada Test Site fell over some parts of Utah, Colorado, Idaho, Nevada, and Montana.21 The radioactive fallout from those tests represents a source of continuing exposure even today, albeit at very low levels (UNSCEAR, 2008).
The nuclear weapons program also resulted in radiation exposures to people employed in uranium mining and milling enterprises and their families from inhaled radon progeny and other hazards in the mines. People of the Navajo Nation worked the mines, often living and raising families in close proximity to the mines and mills. Navajo miners have reported that they were not educated about the hazards of uranium mining and were not provided with protective equipment or ventilation (Dawson, 1992). Today the mines are closed, but a legacy of uranium contamination remains, including more than 500 abandoned uranium mines as well as homes and water sources with elevated levels of radiation.22 Disparities in tribal infrastructure, social and behavioral risk factors for disease, and complex environmental stressors are known to exacerbate risks for disease to Indigenous people (Lewis et al., 2017). These disparities also raise important health questions, for example, whether these communities are at higher risk of developing disease from low-dose radiation exposures and social questions regarding environmental injustice.
A variety of legal, political, and social actions taken by groups representing downwinders, test-site workers, veterans, and uranium workers pressured the government to accept liability for its actions and offer compensation for the damage. As a result, several compensation programs emerged, which are described in congressional testimony (U.S. Congress, House, 2021) and briefly in Box 2.1 in order of enactment.
___________________
21 See https://www.cancer.gov/about-cancer/causes-prevention/risk/radiation/stateandcountyexposure.
22 See https://www.epa.gov/navajo-nation-uranium-cleanup/abandoned-mines-cleanup#
Based on the input from community representatives to the committee, it is apparent that there is continuing distrust toward the U.S. government, and there is a belief that the U.S. government has failed to accept responsibility for past radiation exposures and has failed to develop programs that adequately compensate all impacted groups.23 These groups include downwinders who resided in geographic areas outside those designated by the Radiation Exposure Compensation Act (RECA), uranium workers who worked in mines after 1971, and servicemen who were involved in the cleanup of Enewetak Atoll. The Radiation Exposure Compensation Act Amendments of 2021 (H.R. 5338) aim to extend and expand RECA to include several of these groups, but without further congressional action, RECA will expire in July 2022.
Distrust toward the U.S. government also continues within the Marshallese government and impacted atoll communities. The U.S. government officially returned the atolls to the Republic of the Marshall Islands in 1986. Today, all the atoll islands and the lagoon are accessible except for Runit Island, which contains a structure with radioactively contaminated soil and debris and therefore remains quarantined. Recently, concerns about the leaking structure and the rising sea level around the Marshall Islands led Congress to request that DOE provide a plan to repair the structure, evaluate the environmental effects on the lagoon over the next 20 years, and assess the potential risk to the people who live near it. DOE (2020a) found “no evidence to suggest that the containment structure represents a significant source of radiation exposure relative to other sources of residual radioactive fallout contamination on the atoll.” The Marshallese government rebutted DOE’s finding and noted that the agency is “downplaying” the risks and is declining to take responsibility for Runit Dome and its leaking contents.24
Human health studies on the impacts of nuclear weapons program activities are generally few and have inherent uncertainties, having been conducted decades after the initial exposures occurred when critical data on releases and exposures were often missing or were unreliable because of
___________________
23 Stakeholder engagement panel discussion with President Jonathan Nez, Navajo Nation; Jill Jim, Navajo Nation Department of Health; Mary Dickson, representative of downwinders of U.S. nuclear tests; Keith Kiefer, National Commander of the National Association of Atomic Veterans; Benetick Maddison, Marshallese Educational Initiative (pre-recorded presentation); Arjun Makhijani, Institute for Energy and Environmental Research; Trisha Pritikin, Hanford Downwinder, author of The Hanford Plaintiffs: Voices from the Fight for Atomic Justice; and Beata Tsosie-Peña, Environmental Health and Justice Program at Tewa Women United in New Mexico (pre-recorded presentation) on October 28, 2021.
24 Benetick Maddison, Marshallese Educational Initiative, pre-recorded presentation to the committee on October 28, 2021.
inadequate recordkeeping and monitoring. A sample of these health studies and their conclusions are listed in Box 2.2. Many of these studies recognize the great uncertainty in the estimates of radiation doses and caution that the number of cancer cases possibly attributed to the exposures are also uncertain. Radiation from fallout from atmospheric weapons tests has been dramatically reduced by radioactive decay and weathering (NCRP, 2009a).
2.1.7 Nuclear Waste Management
As the Cold War came to a close in 1991, the United States shifted its focus from nuclear weapons production to remediation of nuclear weapons production facilities. Management of nuclear weapons legacy waste is often used as a demonstration that the federal government is making large expenditures of taxpayer funds to address uncertain and in some cases potentially low or non-existent risks to current and future generations due to exposures to low doses of radiation (Blush and Heitman, 1995). Others have stated that the assumption that even low doses of radiation carry risks make radiation contamination cleanup goals stricter, resulting in extraordinary cleanup costs (Cardarelli and Ulsh, 2018) and misallocation of scarce national resources. Implementation of less stringent regulatory measures and cleanup criteria could accelerate cleanup and result in billions of dollars in savings.
Cleanup of nuclear weapons legacy waste is primarily the responsibility of the DOE-Environmental Management (EM) cleanup program. The program was established in 1989 and has cleaned up 91 sites at a cost of about $170 billion (GAO, 2019). However, the largest and most complex sites—the Hanford Site in Washington, the Savannah River Site in South Carolina, the Oak Ridge Reservation in Tennessee, the Idaho Site, and the Portsmouth Site in Ohio—still require remediation. At all these sites, radioactive and other hazardous contaminants are present in soil, waste burial grounds, groundwater, or surface water. DOE-EM projects related to cleanup of the remaining 16 sites will continue for at least another 50 years (until 2070 or beyond) at an estimated cost of $406 billion (GAO, 2021). DOE’s cleanup responsibilities are almost certainly underestimated because many of the cleanup technical challenges have not yet been sufficiently characterized and costs have not been estimated (NASEM, 2019a).
Doses to the populations living near nuclear facilities that currently hold legacy waste are considered to be very low. According to the National Council on Radiation Protection and Measurements (NCRP, 2009a), the annual exposure to a maximally exposed individual (MEI)25 ranges from a few microsieverts (µSv) to a fraction of a µSv. For example, the annual exposure to the MEI is estimated to be 8.5 µSv for the Portsmouth Gaseous Diffusion Plant and 0.1–0.6 µSv for the Hanford Site. Still, the lives of communities living near the nuclear waste sites are disrupted by cleanup activities and decisions about these activities, with Indigenous communities being disproportionally impacted. At Hanford, environmental contamination of the land and Columbia River (see Figure 2.4) has presented a health risk to
___________________
25 DOE defines an MEI as a hypothetical individual who because of proximity, activities, and living habits would receive the highest radiation dose, taking into account all pathways, from a given event, process, or facility. See https://www.directives.doe.gov/terms_definitions/maximally-exposed-individual.
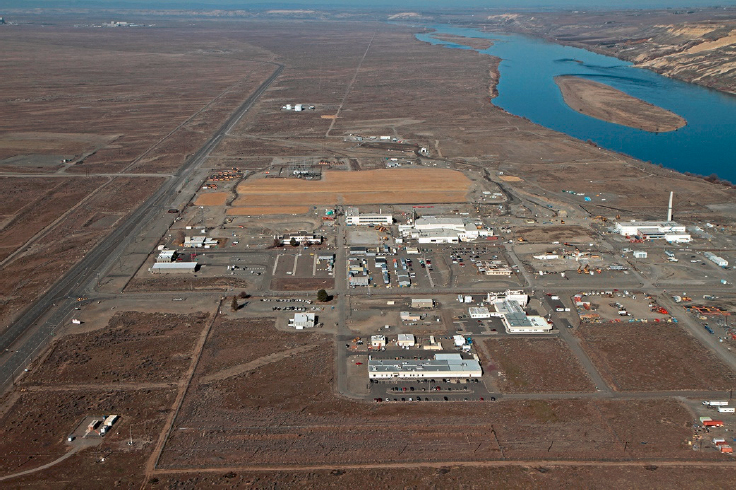
members of the Yakama Indian nation, the Umatilla Tribe, the Wanapum, and the Nez Perce Tribe, and has impacted their cultures as they can no longer use the land for farming, hunting, fishing, or ceremonial purposes.26 Many of the impacted tribes are engaged with the federal government to develop strategies to restore the habitat of the Columbia basin, but unmet milestones and violated agreements27 create issues of distrust.
Concerns about health effects from legacy waste continue to be raised by other communities near nuclear facilities that hold legacy waste and are still in the process of remediation. For example, the 2019 discovery of trace amounts of neptunium-237 near the Zahn’s Corner Middle School in proximity to the Portsmouth Gaseous Diffusion Plant prompted the closing of the school and attracted national media attention. The news stories highlighted the local community’s distrust of DOE, which was exacerbated
___________________
26 See https://www.atomicheritage.org/history/native-americans-and-manhattan-project.
27 Cleanup schedule milestones and endpoints are described in legally enforceable agreements with states and regulators that govern the work to be done. Most of DOE’s major sites have more than one agreement in place. For example, DOE, EPA, and the State of Washington Department of Ecology signed a cleanup and compliance agreement (known as the Tri-Party Agreement) in 1989 for cleanup work to be carried out at the Hanford Site. See https://www.atg.wa.gov/hanford for legal actions related to unmet milestones of the Tri-Party Agreement.
by the delayed release of the 2017 environmental report that first detected the contamination (Ali, 2019). There has been an ongoing discussion about independent examination of the contamination and risks. Concerns about health effects have also been raised by communities near nuclear facilities where cleanup activities have been completed, such as Rocky Flats in Colorado (Tabachnik, 2019).
The lingering mistrust of DOE’s nuclear waste cleanup program is at least partly due to the agency being relatively slow in stakeholder involvement and risk communication for its cleanup program. In 1978, DOE established the New Mexico Environmental Evaluation Group (EEG) to conduct an independent technical evaluation of the potential for radiation exposure to people near the Waste Isolation Pilot Plant (WIPP) Project which became operational in 1999 for the disposal of transuranic radioactive wastes generated by the national defense programs (Neill et al., 1996). EEG was defunded in 2004. Among the last activities conducted by EEG was a workshop on technical issues regarding some of the high-level waste tanks at the Hanford Site and whether some of the wastes could be disposed of at WIPP.28
Based on the recommendation of the National Research Council (NRC, 1994) to involve academia to provide more trust in cleanup activities, DOE began to fund university-based programs. The Consortium for Risk Evaluation with Stakeholder Participation (CRESP), a partnership among university researchers, has been a major recipient of the funding and has been working with DOE through cooperative agreements since 1995. Research goals of these agreements have been largely based on eliciting community concerns and on helping DOE-EM make cleanup decisions by reducing significant uncertainty about risks at the nuclear waste sites that interferes with or delays implementation of cleanup.
CRESP aims to better address citizens’ concerns and needs by engaging with stakeholders and integrating their input into the research process from problem formulation, to analysis of data, to dissemination of research findings (Goldstein et al., 1999). An example of CRESP’s approach is the involvement of the Aleut people in testing radionuclide levels in biota, subsistence foods, and commercial fish on and around Amchitka Island in southwest Alaska, a site of three underground nuclear tests from 1965 to 1971. CRESP’s participatory approach resulted in acceptance of the testing results by the impacted communities and termination of DOE’s managerial responsibility for the site (Burger et al., 2009). Despite this and other examples that demonstrate the effectiveness of CRESP in engaging with
___________________
communities around nuclear sites, some have expressed concerns about CRESP’s independence from DOE.29
2.2 CURRENT EPIDEMIOLOGICAL EVIDENCE ON LOW-DOSE RADIATION HEALTH EFFECTS
This section summarizes some epidemiological evidence of low-dose radiation health effects. Similar to Section 2.1, the committee does not make an attempt to provide a complete review of the existing literature. The primary focus of the section is on most recent studies on the adverse health effect discussed to indicate the current state of knowledge.
Cancer is the most well-established and most studied adverse health effect following exposure to radiation (Section 2.2.1). Recently, associations of other adverse health outcomes including cardiovascular disease (Section 2.2.2), neurological disorders (Section 2.2.3), immune dysfunction (Section 2.2.4), and cataracts and other lens opacities (Section 2.2.5) have been observed at doses lower than previously considered important for these effects. Heritable genetic effects (Section 2.2.6) are also a concern following low-dose radiation exposures. As a result, there is increasing interest in the radiation research and radiation protection communities to better understand the risks and mechanisms of radiation effects at low doses and low dose rates. Research into the mechanisms of low-dose radiation effects has the potential to contribute to improved understanding of risks of adverse health outcomes in humans and into the factors that modulate these risks. Some proposed mechanisms for the radiation-linked health effects are also discussed in this section.
2.2.1 Cancer
Cancer is the most well-established adverse health effect following exposure to radiation, and, accordingly, the extensive literature on this topic is reviewed in a number of publications (Berrington de González et al., 2017; Hauptmann et al., 2020; Kitahara et al., 2015; Little et al., 2022b; Rühm et al., 2022; UNSCEAR, 2006a). The following subsections briefly describe the state of knowledge regarding radiation-related cancer risks following postnatal exposure and exposure in utero, focusing on low-dose exposures.
Postnatal Exposures
Radiation-related cancer risks differ by organ or tissue. The most radiosensitive tissues are the bone marrow, brain, thyroid, skin, and breast,
___________________
29 Letters to the committee from impacted community members and members of advocacy groups on January 6, 2022, and February 1, 2022.
although cancer risks have been demonstrated following radiation exposure for nearly every tissue and organ in the body. Cancers that have not been convincingly or consistently linked to low-dose radiation include chronic lymphocytic leukemia, Hodgkin’s and non-Hodgkin’s lymphoma, and melanoma (Boice et al., 2022b). Compelling evidence based on results from various exposure scenarios supports that, for a given dose, cancer risks are higher among individuals exposed at younger ages. Sex has also been shown to modify the radiation dose-response relationship, and in some cases higher risks are reported for women compared to men (ICRP, 2007; see additional discussion in Chapter 5).
The key data on these observations derive from the Japanese atomic bombing survivor cohorts for individuals of all ages (see Section 4.5.6) and from medical exposures (particularly CT scans, as reviewed in Section 2.1.2), natural background radiation (Section 2.1.1) in childhood, and occupational exposures (Section 2.1.3) in adulthood. The evidence from these studies is also generally consistent with a linear relationship between radiation dose and cancer risk across the dose ranges investigated, even <100 mGy, albeit with different magnitudes of risk for different sexes and tissues. However, a nonlinear relationship at low doses cannot be ruled out. Although substantial data have been presented on the modification of radiation-related risks by age at exposure, data on other potential modifiers of risk are sparse and generally inconsistent.
In Utero Exposures
Overall, in utero exposure studies have revealed that the risk of developing cancer following radiation exposure is no greater than that following exposure during early childhood. The association between low doses of ionizing radiation received by the fetus in utero from diagnostic radiography and the subsequent risk of cancer in childhood was initially reported in the 1950s when the frequent use of abdominal X-ray for pregnant women in the United Kingdom was common. At the time and until about 1975, the frequency of abdominal X-raying of pregnant women accounted for 10–15 percent of all pregnancies and >90 percent during the third trimester. The Oxford Survey of Childhood Cancers (OSCC) was the first and largest in utero exposure study to provide risk estimates with reasonable precision. The fetal dose received was around 10 mGy of X-rays, and the excess absolute risk coefficient at this level of exposure is approximately 6 percent per gray (Doll and Wakeford, 1997; Wakeford and Little, 2003). OSCC found an almost uniformly raised relative risk for nearly all types of cancer that are most frequent in children. This uniformity in cancer risk is the most notable argument against the observed associations being causal. A recent study compared the results found in OSCC with those of all other
case-control and case-cohort studies (Wakeford and Bithell, 2021). The study found that the meta-analysis of the case-control and case-cohort studies also showed consistent and clear elevations of risk for all types of childhood cancer combined, all leukemia, and all cancers except leukemia combined. The expert who briefed the committee, who was also the author of the study, noted that on the balance of the evidence, it seems likely that this association has a cause-and-effect interpretation, but greater understanding of biological mechanisms is required for definitive conclusions to be drawn for specific types of cancer.30
In the Japanese atomic bombing survivor cohorts, individuals exposed in utero received a range of doses with an estimated mean dose of 122.9 mGy, but about half received less than 5 mGy (using maternal uterine dose as a proxy; Sugiyama et al., 2021). Early studies of the atomic bombing survivors showed impaired physical and mental development among children exposed in utero. Associations between in utero exposure and excess cancer mortality or incidence were not observed until later when there was sufficient accumulation of data that accompanied the aging of the cohort (Ozasa et al., 2018). The magnitude of the risks was comparable to those observed in survivors who were exposed at young age (Preston et al., 2008). The most recent update found that atomic bomb radiation was associated with mortality from solid cancer for females but not males (Sugiyama et al., 2021). Only a small portion (14 percent) of the survivors exposed in utero had died at the end of follow-up, so this cohort is expected to continue to provide valuable information on the effects of in utero exposure and cancer mortality later in life. Long-term excess occurrences of leukemia following in utero exposure in the atomic bombing survivor cohorts have not been observed, but these results need to be interpreted cautiously due to the shorter latency period between radiation exposure and leukemia compared with solid tumors.
2.2.2 Cardiovascular Disease
The heart and vasculature have long been recognized as potential targets for the adverse effects of radiation exposure at high doses (see, e.g., HPA, 2010; ICRP, 2012). A substantial body of evidence relating to the effects of radiation on the heart comes from studies following breast cancer patients after radiotherapy, where doses to the heart are on the order of 1–2 Gy and disease presents 10–20 years after exposure (ICRP, 2012). Risk from lower and more protracted exposures has also been observed, for example, in studies of the Japanese atomic bombing survivors
___________________
30 Richard Wakeford, University of Manchester, United Kingdom, presentation to the committee on November 16, 2021.
(Ozasa et al., 2012) and in occupational exposure studies (Azizova et al., 2018; Gillies et al., 2017). In 2012, ICRP issued a statement that “medical practitioners should be made aware that the absorbed dose threshold for circulatory disease may be as low as 0.5 Gy to the heart or brain” (ICRP, 2012). Since the ICRP statement was issued, several analyses and meta-analyses of low-dose radiation epidemiological studies have been published (e.g., Azizova et al., 2018; Gillies et al., 2017; Little et al., 2012, 2021a; Ozasa et al., 2012; Rajaraman et al., 2016; Tran et al., 2017; Zhang et al., 2019). Some of the analyses and meta-analyses indicate an elevation of risk at doses less than 500 mGy (e.g., Little et al., 2012; Tran et al., 2017) and that risk of circulatory disease may follow a linear dose-risk relationship at doses below 500 mGy (Little et al., 2012). Other findings include a reduction in risk with increasing time since exposure (Tran et al., 2017) and an inverse dose-fractionation association in ischemic heart disease mortality in the Canadian Fluoroscopy Cohort Study (Zablotska et al., 2014b). Given the high prevalence of cardiovascular disease, if these associations are confirmed, the estimates of overall radiation-related mortality would approximately double compared with the current estimates based on cancer alone (Little et al., 2012). Many of the epidemiological studies have not fully controlled for potential confounding exposures, notably tobacco smoking and alcohol consumption, and therefore explanations for the observed increased risk in cardiovascular disease other than radiation are plausible (Wakeford, 2019).
2.2.3 Neurological Disorders
The adverse effects of high-dose radiation (Armstrong et al., 2010; Chen et al., 2015) and of radiation exposure prenatally (Black et al., 2019; NCRP, 2013; Otake and Schull, 1998) on neurological disorders are well established. However, the effects of postnatal radiation exposure on neurological disorders including cognitive impairment at low doses and low dose rates are poorly characterized in part because of the difficulty in exploring cognitive deficits in experimental animals and because of the difficulty in discerning the subtle changes that need to be measured at low doses.
Studies funded by the National Aeronautics and Space Administration documented some evidence of cognitive dysfunction in rodent systems, predominantly following high linear energy transfer (LET) GCR exposures to brain tissue (Jones et al., 2020; Perez et al., 2020), including possible inflammatory responses in the central nervous system (Rola et al., 2005). Cognitive decline is likely the result of damage in multiple neural cell types due to vascular changes, glial and neuronal cell function, decreased neurogenesis, and an enhanced inflammation in the central nervous system (Hladik and Tapio, 2016).
In population studies that involve low-LET radiation, Chernobyl cleanup workers were reported to have some cognitive impairment related to effects on the hippocampus, and the effects were evident among workers with doses above 100 mSv, particularly among those with doses above 500 mSv (Bazyka et al., 2015). Blomstrand et al. (2014) noted few cognitive effects following low-dose irradiation of the infant brain in a population-based cohort study in Sweden. More recently, Azizova et al. (2020) demonstrated a linear association of Parkinson’s disease incidence within the Mayak Worker study31 with cumulative dose from external radiation exposure to the brain above 100 mGy, and the risk estimate increased with increasing dose. The Million Person Study is also addressing the issue of low-dose and low-dose-rate exposures and the impact on dementia, Alzheimer’s disease, Parkinson’s disease, motor neuron disease, and cognitive impairment, with some unpublished data suggesting the presence of an effect for some of these adverse health outcomes (Boice et al., 2021).
A recent review of the literature on possible cognitive impairment at low doses of radiation concluded that both biological and epidemiological research provide evidence for an effect, but a better characterization of the effects and improved understanding of the mechanisms are needed (Pasqual et al., 2021).
2.2.4 Immune Dysfunction
A comprehensive review of available data on radiation effects on the immune system was published by the United Nations Scientific Committee on the Effects of Atomic Radiation (UNSCEAR, 2006b) and recently updated with a focus on low-dose radiation effects (UNSCEAR, 2021). This and subsequent evidence indicate that radiation at low doses can significantly alter immune homeostasis by changing the balance within the immune system, its functional integrity, and overall resilience (Lumniczky et al., 2021). Epidemiological, clinical, and experimental studies at low doses in this field tend to report rather subtle changes compared with the more dramatic effects of high-dose exposures, where cytotoxicity plays a major role in immune response, and the clinical effects of this immune dysfunction remain poorly understood.
There is some clinical and experimental evidence that low-dose radiation can drive the functional profile of CD4+ T cells toward a Th2
___________________
31 The study follows workers, both men and women, who during the period 1948–1982 were employed at the Mayak Production Association, the first nuclear complex in the former Soviet Union. Workers were exposed to external gamma rays and to internal exposures from plutonium inhalation which were relatively high during the early years of operations of the nuclear complex.
phenotype.32 CD8+ T-cell damage tends to be less consistent, perhaps because of variation in radioresistance, which may be associated with activation status. A rise in rare double negative CD4−CD8−, alpha/beta T cells has also been reported (Kusunoki et al., 2003; Kyoizumi et al., 2010), which in a non-radiation setting has been associated with lymphadenopathy and splenomegaly, autoimmunity, and autoimmune lymphoproliferative syndrome (Bleesing et al., 2002). Dose-dependent increases in T-cell receptor (TCR) mutant frequency were noted in Chernobyl cleanup workers (Saenko et al., 2011) while the TCR repertoire seems more limited, at least in atomic bombing survivors, which may be associated with low-grade inflammation later in life that involves myeloid cells, known as inflammaging, or defective thymic output (Denkinger et al., 2015; Franceschi et al., 2019; Fulop et al., 2018; Kusunoki and Hayashi, 2008; Kyoizumi et al., 2010).
Epidemiological evidence generally points to the peripheral lymphocyte balance shifting in favor of B cells with increased serum immunoglobulin (Ig) levels and some degree of humoral immune enhancement, even when some individuals seem more prone to certain bacterial infections. However, a dose-dependent decrease in B cells, activation of T cells, and increase in cytokine interleukin (IL)-1 levels can also be seen in individuals externally exposed to whole-body doses below 100 mSv in Chernobyl studies (Ilienko et al., 2018).
Experimental studies of the functional effects of low-dose radiation on humoral immunity are few but stimulatory effects are also generally seen on natural killer (NK) cells. Although these studies are often short term and performed in vitro, Oradovskaia et al. (2011) studied a cohort of cleanup workers who developed malignant disease, and they identified reduced CD3+CD4+ T-cell levels, increased CD8+ T-cell levels, a reduced CD4/CD8 ratio, and a prevalence of NK T cells over conventional NK cells 1–3 years before the manifestation of cancer. Radiation exposure increased the frequency and counts of monocytes in a dose-dependent fashion, and this increase was more evident after 60 years, showing a possible acceleration of age-dependent clonal hematopoiesis (Yoshida et al., 2019). However, among the elderly atomic bombing survivors, response to vaccination did not appear to be impaired by radiation exposure early in life (Hayashi et al., 2018). The perturbed lymphocyte homeostasis, especially at the level of the TCR and the skewing toward memory over naïve subsets, combined with the myeloid shifts that can alter the antigenic epitopes that are presented,
___________________
32 A Th2 profile is indicative of T helper cell differentiation toward production of interleukin (IL)-4, IL-5, IL-9, or IL-13 and has been historically seen as a sign of an immune system skewed toward B-cell activation and antibody production. The Th2 profile is often seen in diametrical opposition to the TNF-alpha, IFN-gamma, and GM-CSF pro-inflammatory Th1 profile needed for macrophage and cytotoxic T-cell activation during cell-mediated immunity and delayed-type hypersensitivity.
provide the framework for induction of symptomatic immune dysfunctions that are clinically relevant such as infections, allergies, autoimmunity, and immunoproliferative diseases. Although it is tempting to relate some of these observations to the anti-inflammatory effects of low-to-moderate doses of low- and high-LET radiation that have frequently been reported to improve the clinical symptoms in individuals with local inflammatory conditions (Donaubauer et al., 2021; Micke et al., 2017; Trott and Kamprad, 1999), long-term observations of cohorts exposed to acute or chronic low doses of radiation indicate a pro-inflammatory immune profile, which might contribute to chronic immune dysfunction and disease.
2.2.5 Cataracts
Cataracts are defined as the opacity or clouding of the lens of the eye, a process that is typically associated with aging (Hamada et al., 2020). It is the leading cause of visual impairment in the world and can be repaired through surgery and replacement with an artificial lens. Classification of cataracts falls into three major categories: cortical, nuclear, and posterior subcapsular (PSC), with PSCs and cortical associated directly with radiation exposure (Hamada et al., 2020). The eye lens is considered one of the most radiosensitive tissues; biological and mechanistic studies in in vitro and in vivo studies have determined that DNA damage and repair mechanisms, extracellular matrix effects, membrane damage, dysregulation in protein and gene expression, and oxidative damage and intercellular communication are some of the processes contributing to lens opacity and cataract development (Ainsbury et al., 2021, 2022; Hamada et al., 2020). Morbidities and environmental exposures other than radiation can also contribute to cataract development.
For more than 50 years, cataracts were considered a tissue reaction with a threshold of absorbed dose to the eye of 2 Gy and annual dose limits to the lens were set at 150 mSv. As more sensitive detection methods for cataracts emerged, the threshold was reduced to 500 mGy (Neriishi et al., 2007), resulting in further reduction of the equivalent dose limits to the lens of the eye for occupational exposures to 20 mSv averaged over 5 years, not to exceed 50 mSv in 1 year (ICRP, 2012; see also discussion in Section 3.3 for NCRP, 2016 recommendation). Shore (2016) reviewed epidemiological data reported since the 2012 ICRP publication and suggested that there could be a dose threshold somewhere between a few hundred mGy and 1 Gy, with little or no excess risk below 100 mGy. Another review by Thome et al. (2018) that included data from the Japanese atomic bombing survivors, Chernobyl cleanup workers, medical workers, and radiotherapy patients suggested that the evidence of an increase in cataract formation at doses below 500 mGy was inconclusive.
New studies continue to provide evidence of radiation-induced cataract at lower doses. The Mayak worker study has shown that the risk for cataracts increased linearly with chronic cumulative effective dose from external gamma rays at ≥250 mSv (Ainsbury et al., 2021). The U.S. Radiologic Technologists cohort study, on the other hand, suggested that radiation exposure at lower doses (<100 mGy) and low dose rates (<0.0005 Gy/h) can indeed lead to cataract development (Ainsbury et al., 2021; Little et al., 2020a,b, 2021a). In general, epidemiological evidence supports a lack of a clear dose-rate effect, with more data needed for a decision on dose-rate dependence (Ainsbury et al., 2021). Regarding high-LET radiation, there is clear evidence of cataract development in astronauts, although collection of epidemiological data was discontinued in 2012 (Hamada et al., 2020). Interventional cardiologists also show an increased risk for PSC opacities (Elmaraezy et al., 2017), seen also in populations living in high background areas, such as in the area of Yangjiang, Guangdong, China (Ainsbury et al., 2021).
2.2.6 Heritable Genetic Effects
A main concern of populations exposed to radiation, including the Japanese atomic bombing survivors, those exposed to radiation from the Chernobyl and Fukushima nuclear power plant accidents (Suzuki et al., 2018), and cancer survivors who undergo radiation therapy, has been the induction of heritable genetic effects due to damage to the DNA of germ cells in the gonads that can cause gene mutations. These mutations, in contrast to mutations in somatic cells, can be transmitted from generation to generation. Heritable genetic effects can include, but are not limited to, malformations, metabolic disorders, chromosome aberrations, and other effects. Although early experimental findings in fruit flies (Muller, 1927) and mice (Russell, 1951) have indicated that ionizing radiation causes heritable genetic effects, human data have not replicated the early experimental findings and often have had limited statistical power owing to small sample size, low gonadal dose, lack of dosimetry information, or inadequate comparison groups (Boice and Miller, 1999; Lim et al., 2015; Signorello et al., 2010).
Among the first studies of the atomic bombing survivors to explore possible heritable genetic effects were studies of untoward pregnancy outcomes such as major congenital malformations, stillbirths, or neonatal deaths, among births to both maternal and paternal survivors. Past analyses of untoward pregnancy outcomes among the survivors (Neel and Schull, 1956; Otake et al., 1990; Schull et al., 1981) generally showed a positive association between total parental dose and the frequency of untoward pregnancy outcomes, but they did not identify significant associations. Researchers updated the latest analysis to account for malformations that
were not included in the 1990 analysis due to different inclusion/exclusion criteria and for updated estimates of gonadal dose (Yamada et al., 2021). The updated analysis showed that parental exposure to radiation was associated with increased risk of major congenital malformations and perinatal death, but the estimates were imprecise for direct radiation effects, and most were not statistically significant. The mean maternal, paternal, and conjoint doses were 30 mGy, 20 mGy, and 50 mGy, respectively (Yamada et al., 2021). The investigators discuss that harsh living conditions and the limited socioeconomic resources available to heavily exposed atomic bombing survivors after the war might have led to an overestimate of genetic effects of radiation and suggest that additional insights on radiation and reproduction might be gained by using contemporary genomic methods to compare the DNA of parents irradiated by the bombings with that of their children (Yamada et al., 2021).
Studies of the offspring of the atomic bombing survivors (F1) also aim to understand the potential heritable genetic effects of radiation by addressing whether radiation-induced mutations that might have been passed to the children of the survivors alter cancer and non-cancer incidence and mortality rates. These studies showed no increase in the children’s mortality rates in relation to parental gonadal exposure (Grant et al., 2015). In addition, earlier molecular analyses of the F1 cohort for possible heritable genetic effects of radiation showed no changes in the sex ratio of births, no increases of chromosomal aberrations among children of exposed parents (Neel, 1998), and no changes in mutation rates at microsatellite loci (Kodaira et al., 2010). Planned genetic analysis of 1,000 parent–child trios, in which parents received varied levels of radiation exposure due to the bombings and children were conceived after the bombings, could help better understand the overall effects of preconception exposure to radiation on this population and on humans in general.33
Trio studies have been conducted using samples from individuals exposed to radiation from the Chernobyl accident. In 2021, NCI and collaborators published the findings of a study that highlighted how advances in DNA sequencing technology and comprehensive genomic analysis in well-designed epidemiological studies can enable addressing questions related to hereditary effects of radiation (Yeager et al., 2021). Investigators analyzed the complete genomes of 130 individuals born between 1987 and 2002 (i.e., between 46 weeks and 15 years after the accident) to parents exposed to radiation from the 1986 Chernobyl accident and their 105 mother–father pairs. The parents were exposed because they were either cleanup workers or residents who had been evacuated because they lived in close proximity
___________________
33 Robert Ullrich, Radiation Effects Research Foundation, presentation to the committee on January 24, 2022.
to the accident site. The study found no evidence that radiation exposure to parents resulted in new genetic changes (de novo mutations) being passed from parent to child regardless of cumulative preconception gonadal paternal (mean dose 365 mGy) or maternal (mean dose 19 mGy) exposure to radiation.
Survivors of childhood cancers and their children are the largest group of people exposed to high doses of radiation for therapeutic purposes before reproduction and can provide insights on the potential heritable genetic effects of radiation. The Childhood Cancer Survivor Study provided evidence that the children of cancer survivors are not at significantly increased risk for congenital anomalies due to their parent’s exposure to radiation or other mutagenic cancer treatments. The mean ovarian radiation dose in this study was 1.19 Gy and the testicular radiation dose was 0.48 Gy (Signorello et al., 2012). Tawn et al. (2011) also reported no increases in mutation rates among children born to survivors of childhood cancer who had received radiotherapy.
2.3 CHAPTER SUMMARY AND FINDING
The U.S. population is exposed or could potentially be exposed to low-dose and low-dose-rate radiation from a number of different sources including natural radiation sources, medical applications, occupational exposures, nuclear power routine operations and accidental releases, nuclear or radiological incidents, the nuclear weapons program, and nuclear waste management. These sources are important in terms of low-dose and low-dose-rate exposures either because they contribute a large portion to the average annual dose to the U.S. general population or to specific populations (e.g., medical applications), or they are of concern to members of the public and impacted communities (e.g., nuclear waste management) or to current and future U.S. policies and plans (e.g., nuclear power operations).
These low-dose radiation sources involve different types of radiation (e.g., alpha, beta, or gamma), routes of exposure (internal or external), and duration of exposure (acute or protracted). Today, in the absence of a mechanistic understanding of different low-dose and dose-rate radiation sources and their direct health effects, estimation of risks and decisions related to exposures from substantially different sources are often made using the same generic approach—by relying on risk estimates derived from higher, acute, external exposures to radiation, largely of the Japanese atomic bombing survivors, and applying appropriate correction factors.
Finding 1: A coordinated multidisciplinary low-dose radiation research program in the United States can improve understanding of adverse human health effects from exposures to radiation at doses and dose
rates of relevance to the U.S. population. In addition, this program can identify mechanisms for induction of these health effects, develop improved risk models for doses and dose rates at which direct measurement of risks is not currently possible, and ultimately develop more individualized risk estimates.