2
Fundamentals of Steel Corrosion, Industry Applications and Approaches, and Sources of Corrosion Data
Corrosion of steel is not complicated and is theoretically predictable at the electrochemical level if the steel and the environment in which the steel is placed are understood. However, at any scale in which there is unpredictable or unmeasurable variation in properties that contribute to corrosion—whether in properties of the steel itself (e.g., imperfections on the steel surface) or of the environment in which the steel is placed—the prediction of steel corrosion becomes complicated. Unpredictable spatial and temporal variations can result in severe corrosion and infrastructure failure and are found in the air that surrounds aboveground and aqueous or marine steel installations. However, the spatial and temporal variations of materials found under Earth’s surface in which steel is buried are even more complex and difficult to understand, and the steel more difficult to protect.
Before presenting information about the complexities of corrosion and corrosion prediction in later chapters, this chapter presents some fundamentals regarding steel corrosion at the electrochemical level, describes different types of buried-steel applications, discusses general industry approaches to protection of steel from corrosion, and highlights sources of data regarding corrosion of buried steel. More details about the subsurface environment and its variations are found in Chapter 3. Descriptions of different mechanisms of corrosion are presented in Chapter 4.
FUNDAMENTALS OF BURIED STEEL CORROSION
Extraction of elemental metals from ores requires the input of large amounts of energy. As such, the elemental metals and metallic alloys are thermodynamically unstable and tend to revert to stable oxides similar in form to their ores found in nature. Therefore, from a thermodynamic perspective, it is logical to expect that steel—whether buried or exposed to the atmosphere or some other environment—will corrode. The corrosion of steel buried in soil, rock, or grout occurs predominantly through an electrochemical process involving three key components: an anodic site of the steel (where the steel is electrochemically active—oxidation occurs at this site and electrons are lost), a cathodic site of the steel (a less active region—reduction occurs at this site and electrons are gained), and an electrolyte in contact with the two sites, which completes the circuit by conducting ionic current between the anodic and cathodic sites. The soil, rock, or grout in which the steel is exposed can be the electrolyte if it is sufficiently wet and conductive. The scales at which corrosion is facilitated can range from less than a nanometer to more than a meter, depending on material and environment. In other words, in essentially all instances, if steel is buried in these materials, the condition exists for corrosion to occur. The focus in this report hereafter will be on soil as the electrolyte.
At the anodic sites, iron atoms [Fe0 or Fe] are stripped of electrons to form iron ions, Fe2+:
Fe → Fe2+ + 2e– | Equation 2.1 |
This corrosion reaction causes the loss of material, and the reaction is an oxidation (or anodic) reaction because electrons are generated. It is a half-cell reaction that must be accompanied by one or more reduction (or cathodic) half-cell reactions that consume the electrons to uphold the requirement of charge conservation.1 For corrosion in wet environments, such as in moist soils, the typical cathodic reactions that occur at the cathode are oxygen reduction and hydrogen evolution reactions. If dissolved molecular oxygen [O2] is present in the soil electrolyte (oxic conditions), it can be reduced to form hydroxyl ions [OH−]:
O2 + 2H2O + 4e− → 4OH | Equation 2.2 |
The presence of oxygen will also further oxidize the dissolved Fe2+ ions to create various types of hydroxides or oxides, such as hematite, Fe2O3, that form the familiar red rust corrosion product so strongly associated with corroded steel:
4Fe2+ + O2 + 4H2O → 2Fe2O3 + 8H+ | Equation 2.3 |
If there is no dissolved oxygen in the soil electrolyte (anoxic conditions), the electrons generated by the anodic dissolution reaction can be consumed by the hydrogen evolution reaction through the reduction of water to form hydrogen gas [H2]:
2H2O + 2e− → H2 + 2OH− | Equation 2.4 |
Another form of the hydrogen evolution reaction involving the reduction of H+ ions might occur in acidic soils where these ions are in abundance:
2H+ + 2e− → H2 | Equation 2.5 |
Electrons flow through the steel between the anodic and cathodic sites on the steel, and ions released as a result of the reactions are transported through the electrolyte to complete the electrochemical circuit. The driving force for corrosion is the corrosion potential—or the measured voltage between the steel surface and the electrolyte. Box 2.1 provides a description of corrosion potential. More details of steel corrosion are provided in Chapter 4.
BURIED-STEEL APPLICATIONS
Soil and rock serve two primary but distinct functions in infrastructure construction: first, they can be used as building materials to construct geotechnical assets (e.g., embankments, dams, slopes, and some types of retaining walls); and second, they can be used as foundations to support load and to limit settlement of surficial or buried structures (e.g., building foundations or buried tanks). In the first case, soil and rock are used as construction materials, while in the second case, soil and rock are the foundation materials on or in which construction is performed. The role of buried steel will be different depending on the type of construction application. For example, when soil is used as a construction material in an earthen retaining wall, steel strips may be embedded in the soil to carry tensile load within the structure of the wall (see Figure 2.1A). This is because the tensile strength of soils is effectively zero even though soils can carry compressive and shear loading. When used as foundation materials,
___________________
1 A half-cell generally refers to one part of two half-cell readings that comprise an open circuit of corrosion potential. Different technical communities might not consider “half cell” as an acceptable equivalence to open-circuit potential, but the term is used here because it is more commonly used in the geotechnical community.
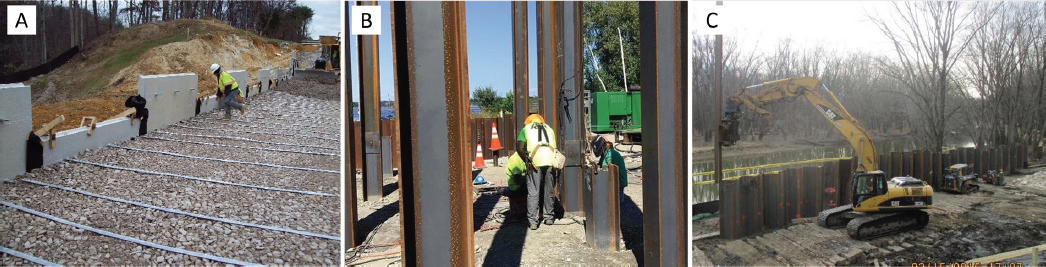
SOURCES: Photos from the (A) Reinforced Earth Company; (B) City of East Providence, Rhode Island (2021); and (C) EPA (2015).
soil and rock provide strength to prevent structure failure and stiffness to resist excessive structure settlement. For example, when a deep foundation is constructed using a steel H pile (so-called because the cross section is shaped like an “H”; see Figure 2.1B), the soil exerts resisting friction on the side of the buried pile, preventing it from settling more than specified by the design criteria. In many cases, infrastructure is designed to transmit loads exerted by the structure through steel components to the surrounding soil, which requires interaction through intimate contact between the steel and soil to prevent failure and limit settlement.
Manufactured steel components are designed with specific materials and defined shapes for desired properties. However, during exposure to the environment during service life, those properties can diminish as a result of corrosion. Most metals, including iron used in engineering applications, are refined from naturally occurring ores (i.e., oxides, carbonates, hydroxides, and silicates). The interaction between the subsurface environment (with its various solid, liquid, or gas phases) and the steel will determine the occurrence, extent, and rate of corrosion, but it is important to note that the design of the buried steel contributes to how much surface area contact there is between the subsurface and the steel and therefore how much of the steel is exposed to corrosive environments. Circular steel bars, for example, have a low ratio of surface area to volume while thin sheet piles (see Figure 2.1C) have a high ratio of surface area to volume. In contrast, the surface area contact between the subsurface and pipelines is dominantly dependent on the quality of installation and the technique used. Installation of pipelines can occur in an excavated trench where pipe will be placed in contact with backfill. This backfill can have a highly variable degree of quality control depending on the uniformity of soil compaction around the pipe and the presence or absence of air gaps on the surface of the pipe larger than the pore spaces of the soil. Alternatively, pipe can be placed through horizontal directional drilling, where it will have less uniform contact with the native soil and possibly non-native drilling mud. Table 2.1 outlines common types of infrastructure that include buried steel components.
GENERAL INDUSTRY APPROACHES TO PROTECTION AGAINST STEEL CORROSION
The committee identified two general approaches to corrosion protection applied to buried steel. These tend to drive methods of site characterization and infrastructure design but may also contribute to what the committee identifies as “silos” in practice and corrosion-related research. One approach compensates for corrosion by designing for the expected corrosion-related loss of metal over time. It is applied, for example, to structural foundations, earth retaining structures, dams, and tunnels. The committee calls this the “corrosion allowance approach” and calls those industries in which this approach is applied the “geo-civil industries.” The geo-civil industries attempt to predict corrosion rates by characterizing the corrosivity of the earth materials in which the steel is placed. The steel components are then designed with sufficient volumes of steel to compensate for expected steel loss due to corrosion over the design lifetime of the component, the application of protective coatings (e.g., galvanized zinc-coated steel reinforcing strips used in mechanically stabilized earth [MSE] walls), or both. These designs include redundancies in the system so that failure of one component does not result in catastrophic failure of the system.
The other approach to corrosion protection focuses on the prevention or reduction of the rate of corrosion through such means as coatings and cathodic protection. The oil and gas pipeline industries typically apply these approaches and tend to consider the life cycle and effectiveness of the prevention measures. Those industries that apply what this report calls the “corrosion prevention approach” are less interested in the lateral and vertical variations in corrosivity of the subsurface environment and more interested in controlling the rate of corrosion.
Not all industries or applications fit cleanly into one of these approaches, and there are exceptions within the applications described above. Some designs may use hybridized approaches, or an industry may use one or the other approach in a given application. However, this delineation of approaches is a useful way to generalize and categorize industry practices. In all cases and industries, the corrosion of buried steel is caused by the same mechanisms, and the subsurface characterization techniques used by the different industries might be combined to inform both modeling of corrosion rates and design of protective measures.
MICROORGANISMS
The presence of microorganisms and microbial activity can be anticipated on most buried steel infrastructure. The microbial community controls the chemistry at the soil–steel interface and can affect corrosion. All subsurface environments that contain water, nutrients, and energy sources in addition to electron donors and acceptors are biologically active. Essential nutrients include assimilable forms of carbon, nitrogen, phosphorus, sulfur, and trace elements. Respiration provides cellular energy through a series of coupled oxidation and reduction reactions involving the transfer of electrons from donors to acceptors. Electron donors can include organic carbon compounds (e.g., acetate, lactate, glucose), H2, or iron (Fe0). In aerobic respiration, the electron acceptor is oxygen (O2). In anaerobic respiration, electron acceptors are molecules other than oxygen (e.g., CO2) or ionic species (e.g., sulfate, nitrate, Mn+4, and Fe+3). With a given electron donor (e.g., glucose) aerobic respiration yields more energy than anaerobic metabolism.
Subsurface microorganisms can form biofilms directly on buried steel surfaces. Biofilms contain living and dead cells enmeshed in a matrix of extracellular polymeric materials and can range in thickness from a few to several hundred micrometers. A property of undisturbed biofilms in oxygenated environments is stratification of electron acceptors with depth (Nealson and Stahl, 1997). A typical progression of stratification begins with oxygen depletion and ends with sulfate reduction or carbon dioxide reduction. The reduction of sulfate produces sulfide and the reduction of carbon dioxide (i.e., methanogenesis) produces methane (Nealson and Stahl, 1997). The thickness of biofilm required to produce an anoxic environment depends on the respiration rates of organisms in the biofilm and the availability of oxygen in the environment. Aerobic respiration rates can be faster than the rate of oxygen diffusion (Yu and Bishop, 2001). In the presence of a biofilm, steel may be subject to anaerobic corrosion processes when the bulk environment is oxic. Microorganisms in biofilms do not behave as individuals. Instead, microorganisms engage in cooperative activities by responding to diffusible molecules and produce stratified communities based on electron acceptor availability.
Soils contain an enormous diversity of microbial communities that are metabolically versatile and adaptable. Soil microorganisms play crucial and often complementary roles in nutrient cycling, processes that can include biodegradation of complex carbon compounds, nitrogen fixation, and phosphorus solubilization (Barros, 2021). As an example of extreme microbial adaptation, Ortiz et al. (2021) identified microorganisms in Antarctic desert soils that were capable of harvesting solar energy, oxidizing inorganic substrates, and adopting symbiotic lifestyles.
Bacteria are the microorganisms that have received the most attention in the characterization of subsurface environments for corrosivity, but representatives from the three branches of life (bacteria, archaea, and eucaryotes [fungi]) have been identified in soils and rocks (Banciu, 2013; Bintrim et al., 1997). Microbial growth may occur on the surface of rocks or in crevices or fissures centimeters deep within the rock (endolithic) (Gorbushina, 2007). While microbial population sizes, distributions, and growth rates can be determined by the availability of water (Stevenson et al., 2015), endoliths have developed several survival strategies even in non-optimum conditions. For example, some microorganisms can survive long periods of starvation and desiccation, and their spores can remain viable for hundreds of years. In summary, microbial activity can be present in a wide range of geologic settings, and microbes are adept at adapting to different environments.
TABLE 2.1 Common Types of Infrastructure That Use Buried Steel
Type of Infrastructure | Definition and Function | Construction and Design | |
---|---|---|---|
Deep foundations | What are they? Deep foundations are long, slender infrastructure components (depth > diameter) designed to transfer loads from infrastructure to soil or rock. Used when loads cannot be sufficiently supported, when the foundation must penetrate water, or when excessive settlement would result from using shallow foundations. How common? Most bridge foundations, taller structures (>5 stories), and offshore structures incorporate deep foundations. Corrosion implications? When deep foundations corrode, they lose ability to carry load; structures tilt or collapse. |
Piles: Driven or vibrated into the ground. All piles are designed for intimate contact with soil and may bear on or into rock. Steel pile soil–steel interfacial contact area will be a function of geometry and influences corrosion. Corrosivity is addressed by increasing steel cross sections (i.e., thicker steel) or through addition of protective coating. Drilled shafts: Constructed by drilling a hole larger than 30.5 cm (12 inches) in diameter, inserting steel, and pouring in concrete. Steel is required for structural reinforcement and may corrode if the concrete cracks. Micropiles: Constructed by drilling a hole with a diameter less than 30.5 cm (12 inches), inserting steel, and pumping in grout. Steel casing may also be inserted in the upper portion of the hole and grout pumped through the center. Steel casing will be in direct contact with the soil, making corrosivity a significant design consideration that can be addressed with thicker steel casing cross sections or protective coating of steel. The reinforcement may corrode if the grout cracks. |
![]() Piles ![]() Drilled Shaft |
Type of Infrastructure | Definition and Function | Construction and Design | |
---|---|---|---|
Earth retaining structures | What are they? Designed to resist lateral load and to retain soil and groundwater. Can be rigid or flexible and are generally constructed of concrete or steel, with soil as an integral part of the design. Drainage is a critical stability consideration in most retaining structure designs—most designs include drainage of the retained soil. How common? Areas with grade changes that must be controlled for infrastructure (e.g., highway embankments, building foundation slabs). Also used for support of excavations and along waterfronts to harden the shoreline or contain reclaimed land. Corrosion implications? When steel components in earth retaining structures corrode, they lose the ability to resist lateral loads and retain soil, and structures will deform, which can lead to collapse of the structure and supported infrastructure. |
Mechanically stabilized earth (MSE) walls: Designed to use friction between the soil and long thin steel or polymeric strips that are embedded horizontally behind the wall face to prevent deformation. The strips are connected to the wall face and are designed to resist lateral movement of the wall. Corrosivity is a significant design consideration for the steel strips—often low-corrosivity soils are selected, and thicker steel cross sections are used with protective coatings (e.g., galvanized steels). Sheet piles: Driven, pushed, or vibrated thin steel sections frequently used in soft soils. Sheet piles are in intimate contact with the soil being retained. Corrosivity is addressed with thicker cross section (i.e., thicker steel). |
![]() MSE Wall ![]() Sheet Pile |
Type of Infrastructure | Definition and Function | Construction and Design | |
---|---|---|---|
Ground anchors (also known as tiebacks) | What are they? High-strength steel bars or cables used to transfer tensile loads to stabilize ground. How common? Used when additional support is needed to resist undesired movement of a retaining wall face; to uplift from tall slender structures such as towers; or to add stability for dams, tie downs, retaining walls, or foundation systems. Often used in retrofit applications. Corrosion implications? When the steel cable corrodes, it loses the ability to maintain the tensile load, which will allow undesired movement. Excessive movement can lead to failure. |
Ground anchors/tiebacks: Constructed by drilling a hole through the unstable portion of the soil or rock mass and into the underlying stable zone, inserting a steel cable or bar, and grouting in place. A steel sleeve can also be used around the steel above the grouted stable zone so the steel can be tensioned and connected to the structure. Corrosion protection is sometimes referred to as double corrosion protection since the steel is encapsulated in grout and also encased in a sleeve. | ![]() Ground Anchors |
Soil nails | What are they? Closely spaced tension-carrying steel bars grouted into soil. How common? Used as a retrofit to stabilize slopes/landslides or to add lateral support to tall excavations and retaining walls (>5 stories). Corrosion implications? When the steel corrodes, it loses the ability to maintain the tensile load, which will allow undesired movement that may cause failure. Excessive movement in a retaining wall or excavation can lead to failure. |
Solid-bar soil nails: Constructed by drilling a hole, inserting a steel bar, and pumping in grout along the length of the bar. After installation, soil nails are covered with pumpable, sprayed concrete and capped with bearing plates. The grout mitigates corrosion via encapsulation. Steel may be coated with zinc (galvanization) for additional corrosion protection. Hollow-bar soil nails: Installed in a single-step process where the bar is used to advance the hole, and grout is injected through the hollow center of the bar. Additional corrosion mitigation from galvanization (zinc coating) is not feasible due to the way the bars are installed. Corrosion is addressed by increasing the bar cross section. |
![]() Soil Nails |
Type of Infrastructure | Definition and Function | Construction and Design | |
---|---|---|---|
Rock bolts/shear pins | What are they? High-strength steel bars or cables that use tensile forces to anchor unstable rock masses. How common? Used when a slope or cut slope in a rock mass is unstable. Used as part of tunnel support systems and as roof supports in underground mines. Corrosion implications? When the steel corrodes in a section under tension, it cannot stabilize the mass and the rock face or slope will move (or fail). |
Rock bolts: Tensioned bolts for use in an unstable rock mass. Constructed by drilling a hole, inserting the steel, and pumping grout along the length of the steel. Grout is used for stability in the stable rock and to control corrosion due to water seepage. Shear pins: Untensioned pins for use in an unstable slope. Constructed by drilling a hole into the rock, and inserting and grouting a steel rod. The steel rods mitigate slip by resisting shear through their cross section but do not undergo tension that would otherwise add to a frictional resistance due to an increase in normal stress across the shear plane (i.e., shear pins offer shear resistance but do not otherwise affect the state of stress within the rock). |
![]() Rock Bolts |
Pipelines | What are they? Long, linear infrastructure networks used to transport gases and liquids great distances (in some cases thousands of kilometers). Installed by trenching or pushing the pipe into the soil from access trenches using hydraulics (i.e., pipe jacking), or by pulling pipe through a drilled hole. How common? Most water, oil, and gas are transported through a pipeline. Corrosion implications? Characterization and control of the burial environment is limited due to length. If a water pipeline corrodes, there can be a loss of structural support and transported liquid can leak. Water leaks can cause a localized loss of water pressure or remove any surrounding soil or infrastructure such as a road. Corrosion of oil or gas lines can cause loss of structural support or a catastrophic failure, sometimes leading to environmental damage or an explosion. |
Water lines: Primarily manufactured from cast or ductile iron. Corrosion is addressed by increasing the cross section of the pipe (i.e., steel thickness) based on the soil corrosivity. Oil or gas lines: Primarily manufactured from plain low-carbon steel. Coatings and cathodic protection are used across pipeline systems to address corrosion. |
![]() Pipeline |
Type of Infrastructure | Definition and Function | Construction and Design | |
---|---|---|---|
Underground storage tanks | What are they? Cylindrical or rectangular prisms or other shaped steel containers used for storing liquids such as water and fuel. How common? Not as common as other types of buried steel. Common application has been at gas stations but is becoming less frequently used compared to inert polymer and plastics. Corrosion implications? Corrosion can result and leakage, depending on the type of liquid, can result in environmental contamination including groundwater. |
Underground storage tanks: Fuel and water storage tanks are made of ductile iron or mild steel. Tanks are generally made by welding steel plates together to form the desired shape of the tank. | ![]() Underground Storage Tank |
SOURCE: Diagrams of piles, drilled shafts, MSE walls, sheet piles, ground anchors, soil nails, and rock bolts from Keller Management Services, LLC (2022).
SOURCES OF CORROSION DATA
Long-term in situ testing to understand and measure the corrosion of buried steel is limited, but the most well-known studies were performed from 1922 to 1940 by the National Bureau of Standards (NBS, now National Institute of Standards and Technology) (Logan, 1945; Romanoff, 1957). In these studies, samples of uncoated alloy steel and wrought iron (i.e., low-carbon steel) were buried at 47 locations throughout the United States. The locations were chosen to test corrosion of steel in a range of soil types and climates. To mimic real-world conditions, the long-term experiments performed by NBS were designed to test steel in field conditions with no control or alteration of the soil properties. The steel was allowed to corrode in nonuniform soil conditions. Interpretations of the resulting data indicate that corrosion rates typically attenuated with respect to time, depending on the subsurface conditions.
The NBS studies had several experimental design limitations. Soil environments were not thoroughly characterized, burial depths and exposure times were variable, exposure to moisture was not monitored, rainfall was not quantified, climatic variables were reported as averages, many soil properties were measured off-site, and the statistical design of the study was weak (de Arriba-Rodriguez et al., 2018). Despite experimental limitations, the NBS data still are the basis for steel corrosion rate predictions today. It is reasonable to conclude that the high level of uncertainty associated with the experimental data could lead to either costly overconservative design or unsafe underconservative design.
Since completion of the NBS studies, others have studied the performance of buried steels and archived data from specific applications (e.g., related to MSE walls, piles, ground anchors, rock bolts, and culverts). Some of those studies are described in the list below, and data from them may be used to better understand the reliability of existing corrosion models for estimating service life or the factors that contribute to corrosivity in different applications. Although these studies are not as comprehensive as the NBS efforts, the resulting data supplement the NBS data and are applicable to specific sets of conditions that were not addressed in the NBS study. For example, less than 10 percent of the NBS data were from free-draining granular soils such as those used in constructed earth applications. Even fewer of the data are related to corrosion of galvanized steels. Below are some additional sources of data that supplement the NBS database.
- Corrosion of metallic earth reinforcements in MSE wall backfill is addressed with a 20-year study by Darbin et al. (1986). NBS data (Romanoff, 1957) on the performance of MSE reinforcements were supplemented by industry research (Darbin et al., 1986; Haïun et al., 2007), by research of proprietors of MSE wall systems in Europe (France) beginning in the 1970s, and with results from recent research sponsored by the National Cooperative Highway Research Program (Fishman and Withiam, 2011).
- Corrosion, durability, and service-life modeling for steel piles including steel (mostly), reinforced concrete, and prestressed concrete piles have been described by Schwerdtfeger and Romanoff (1972), Statfull and Seim (1979), Ohsaki (1982), Long (1992), Long et al. (1995), Beavers and Durr (1998), Törnqvist and Lehtonen (1999), Wong and Law (1999), Decker et al. (2008), Sagues et al. (2009), Gu et al. (2015), and Poursaee et al. (2019). (Note that reinforced concrete and prestressed concrete piles are not covered in the present report.)
- Data related to the corrosion of various types of steel piling exposed to underground conditions at 53 different sites are included in NBS Monograph 127 (Schwerdtfeger and Romanoff, 1972). The steel was buried in climate ranging from semitropical to frigid, above and below the water table, and in soils encompassing a broad range of corrosivity.
- Data related to pile performance from seven sites located in New York, Connecticut, Maryland, North Carolina, and Mississippi were collected by Beavers and Durr (1998). In all cases the subsurface was layered, and corrosion observed along the piles was confined to one of the layers. The highest corrosion rates were observed within non-natural fills such as ash, slag, and cinders where the interface between native soils and non-natural fills was at or near the water table. The results reported by Beavers and Durr
- Data from four cases where severe corrosion of steel piles advanced through industrial fill including slag and cinder ash with groundwater near the base of the fill are reported in Connecticut (Long et al., 1995), in New York (Moody, 1993), in Pennsylvania (Gu et al., 2015), and by the Wisconsin Department of Transportation (WisDOT, 2015). In cases where piles have not been advanced through industrial or engineered material, the observed corrosion is low.
- Corrosion rates and predictions based on the long-term field performance of piles in Utah were evaluated by Decker et al. (2008). Twenty piles were extracted from five sites after service lives of 34–38 years. Measurements included soil index properties, resistivity, pH, cation/anion concentrations, and water table elevation.
- The possibility of correlating water pH, soil resistivity, and soil corrosion potential with the durability and corrosion of culverts was investigated by Boyd et al. (1999). Fifty-one sites with a variety of culvert types were included in the study. Variables including soil resistivity, flow rate, and streambed geometry were measured, and descriptions of the pipe slope, presence and speed of water, streambed type, and other parameters were recorded.
- Possible correlations among bed load, water velocity, corrosion rate, culvert installation design, and site conditions are described by Ault and Ellor (2000). They studied the durability of corrugated (mostly aluminized Type 2; see Chapter 3) metal pipe. Field and laboratory studies included 32 culverts in three states. The effects of a number of different parameters were investigated, including pipe size, slope, and corrugation type; soil temperature, resistivity, pH, and abrasiveness; and water temperature, resistivity, pH, chemistry, and flow rate.
- Studies on the corrosion of ground anchors, rock bolts, and soil nails are reported by the National Cooperative Highway Research Program (Withiam et al., 2002), the Fédération Internationale de la Précontrainte (1986), the New Hampshire Department of Transportation (Fishman, 2005), the New Brunswick (Canada) Department of Supply and Services (Snyder et al., 2007), the Washington State Department of Transportation (Kramer, 1993), the Federal Highway Administration (Cheney, 1988), the Texas Department of Transportation (Briaud et al., 1998; Gong et al., 2006), and the Geotechnical Engineering office of Hong Kong (Shiu and Cheung, 2008), and for sites in Japan (Tayama et al., 1996) and Scandinavia (Baxter, 1996; Lokse, 1992). Other studies were conducted by Sundholm (1987).
- Reported incidents of ground anchorage failures caused by corrosion compiled by the Fédération Internationale de la Précontrainte (1986) were summarized by Littlejohn (1992). Thirty-five case histories comprising 24 failures of permanent installations are included. Based on the results of reported incidents relative to the number of anchors installed during the 31-year time frame covered by the survey, the incidents of prestressed ground anchor failures by corrosion were limited and generally random, with the possible exception of steel-type (grade, manufacturing process, etc.). Corrosion was localized and appeared irrespective of tendon type (i.e., bar or strand). Corrosion generally occurred where the tendon steel intersected a crack in the protective grout. Cracks in the free and fixed anchor zone were formed either as the result of shrinkage strains due to curing, or the result of tensile loading.
- Data on rock bolt performance, mostly from underground mining and tunneling applications in Scandanavia, Australia, Canada, and the United States are summarized by Kendorski (2000). Kendorski compared performances considering the effects from different grouts used to install the anchorages, and for different types of rock bolt and ground anchor systems.
demonstrate how site conditions in addition to the electrochemical properties of the earthen materials have a significant effect on corrosivity.