7
The Built Environment
ABSTRACT
The planning, design, construction, maintenance, and disposition of the built environment creates more than 40 percent of U.S. energy demand and resulting greenhouse gas (GHG) emissions. From our individual homes, workplaces, and institutions through neighborhoods and greater metropolitan and regional geographies, the built environment’s demand for energy continues to evolve in complex ways. A broad range of public policies and private actors make decisions fundamental to the built environment that, in turn, define how, how much, and what kind of energy is consumed. The overall reduction of total energy demand in the built environment through energy-efficiency improvements and advanced energy management is as critical for U.S. decarbonization as the elimination of carbon-based energy directly used by them through building electrification and property-level renewable energy installations. The execution of these integrated building-level decarbonization actions alongside community-scale interventions such as mixed-use, transit-oriented redevelopment, and shared renewable power generation could be transformational.
Most of these actions are well within the current technological capacity and knowledge of the industries that support new and existing buildings. Movement along the decarbonization path has indeed accelerated since the committee’s first report owing to new funding and supportive policy at all levels of government, not the least of which are the 2021 Infrastructure Investment and Jobs Act (IIJA) and the Inflation Reduction Act of 2022 (IRA). However, further opportunity for decarbonization exists in the built environment, well beyond the goals that underpin these policies, and more aggressive targets for the built environment’s decarbonization are feasible.
The immediate challenges lie in these policies’ implementation. This holds particularly true of their primary use of voluntary, financial incentives that will require extensive coordination with existing private service providers, owners and occupants of buildings that must be harnessed and sustained beyond their course over the next decade. To succeed, there needs to be clarity and deep coordination in federal, state, and local building programs, with a commitment to ensuring uniform access, minimal burden on both providers and households, and diligent monitoring of take-up across all states. The current incentives should catalyze the institutional changes—the
technologies, workforce, industrial organization, owner demand, and, where needed, sufficient household assistance—that will facilitate the move to more regulatory policies for eliminating carbon-based demands in the built environment.
The committee identified immediate actions regarding the implementation of recent investments to ensure that the next decade’s targets are met, including promoting equity and access to the benefits availed under the incentives within the IRA and working to improve and expand programs such as the Weatherization Assistance Program. Deep conservation and innovations at the building to community level are needed to support the strategic integration of the building sector with the emerging renewable electric grid. For this to succeed, there needs to be clarity and deep coordination in federal, state, and local building programs with increased regulatory rigor for both efficiency and electrification in buildings, appliances, and equipment. There is an equally critical need for national investment in increased research, development, demonstration (RD&D), and commercialization of building technologies for homes, commercial buildings, and grid-interactive efficient buildings (GEBs) with distributed renewables and storage. Last, there is the transformational pursuit of community-level decarbonization of buildings and community infrastructures that integrate buildings more comprehensively with transportation systems and the grid.
INTRODUCTION
The committee’s first report highlighted the built environment of the United States—the millions of individual homes, offices, schools, and other facilities as well as the communities and infrastructure that serve and connect them. Although not separately analyzed for its decarbonization potential, the sector was the subject of numerous recommendations. Movement along that path has accelerated since the first report’s publication owing to new public funding and supportive policy at all levels of government, not the least of which are the IIJA, the IRA, and their combined federal investment in the electrification and energy-efficient improvement of our residential and commercial building stocks, the largest component of the stationary built environment’s contribution to GHGs by far. Combined with the current administration’s presumed targets for the built environment’s decarbonization by 2050, simply put, the goal posts have moved. Consequently, the committee provides three overarching observations in relation to these recent changes in addition to specific recommendations and the individual findings that are enumerated in this report.
First, the committee is very conscious of the pivotal moment in building decarbonization in which the nation finds itself, but also that the transformation that is asked of our buildings is largely voluntary and market based. It requires extensive coordination
with existing private providers of building services. However, that coordination must be sustained and formalized beyond the next decade. The practices established now must be based on solid principles of equity, efficiency, and effectiveness. Therefore, the committee’s first recommendations center on the implementation of recent investments to ensure that the next decade’s targets are met.
- Recommendation 7-1: Ensure Clarity and Consistency for the Implementation of Building Decarbonization Policies.
- Recommendation 7-2: Promote an Equitable Focus Across Building Decarbonization Policies.
- Recommendation 7-3: Expand and Evaluate the Weatherization Assistance Program.
- Recommendation 7-4: Coordinate Subnational Government Agencies to Align Decarbonization Policies and Implementation.
Second, the committee explicitly calls for approaching the built environment’s decarbonization through both the reduction of carbon-based fuels that are directly consumed in building (a shift to electrification powered by renewables) as well as the range of interventions that reduce the overall demand for energy production. Reducing overall demand ensures that energy production decreasingly relies on any carbon-based sources, but also promotes management of a clean electricity grid.
This approach begins with improving all buildings’ energy efficiency. The committee referenced this multi-pronged strategy in the first report but provides more detail about the range of building interventions that are possible and whose sum are greater than the individual parts that are proposed in contemporary efforts. Additional recommendations ensure that this range of interventions are central to the mid- and long-term plans for building decarbonization.
- Recommendation 7-5: Build Capacity for States and Municipalities to Adopt and Enforce Increased Regulatory Rigor for Buildings and Equipment.
- Recommendation 7-6: Increase Research, Development, Demonstration, and Deployment for Built Environment Decarbonization Interventions.
Third, the committee believes that more aggressive actions are needed and possible from buildings than what is projected in the original report’s targets, what is enabled with IRA funds, and what is stipulated even under current White House ambitions. The building industry’s proven capacity to adapt, combined with the urgent co-benefits to occupant health and reduced cost burdens as well as community economic development, demand that our nation’s decarbonization strategy center the built environment more squarely.
To that end, one additional strategy is needed to ensure that the lessons of this first decade in comprehensive building carbonization are sustained.
- Recommendation 7-7: Extend Current Decarbonization Incentives Beyond the Next Decade While Scaling Up Mandates.
Given the significant portion of our GHG emissions that come from both the direct combustion of natural gas and other carbon fuels in our buildings and in the electricity sources that power them, the committee expands on the earlier recommendations by identifying the gaps between current conditions and the first report’s targets as well as the estimated contributions from recent policy. This report describes the range of physical interventions in the built environment that present decarbonization opportunities, arguing for a nuanced approach to policy and program priorities between them. Last, the report sheds light on the physical, industrial, and social parameters of the built environment’s energy demand that shape the implementation of recently passed legislation as well as the committee’s recommendations, as these structures will define how midcentury decarbonization goals will be met in this sector. Table 7-8, at the end of the chapter, summarizes all the recommendations that appear in this chapter to support decarbonizing the built environment.
SUMMARY OF CURRENT GOAL SETTING
Several proposals have been put forth regarding the built environment’s capacity to decarbonize and, consequently, quantified targets for this vision, over the past decade. Two are most relevant to the committee’s current considerations: (1) the committee’s last set of recommendations in 2021 that are mirrored in recently enacted public policy, and (2) the more recent goals defined by the Biden administration in 2022 for keeping with international promises for the nation’s GHG reductions. Both included the built environment as sectors to be decarbonized within a larger economic and material transition, but to variable degrees of magnitude and urgency.
2021 Committee Goals
The committee’s first report addressed the contribution from the built environment to the national decarbonization vision at different levels of policy magnitude. These included the broad goal of reductions in total energy consumption (not exclusively carbon-based energy consumption) as well as detailed heat pump adoption targets (as the largest technological opportunity for both electrification and efficiency gains in the building sector). These goals and targets included variably quantified outcomes.
Specifically in relation to overarching goals at the time, the report recommended the reduction of overall energy consumption in existing buildings by 3 percent per year over the next decade to achieve a 30 percent total reduction, and in new construction by 50 percent total, both by 2030. As described in the report, the strategy for meeting these
targets combines incentives for private-sector voluntary actions as well as mandates for new construction and equipment (the latter primarily for buildings supported with public funding). Beyond the first decade, the committee provided a broad range of long-term goals that also differentiate between new buildings (designated to be carbon-neutral by 2050) and existing ones (to have 50 percent reductions in overall energy demand by 2050). In short, the higher-order goals target overall energy demand as opposed to the carbon-based energy sources that currently fill that demand.
Last, the committee provided specific measurable targets for one strategy toward these decarbonization goals. The primary technological vehicle for reaching the report’s targets is the electrification of space and water heating; the report set a target of increasing electric heat pumps’ share of heating and hot water equipment to 25 percent in residential buildings and 15 percent in commercial buildings by 2030 (NASEM 2021a, p. 6). This specific intervention is particularly strategic given that heat pumps bring important benefits from reducing carbon-based fuel dependence and indoor air pollution for occupant health and from enhancing electrical grid management and optimization (owing to the digitalization of electrical information and energy-efficiency of new equipment). The recommended enabling policy to support this target is in the form of national equipment and appliance standards with increasing requirements for their electrification.
The committee also recommended a few other policy actions to assist in meeting the 2030 targets, including expanding funding for the Weatherization Assistance Program (WAP) and including electrification requirements within the program’s eligible activities; enforcing efficiency standards on federally owned buildings; and encouraging more RD&D across a range of building interventions, such as building benchmarking, district and combined building energy, building-level energy storage, and embodied carbon in construction materials.
When taken in aggregate, the first report encouraged building electrification policies that reduce and eventually eliminate carbon-based fuel consumption directly in U.S. buildings, while also acknowledging the critical role that increasing energy efficiency requirements of new buildings and expanding energy-efficient retrofits in existing ones have had for reducing the overall demand for energy and, in turn, reducing the magnitude of carbon-based energy needs. However, although the report addressed a range of technological interventions that fall within the built environment’s realm, it does not prioritize or assign quantitative targets for each intervention’s contributions to decarbonization.
Finding 7-1: The first report’s recommendations for the built environment’s decarbonization were multipronged, with overarching goals and specific activity targets aimed at different challenges and variable actions. The overall reduction
of buildings’ total energy demand is as critical for comprehensive decarbonization as the elimination of carbon-based fuels that are directly used in buildings—the former generating benefits in equitable building transitions, grid management in the built environment, and overall energy demand beyond buildings.
2022 Federal Goals
The reentry of the United States into the global climate treaties collectively known as the Paris Agreement in January 2021 launched a reconsideration of the magnitude of ambition in the national decarbonization efforts and each sector’s contribution to it.1 The goal posts moved significantly. In April of 2021, the nation committed to reducing net GHG emissions to 50 to 52 percent below 2005 levels by the year 2030 and to zero by 2050 (DOS and EOP 2021).
For the building sector, the policy commitment combines the multi-pronged strategies of energy efficiency and electrification recommended by the committee with consequent, critical interventions such as digitalization. Equity, mainly with regard to building decarbonization’s costs, is noted as part of this vision and moved forward through the implementation of Justice40 across the federal government (see Chapter 2 for more details on Justice40). Policy actions like RD&D funding, support for increasingly rigorous codes and standards, and investments in the public building stock add to the approaches for realizing the vision.
However, the ambition for the built environment’s decarbonization is more muted than for other sectors, and less ambitious than the committee’s prior (and achievable) 30 percent reduction goal. Emissions from buildings have already been falling since 2005 owing largely to increases in energy efficiency, even though both the number and size of residential and commercial buildings have continued to increase. The potential for much deeper emissions reductions is large. More aggressive actions are needed to ensure that such reductions are tapped.
Finding 7-2: The White House’s 2022 projections for each sector’s decarbonization understate buildings’ decarbonization magnitude and pace. As a leading contributor to GHG emissions from both direct carbon-based fuel use and as an end use for utility-provided electricity that relies on carbon-based fuels, the built environment has shown demonstrable transformation over the past half-decade and could, technologically and economically, transform further.
___________________
1 This chapter considers the current administration’s proposal as the overarching update to the committee’s 2021 goals as described in The Long-Term Strategy of the United States: Pathways to Net-Zero Greenhouse Gas Emissions by 2050 (DOS and EOP 2021).
GAPS
The built environment directly consumes a significant amount of energy, most of which continues to derive from carbon-based sources. The aggregation of buildings and their spatial placement has historically relied on carbon-based energy sources directly (50 percent of residences and 47 percent of commercial buildings use oil or natural gas energy directly) and through electric power production (adding another 25 percent of residences and 30 percent of commercial facilities that rely on electricity produced through fossil fuel or coal combustion). When including the electricity transmission for their consumption, buildings account for the largest end-use share (35 percent) of total U.S. GHG emissions of any sector (EIA 2022a,b). (See Chapter 8 for a discussion on emissions from construction material extraction and production.)
Current Energy Use
Consequently, the residential and commercial building sector accounted for more than 40 percent of total energy end uses last year, including electrical generation, transmission, and losses, as well as direct energy uses in buildings, or almost 23 quadrillion BTUs, according to the Energy Information Administration (EIA 2022c). Through direct combustion alone, buildings consumed an aggregate 7.2 quadrillion BTUs in energy from natural gas, heating oils, and other carbon-based fuels according to recent consumption surveys, almost two-thirds of it coming from the housing stock and the remainder from commercial buildings (Table 7-1). Buildings, in short, matter.
Comparing the White House’s 2021 long-term national targets (DOS and EOP 2021) with the current energy demands of the built environment provides important perspective. Projections of building decarbonization to support the national goals suggest that the sector’s emissions would be reduced to 0.4–0.5 gigatons from the 0.6 gigatons at 2005 levels by 2035 and to 0.1–0.3 gigatons by 2050 (DOS and EOP 2021, Figures 4 and 9). The reductions stem in part from the reductions in carbon-based fuels used directly in buildings, as they electrify and increase efficiency, presumed to decrease from approximately 11 exajoules in 2005 to 7.5–9 exajoules (an 18–32 percent reduction, or the equivalent of saving 2–3 quadrillion BTUs) by 2035 and to 2–6 exajoules by 2050 (or 45–82 percent reductions, or 5–8.5 quadrillion BTUs saved).
If the lower range of these values were achieved, however, fully 2.2 quadrillion BTUs of carbon-based fuels would still be used directly in buildings by 2050—almost one-quarter of what is currently used. This sizable, persistent use of carbon fuels in buildings is neither aligned with the transformations proposed for other sectors, nor is
ABLE 7-1 Recent Annual Total and Building Average Primary Energy Consumption
Number of Buildings (thousands) | Energy Sources (millions of BTUs) | ||||
All Energy Sources | Electricity | Natural Gas and Fuel Oil | Other Source | ||
2015 Residential | 118,200 | 9,114,000,000 | 4,324,000,000 | 4,790,000,000 | — |
Building average | 118,200 | 77 | 37 | 34 | — |
Recent average | 3,800 | 67 | 40 | 27 | — |
2018 Commercial | 5,918 | 6,807,000,000 | 4,090,000,000 | 2,401,000,000 | 316,000,000 |
Building average | 5,918 | 1,150 | 691 | 406 | 53 |
Recent average | 537 | 1,359 | 885 | 439 | 35 |
NOTES: Averages for energy source types in buildings derived across the entire building stock (not by buildings that use an individual type solely). Most recent = 2010–2018 commercial buildings; 2010–2015 residential. Other sources include district heating. Primary electricity and all energy consumption numbers do not include transmission losses. SOURCE: Tabulations of 2015 Residential Energy Consumption Surveys and 2018 Commercial Buildings, courtesy of the U.S. Energy Information Administration, May 2018 and December 2022 (EIA 2018, 2022a).
it compatible with the usual functional life cycle of energy-consuming equipment and appliances in buildings or the turnover in building ownership.2
To reach a more aggressive outcome for transforming individual buildings, each with its own owners and occupants, construction qualities, and local building markets, significant implementation challenges must be overcome. Reducing buildings’ energy use requires both eliminating their direct use of fossil fuels toward electric appliances and equipment alongside deep efficiency improvements and energy management innovations.
For the former objective, the gap between current consumption and the desired reduction is formidable. Over half of all commercial buildings and almost two-thirds of homes currently combust natural gas, fuel oil, or propane solely or in some combination directly in buildings. In absolute terms, this translates to almost 3 million commercial buildings and almost 75 million homes using just natural gas currently that would need to be electrified to meet national net-zero decarbonization goals by midcentury (Table 7-2). Like other sectors, fortunately, buildings
___________________
2 For example, the average life cycle of a natural gas space-heating furnace is 15 to 20 years, and the current median duration of home occupancy is 13 years, per DOE technical monitoring (Energy Star Undated) and census estimates (NAR 2020). In theory, homes could have two system replacements and two new owners in the 3 decades before 2050.
ABLE 7-2 Building Counts by Energy Source and Year of Construction (millions of buildings)
All Buildings | Electricity | Natural Gas | Fuel Oil | Propane | Wood, Coal, Other | District Heat | District Chilled Water | |
Residential | 123.53 | 123.53 | 74.65 | 5.72 | 11.68 | 10.83 | — | — |
Before 1950 | 20.26 | 20.26 | 15.28 | 1.87 | 1.83 | 1.75 | — | — |
1950 to 1959 | 12.48 | 12.48 | 9.09 | 1.06 | 0.78 | 0.80 | — | — |
1960 to 1969 | 12.76 | 12.76 | 8.72 | 0.72 | 0.98 | 1.06 | — | — |
1970 to 1979 | 18.34 | 18.34 | 9.76 | 0.76 | 1.65 | 2.01 | — | — |
1980 to 1989 | 16.30 | 16.30 | 8.10 | 0.55 | 1.37 | 1.89 | — | — |
1990 to 1999 | 17.16 | 17.16 | 9.30 | 0.42 | 2.08 | 1.68 | — | — |
2000 to 2009 | 16.16 | 16.16 | 8.97 | 0.31 | 1.91 | 1.20 | — | — |
2010 to 2015 | 5.53 | 5.53 | 2.93 | 0.03 | 0.58 | 0.24 | — | — |
2016 to 2020 | 4.56 | 4.56 | 2.49 | — | 0.49 | 0.19 | — | — |
Commercial | 5,918 | 5,613 | 2,974 | 583 | 676 | 180 | 86 | 55 |
Before 1920 | 329 | 323 | 184 | 54 | 49 | — | — | — |
1920 to 1945 | 379 | 368 | 172 | 52 | 65 | — | 11 | 3 |
1946 to 1959 | 517 | 496 | 310 | 56 | 52 | — | 20 | — |
1960 to 1969 | 685 | 673 | 420 | 57 | 61 | — | 14 | 10 |
1970 to 1979 | 831 | 787 | 400 | 87 | 115 | 25 | 8 | 6 |
1980 to 1989 | 794 | 752 | 372 | 69 | 85 | — | 6 | 4 |
1990 to 1999 | 921 | 844 | 412 | 73 | 113 | — | 8 | 10 |
2000 to 2009 | 924 | 860 | 454 | 94 | 91 | — | 6 | 6 |
2010 to 2018 | 537 | 508 | 250 | 41 | 45 | — | 4 | 4 |
NOTES: Rows do not sum, as multiple sources may be used in individual buildings. Approximately 3.7 percent of residential buildings and 1.8 percent of commercial buildings have property-level solar installed as part of their electric source (EIA 2022a,b). SOURCE: Tabulations of 2018 Commercial Buildings and 2020 Residential Energy Consumption Surveys, courtesy of the U.S. Energy Information Administration, December 2022 and May 2022 (EIA 2022a,b).
have undergone a significant although gradual internal transformation over the past half-century.
Increasing electrification and consequent reductions in carbon-based fuels have been the long-term trend, albeit a slowly realized one. For example, 75 percent of homes built before 1950 currently rely on natural gas compared to 55 percent of the homes built more recently. Although 60 percent of commercial buildings built before the mid-1900s rely on natural gas, that share is about 47 percent in the buildings built in the past decade (see Table 7-2). Continuing the trend toward full electrification in new buildings along with a massive transformation for electrification within existing buildings is needed to reach net-zero carbon goals solely within their operations—that is, before considering electrical sources and transmission.
Regarding the second objective of decreased total energy consumption, new commercial and residential buildings are built to stricter energy performance requirements, using more efficient equipment and appliances and often with their own energy production potential via distributed, building-level renewable energy installations. Consequently, the average energy consumption (and certainly the energy intensity) for the most recent additions to the building stock tend to be lower than their older counterparts. (See Table 7-3 for average residential energy consumption for different energy sources by year of construction.)
Efficiency improvements in newer building construction over time, however, are offset by two other realities that affect overall energy use and GHG emissions. First, the number of buildings continues to increase, mirroring national demographic and economic growth. Second, the average size of new buildings has been increasing in both commercial and residential construction. Consequently, both primary energy use and total consumption from the sector’s two building stocks have leveled over the past 2 decades (Figure 7-1).
Because neither of these trends is expected to change, the ability to further decarbonize buildings requires an intricate surgery to (1) gradually remove the primary use of natural gas, heating fuels, and other carbon-based sources—that is, electrify; and (2) further reduce the overall energy demand through efficiency improvements, grid management, distributed electricity production, and other electric grid management strategies. These approaches are required for both existing and new construction. Because of the magnitude of these challenges, targeting the largest end uses in buildings of both primary carbon-based fuel consumption as well as the overall largest uses of electric energy provide strategic opportunities for immediate reductions.
TABLE 7-3 Average Annual Residential Energy Consumption by Year of Construction, 2015
Year of Construction | All Fuels | Electricity | Natural Gas (millions of BTUs) | Propane | Fuel Oil/Kerosene |
---|---|---|---|---|---|
Before 1950 | 88.7 | 30.1 | 65.3 | 34.5 | 68.7 |
1950 to 1959 | 84.4 | 31.7 | 60.3 | 26.9 | 79.9 |
1960 to 1969 | 75.0 | 32.5 | 53.9 | 26.3 | 63.2 |
1970 to 1979 | 70.3 | 36.7 | 52.2 | 28.0 | 64.3 |
1980 to 1989 | 65.7 | 37.5 | 48.0 | 25.8 | 58.6 |
1990 to 1999 | 78.3 | 42.3 | 60.2 | 29.8 | 62.0 |
2000 to 2009 | 78.2 | 43.8 | 59.1 | 40.9 | 63.6 |
2010 to 2015 | 67.0 | 39.8 | 51.7 | 31.2 |
NOTES: The “All Fuels” category includes consumption for biomass (wood), coal, district steam, and solar thermal, which are not represented in individual columns in this table. Electricity consumption from on-site solar photovoltaic generation (i.e., solar panels) is included. SOURCE: Calculated using data from Table CE2.1, “Annual Household Site Fuel Consumption in the United States—Totals and Averages,” in the 2015 Residential Energy Consumption Surveys, courtesy of the U.S. Energy Information Administration, May 2018 (EIA 2018).

Technology Gaps and Transitions: The Example of Heat Pump Adoption
The first report’s recommendation to focus on space and water heating as the largest carbon-based fuel end uses in commercial and residential buildings and its subsequent integration into national policy as noted later in this chapter, then, is well founded.3 Approximately 69 percent of all carbon-based energy consumed in homes is used for space heating, 24 percent for water heating, and another 3 percent for cooking (Table 7-4). These end uses dominate consumption of most energy sources except for fuel oil (which includes kerosene), 86 percent of which is used for space heating and 13 percent for water heating. The shares of carbon energy sources in commercial buildings for space and water heating are comparable—for example, 70 percent of the 2,974 commercial buildings that use natural gas use it for space heating.
The committee’s focus on heat pumps as the vehicle for electrification of current space and water heating’s energy consumption is also supported by the evidence, given current technological options for immediate electrification and the financial feasibility of their installation. The gap between heat pumps’ diffusion and the rates of this technology’s adoption that would be necessary to meet the stated decarbonization goals is significant. For example, approximately 673,000 commercial buildings currently use heat pumps as primary heating equipment (or 11.4 percent of commercial buildings) (EIA 2022a). This leaves less than a 4 percentage-point gap between current conditions and the committee’s original targets for commercial building diffusion. If 2022 construction rates hold, half of all new buildings would need heat pumps to meet the 15 percent target by 2030.
The residential sector demonstrates similar challenges. At last count, slightly more than 14.4 percent of homes use electric heat pumps, posing a more than 10 percentage-point gap from committee targets by 2030 (EIA 2022b) (Table 7-5).4 This share represents a significant increase of residential buildings from 5 years prior, when only 10 percent of homes contained heat pumps (EIA 2018). In fact, new homes constructed in the past 5 years for which data are available show a diffusion rate of almost 24 percent—or only 1 percentage-point away from committee targets (NASEM 2021).
___________________
3 The White House goals do not specify the technologies that would need to be adopted in order to meet the reduction targets in buildings. However, the IRA incentivizes a range of energy-efficiency and electrification technologies, with electric space heating and cooling heat pumps receiving the largest incentives by dollar value.
4 This count does not include ductless heat pumps, commonly called “mini-splits,” which would increase the current share to 15.3 percent of all homes. It does not include natural gas heat pumps.
TABLE 7-4 Aggregate Residential Energy Consumption by Source and End Use, 2015
Total Consumption | Electricity | Natural Gas | Fuel Oil | Propane | |
Total | (trillion BTUs) | ||||
4,790 | 4,324 | 3,965 | 464 | 361 | |
Space heating | 3,307 | 638 | 2,678 | 397 | 234 |
Water heating | 1,154 | 590 | 1,019 | 59 | 76 |
Space cooling | 731 | 731 | — | — | — |
Cooking | 132 | 62 | 113 | — | 19 |
Clothes drying | 39 | 196 | 36 | — | 3 |
Other | 156 | 1,804 | 119 | 8 | 30 |
Average | (million BTUs) | ||||
77.1 | 36.6 | 57.8 | 67.3 | 31.2 | |
Space heating | — | 11.1 | 45.0 | 59.9 | 31.0 |
Water heating | — | 10.7 | 18.1 | 20.7 | 17.8 |
Space cooling | — | 7.1 | — | — | — |
Cooking | — | 0.8 | 2.9 | — | 3.1 |
Clothes drying | — | 2.6 | 2.1 | — | 2.4 |
Other | — | 16.6 | 0.9 | 24.4 | 1.9 |
NOTE: Averages are based on population of homes using each energy type as a primary source for an end use and therefore do not sum across energy types. SOURCE: Tabulations of 2015 Residential Energy Consumption Surveys, courtesy of the U.S. Energy Information Administration, May 2018 (EIA 2018).
If all new homes were required to have heat pumps, the housing stock would meet the committee’s heat pump diffusion rate targets by 2028, assuming the average construction rates of approximately 1.2 million new homes built each year continues (U.S. Census Bureau 2023). However, requirements that all new construction be built to certain specifications are not always viable financially or logistically, nor are mandates without incentives politically viable. They also do not catalyze the industry to retrofit the existing building inventory—an industrial transformation that is needed to meet long-term decarbonization goals beyond 2030.
TABLE 7-5 Share of Homes with Electric Heat Pumps by Year of Home Construction over Time
Year of Home Construction (percentage of home age group) | ||||||||||||
---|---|---|---|---|---|---|---|---|---|---|---|---|
Year of Observation | Total Share | Before 1950 | 1950 to 1959 | 1960 to 1969 | 1970 to 1979 | 1980 to 1989 | 1990 to 1999 | 2000 to 2009 | 2010 to 2015 | 2016 to 2020 | 2020 to 2030 | 2030 to 2040 |
2009 | 8.6% | 3.1% | 3.7% | 5.3% | 8.7% | 11.8% | 13.4% | 14.1% | — | — | — | — |
2015 | 10.0% | 1.9% | 7.1% | 7.8% | 9.8% | 14.4% | 14.3% | 14.1% | 13.2% | — | — | — |
2020 | 14.4% | 5.5% | 8.4% | 11.3% | 14.2% | 18.4% | 19.1% | 18.8% | 21.0% | 23.7% | — | — |
2030 (est.) | 20.6% | 7.7% | 12.7% | 16.8% | 19.1% | 24.4% | 24.2% | 23.1% | 40.0% | 45.2% | 25% | — |
2040 (est.) | 25.8% | 9.9% | 17.0% | 22.2% | 24.1% | 30.5% | 29.3% | 27.4% | 59.1% | 66.7% | 25% | 25% |
NOTES: The committee’s estimates assume identical annual increases in rates of replacement for homes built before 2015 based on the 2009–2020 average and a consistent adoption rate of 25 percent for new homes built after 2020. New home counts after 2020 assume 1.2 million completions annually. SOURCES: Data from 2009, 2015, and 2020 Residential Energy Consumption Surveys, courtesy of the U.S. Energy Information Administration, May 2013, 2018, and 2022 (EIA, 2013, 2018, 2022b). Data for estimates of 2030 and 2040 heat pump adoption rates and housing units were committee generated.
Existing buildings’ retrofitting will be the critical vehicle for the sector’s decarbonization. As such, another important consideration from the review of past diffusion rates is the fact that replacement rates of heating equipment across the inventory of older building stock are also trending toward electric heat pumps—that is, not all gains in heat pumps’ diffusion are from new construction. For example, almost 12 percent, or about 2 million homes, built in the 1980s had heat pumps over a decade ago. Now, 18.4 percent of the same homes have heat pumps—or about 3 million homes (see Tables 7-2 and 7-5). The transformation is notable because equipment in homes built in that decade will have completed its functional life and require replacing.
If electrification of space heating equipment in existing housing persists at the same acceleration in the future, the residential stock could hit 25 percent total adoption rates—that is, including existing and new housing barring any new policy intervention—by 2039. Assuming a 50 percent reduction in space heating energy consumption in these homes owing to heat pump installation, this also translates to about a 7.4 percent reduction in total residential energy demand by 2038 from heat pump adoption alone—below the committee’s 30 percent reduction goal or the administration’s more varying 18–32 percent goal (Energy Saver 2022).5 Furthermore, assuming that all existing homes that transition to electric heat pumps were using carbon-based fuels for space heating beforehand, this would result in a 14.4 percent reduction in total demand for those fuels. In short, although the heat pump revolution is already happening, and adoption rates are increasingly meeting the committee’s original targets or the new national projections, it is not happening fast enough to meet the broader goals of reductions in carbon-based energy demands or overall energy demand.
A second consideration regarding the characteristics of households that are likely to replace current equipment with heat pumps and to live in the new homes in which heat pumps are installed during construction is also worth noting (Table 7-6). Currently, the rates of heat pump adoption do not vary significantly across either income or housing tenure groups—for example, 14.5 percent of households with annual incomes lower than $40,000 have heat pumps compared to 14.3 percent of households with incomes greater than $40,000 (EIA 2022b). Similarly, 14.8 percent of owner-occupied homes have heat pumps compared to 13.5 percent of renters’ homes. When factoring in the size of these groups, however, wide differences are seen in where heat pumps are found. Very low income households make up a much smaller
___________________
5 A reduction of 50 percent of space heating energy consumption (or about 20 mBTUs per home annually) in 25 percent of the existing housing stock (or 33.9 million homes by 2030) could result in approximately 678 tBTUs reduction in primary overall energy demand from residential buildings.
TABLE 7-6 Share of Households in Homes with Heat Pumps by Income and Tenure, 2020
Total U.S. | Less Than $5,000 | $5,000–$9,999 | $10,000–$19,999 | $20,000–$39,999 | $40,000–$59,999 | $60,000–$99,999 | $100,000–$149,999 | $150,000 or More | |
Income group | 14.4% | 12.7% | 14.0% | 14.2% | 15.1% | 15.7% | 14.6% | 13.3% | 13.0% |
Heat pump homes | 100.0% | 3.2% | 3.2% | 8.2% | 20.5% | 17.4% | 22.8% | 12.4% | 12.4% |
Total U.S. | All Owners | All Renters | SF Owners | SF Renters | All SF | MF Owners | MF Renters | All MF | |
Income group | 14.4% | 14.8% | 13.5% | 15.1% | 14.7% | 15% | 9.5% | 12.9% | 12.5% |
Heat pump homes | 100.0% | 69.1% | 30.9% | 66.8% | 10.6% | 77.4% | 2.3% | 20.3% | 22.6% |
NOTE: MF = multifamily; SF = single family. SOURCE: Tabulations of 2020 Residential Energy Consumption Surveys, courtesy of the U.S. Energy Information Administration, May 2022 (EIA 2022b).
share of heat pump home occupants than their moderate-income counterparts (although wealthier counterparts lag as well). Heat pumps are also more than three times more likely to be found in single-family homes than multifamily apartment buildings. Despite modest differences across household demographic groups, then, there is still sufficient disparity in adoption to warrant a policy intervention to close the gaps.
Equity Gaps: The Role of the Weatherization Assistance Program
Along this same vein, a review of the committee’s remaining quantified target regarding funding for WAP is also in order. However, identifying the gap between the committee’s recommendations is more complicated because eligibility is based on income qualifications (specifically, households earning less than 200 percent of the federal poverty level) rather than the energy consumption, efficiency or performance, or energy source of the home. Further complicating this calculation is the fact that energy benefits, including natural gas savings, from WAP have been estimated variably (Fowlie et al. 2018; Tonn et al. 2018).6
Barring more refined monitoring, outcome evaluation, and tracking of implementation across grantees, rudimentary calculations estimate the committee’s recommendations as minimally contributing to decarbonization goals.7 Assuming an accurate estimate lies between currently measured ranges, the increase in appropriations recommended by the committee would lead to about 105,000 homes being weatherized per year, for an additional nearly 0.95 million homes by 2030 since the first report. The committee’s desired program budgets could lead to total reductions of approximately 23.8 tBTUs in overall energy demand (or about a 0.3 percent reduction from the current total residential demand of 9.1 qBTUs) and a reduction of 19 tBTU in natural gas demand (the equivalent of 0.4 reduction).8 Even when added to the reductions estimated for the heat pump goals, then, WAP contributes very modestly to reductions in
___________________
6 Tonn et al. (2018) estimated a 29.3 mBTU/unit reduction in 2008 and a 26.6 mBTU/unit reduction in 2010; Fowlie et al. (2018) calculated annual energy savings of 17.2 mBTU/unit. For gas savings, Allcott and Greenstone (2012) calculate 20–25 percent natural gas savings, while Brown et al. (1994) estimated first-year savings of 17.3 mBTU.
7 The Department of Energy (DOE) estimates that 35,000 households are served per year based on an annual budget of approximately $300 million, or an average $8,571 per home (EERE 2022a). However, the most recent accounting of WAP grantees in 2019 found that the $1.12 billion in funding for the program year resulted in 85,200 weatherized homes, averaging $13,146 per home (NASCSP 2019). For the purposes of this exercise, the average of $10,858.50 is assumed.
8 The estimate assumes 950,000 weatherized homes with an average 25 mBTU total energy savings and a 20 mBTU natural gas savings per unit by 2030.
both overall and specifically carbon-based energy demand given its current statutory rules and implementation framework.
In addition to its energy ambitions, WAP is widely varied in its ability to meet its social impacts. Approximately 38.8 million households (about 30 percent of the United States) are currently at or below twice the U.S. poverty level and therefore eligible for WAP assistance—a need that would take centuries to serve even with the committee’s ambitious budget recommendation. Other indicators for identifying energy-specific needs may help better target decarbonization efforts. For example, recent data suggest a growth in overall energy insecurity. Considering just energy cost hardship, high energy burden (defined by Drehobl et al. [2020] and Fisher Sheehan & Colton [2021]9 as paying more than 6 percent of income for energy bills) remains a persistent national challenge for at least a quarter of the nation’s households, and two-thirds of WAP-eligible households face this burden (Drehobl et al. 2020). Median energy costs as a share of a household’s total housing costs are more than one-third lower for wealthier counterparts than WAP-eligible households, although lower-income households consume a third less energy per household and per household member.10
Other measures of household energy hardships such as housing inadequacy may provide a narrower target of opportunity for WAP than solely financial hardship. The physical energy performance of homes could be one obvious consideration. Such an overlay could better serve the 4.5 million households that experience uncomfortable cold or heat for extended periods owing to equipment breakdown, utility interruption, inadequate heating system capacity, or inadequate insulation among other reasons—568,000 of which experience severe housing-based heating inadequacy (data from AHS 2021; U.S. Census Bureau 2022). Alternatively, if the committee’s recommendation to include electrification as a WAP activity could be used to help further identify the most burdened households, then serving the 25.7 million WAP-eligible households that live in homes that rely on natural gas or heating oils may be a preferrable target.11 There are also 13.1 million households with incomes between $40,000–$60,000 living
___________________
9 This affordability percentage is based on the assumption that an affordable housing burden is less than 30 percent of income spent on energy, and no more than 20 percent of housing costs should be allocated to energy bills.
10 For households with incomes under 200 percent of poverty thresholds, median energy costs across all energy types are 32.8 percent of median housing costs compared to 22.3 percent of households with incomes above per 2021 AHS housing cost data. Per 2015 Residential Energy Consumption Survey (RECS) consumer expenditure data, households with incomes less than $40,000 consumed 63.4 mBTUs compared to the 92.2 mBTUs for those with higher incomes (EIA 2018).
11 Using the 25.7 million households in homes with carbon-based energy uses and the $13,146/unit average, the total budget needed is $337.9 billion, or $33.8 billion annually for the next decade.
in homes that rely on carbon-based fuels that are not WAP eligible but may not be able to afford electrification (AHS 2021).
Furthermore, the range of housing inadequacies that need to be addressed such that weatherization interventions are beneficial for decarbonization and energy reductions—as well as for the recipient households’ health and finances—are greater than the program currently has capacity to address (Kresowik and Reeg 2022). Given that the predominate beneficiaries of positive energy improvements continue to be wealthier households, a more nuanced eligibility metric that includes households’ energy burdens and their housing’s energy performance and decarbonization needs is needed (Frank and Nowak 2015; Hernández et al. 2014). Ultimately, targeted definitions of WAP eligible populations, services, and allowable expenditures per household would provide analysis to assess gaps more fully between need and committee recommendations.
Finding 7-3: The committee’s original recommendations for increased investments in WAP are meant to prioritize decarbonization efforts for households experiencing a range of energy hardships. However, defining the population and articulating outcomes (in both removal of carbon-based energy sources as well as improving energy efficiency to reduce overall energy burden) remains a work in progress, as does WAP’s capacity to meet the demand for eligible households.
Workforce Gaps
A major implementation challenge will be ensuring an adequate workforce to accomplish the necessary building, retrofitting, and construction. In 2022, the construction industry experienced its highest recorded level of job openings combined with an industry-low unemployment rate, and the Associated Builders and Contractors estimated that in 2023, the construction industry would need to attract an estimated 546,000 workers in addition to the normal pace of hiring to meet the demand for labor (ABC 2023). The federal and subnational actions described in the following section will further increase spending on construction. McKinsey modeling of the Bipartisan Infrastructure Law found that it could create 3.2 million new jobs across the nonresidential construction value chain, ~30 percent increase in the overall U.S. nonresidential construction workforce, or 300,000 to 600,000 new workers entering the sector every year (Hovnanian et al. 2022). However, these major legislative actions primarily include labor provisions that would grow the workforce (e.g., prevailing wage and apprenticeship programs) not as requirements, but as opportunities for “bonus” rates on tax incentives. The major legislation passed includes
workforce training as an option for several funding opportunities but does not directly invest in the education and training of tradespeople. (See Chapter 4 for a more detailed discussion of the labor provisions in recent legislative actions and on the challenge of attracting and retaining the necessary workforce to accomplish decarbonization.)
Summary of Gap Analysis
In sum, the committee’s targets for specific technological interventions (e.g., heat pump adoption) and program budgets (for WAP) lead to relatively modest levels of decarbonization. The short-term targets established in the first report are likely to be achieved independent of external intervention, although additional policies are needed to ensure that desired outcomes are met in future buildings and more tenuously for existing ones. Given the modest gap to meet the short-term targets, voluntary financial incentives would be reasonable to meet the committee’s original milestones. Yet, the sum of these individual targets is not likely to meet the committee’s more aggressive goals for energy reductions over the same time.
As precedent for the net-zero decarbonization visions for 2050, the targets are even more modest. For outcomes beyond the next decade, more concrete targets for the range of building interventions could be established in addition to clearer goals for both primary energy decarbonization and overall reductions in energy consumption. Like the committee’s first recommendations, these should be specified for new and existing buildings distinctly. Short-term targets such as heat pump adoption rates would need to expand to meet the magnitude of those goals in combination with other grid-level decarbonization and building-level direct interventions.
Significant intervention is needed to make up the gaps between current conditions, the committee’s more detailed intervention targets, and the committee’s broad goals for 2030 as stated in the first report. This holds true for all the quantified gaps in overall energy efficiency (particularly for the least efficient older building stock), heat pump diffusion, weatherization funding, and need. Yet, it holds even more true for what is needed beyond 2030. The interventions that could be employed for the first decade of the decarbonization transition must also set the stage for the decades after.
Finding 7-4: The committee’s original technological targets were modest and could be accomplished within a longer timeframe, although not one ending at 2030. Even new national goals established by the current administration, further, are more modest than the sector is capable of achieving. Meeting the overarching goals for reductions in the building sector’s energy demands and the removal of carbon-based fuels that are currently used directly in building operations requires
more aggressive action. Both policy incentives and regulatory intervention are needed to secure current trends and to move the sector toward the committee’s and the administration’s broader goals of comprehensive building-level electrification, overall energy demand reductions, and equitable transitions to both.
Policy Updates
Major policy adoption since the first report’s publication have set us on a few of these pathways. Indeed, federal, state, and municipal policy support for building decarbonization have increased during and since the publication of the committee’s first report. Each provides some achievement against the committee’s original 2030 energy reduction goals, although none as much as the federal investments in the past 2 years.12
National Policy Changes
In particular, the IIJA authorized $3.5 billion to WAP until expended (§40551)—which the White House estimates will aid 700,000 income-eligible households over the next decade based on past WAP mean expenditures per unit.13 However, a likely scenario given past expenditure rates is more than 322,000 homes weatherized, resulting in an additional 8 tBTUs reduced in total energy consumption (6.5 tBTUs in natural gas consumption). Assuming these funds get expended before 2030, this is only a fraction of $1.2 billion annual budget increases, or $10.8 billion by 2030, recommended by the committee in its first report (NASEM 2021).
Other IIJA authorizations that will shape the outcomes projected in the committee’s goals include the Energy Efficiency and Conservation Block Grant Program (§40552), State Energy Program (§40109), and Energy Efficiency Revolving Loan Fund Capitalization Grant Program (§40502), although their individual contributions are not readily measurable given the lack of evidence of reductions for the share of these
___________________
12 It should be noted that the IRA and the IIJA are not equivalent in funding mechanisms. The IRA primarily consists of spending programs (appropriations) and tax expenditures. Spending programs can allocate federal resources to projects and activities up to the amount of their appropriation. By contrast, tax expenditures, such as the production tax credits in the IRA, typically have no limit on the amount that could be claimed by taxpayers. The IIJA consists of a mix of authorizations and appropriations. Authorizations are laws that establish or continue a federal program or agency and are typically passed by Congress for a set period of time, but authorizations require appropriations before funds can be spent. Appropriations are laws that actually provide the money for government programs and must be passed by Congress every year in order for the government to continue to operate.
13 DOE assumes an average $4,695/unit (EERE 2022b).
programs that already exist and the implementation questions for the new programs. The IIJA authorizations comes with no extensive statutory changes to the program beyond the removal of Davis-Bacon requirements for the weatherization of buildings of fewer than five units, which make up the bulk of interventions. This change will likely increase the number of those building types given the added program resources that can be used for their weatherization, although at the expense of prevailing wage benefits. This segment of the residential stock is also the least efficient.14 Consequently, this targeting could result in greater efficiency gains than estimated previously (e.g., closer to 25 percent natural gas reductions).
The IRA, however, is the most relevant federal policy change for decarbonizing the built environment due its combination of tax credits and direct rebates for a wide range of technologies and energy-performance actions in new and existing stocks of both residential and commercial buildings (ACEEE 2022; Azerbegi 2022; DOE Office of Policy 2022; Evans 2023; Guidehouse Insights 2022; Philadelphia Energy Authority 2023; Smedick et al. 2022). Estimates of the act’s possible building outputs for electrification and energy efficiency range widely.
Taking heat pump adoption projections alone, the IRA is projected in one scenario to directly install 7.2 million in retrofits via the 25C tax credit.15 Additional opportunities that are likely to add to heat pump adoption counts in the existing housing stock include the low-income rebate and home energy retrofits programs, respectively projected to touch 2.4 million and 1.2 million households (Smedick et al. 2022). Presuming that one-half of these later programs’ recipients opt to utilize the incentives for heat pump installation, 1.8 million more heat pumps will be added. Current projections for new homes benefiting from the 45L tax credits can also assume to add 650,000 heat pumps at a minimum to the housing stock (Smedick et al. 2022), bringing the total to 10 million existing homes with new heat pump installations by 2032.
With these new tax credits and direct rebates from the IRA, the committee estimates increased heat pump adoption rates starting in 2023, which would accomplish the committee heat pump adoption goals by 2029, 10 years before the currently projected industrial diffusion. By the IRA benefits’ closeout in 2033, furthermore, the residential heat pump adoption will have surpassed the committee’s goals by an additional 2.3 percent and 27.3 percent of the entire housing stock (or 38 million homes) will have electric heat pumps. The IRA’s heat pump incentives alone would
___________________
14 According to WAP American Recovery and Reinvestment Act evaluations and RECS consumption data for 2015, 2–4 unit residential buildings consumed 52.5 kBTUs/SF compared to 37.1 kBTUs for single-family attached and 38.8 kBTUs for buildings of more than five units. See also Martín et al. (2023).
15 Among the most extensive estimates are those produced by RMI (Smedick et al. 2022).
reduce overall residential energy consumption by 8.3 percent and carbon-based fuels specifically by 15.9 percent by 2033—or one-third of the committee’s 2030 goal.16
The range of other electrification and energy-efficiency improvements incented by the IRA would produce additional modest reductions. For example, based on analysis of realized project savings in annual consumption averages at current rates, the approximately 1.2 million households who could benefit from Home Owner Managing Energy Savings (HOMES) rebate programs17 would produce 32.4 tBTUs aggregate reduction in overall energy consumption (or about 0.7 percent), assuming all households attempt the aggressive 35 percent reduction rebate threshold. If 115 million square feet of commercial building space are retrofitted at 25 percent efficiency improvement owing to the IRA’s extended 179C tax credit, 2 tBTUs are reduced.
Appropriations for federal buildings—another opportunity targeted by the committee—could result in additional gains.18 Other IRA provisions, such as local government incentives to adopt and enforce more rigorous building energy codes, would lead to additional reductions for new construction (Tyler et al. 2021). Of important note is the IRA’s Greenhouse Gas Reduction Fund (§60103), the $27 billion set of competitive grants for “green banks” that could finance distributed community energy projects as well as household efficiency and electrification programs.
Finding 7-5: The new federal policy terrain helps to fill the gaps or, more accurately, expedite the achievement of several of the committee’s original targets. IIJA funding for WAP will provide an opportunity to target eligible households and energy-reducing improvements, including electrification, more effectively. IRA tax credits and rebates are also significant: IRA incentives for heat pump installations will meet the committee’s targets by 2029 (a decade ahead of business-as-usual adoption rates). These installations alone could help reduce energy in 38 million homes by 8.3 percent of current consumption (15.9 percent of carbon-based energy consumption). Combined with other building improvements funded, recent federal laws could move the country significantly toward the committee’s 2030 goals and the sector’s expected national greenhouse gas reductions.
___________________
16 See Table 7-5 for more on the committee’s estimates for heat pump diffusion, and NASEM (2021a) for more on the committee’s 2030 goals.
17 HOMES rebate programs are implemented by state energy offices, providing rebates to cover a percentage of costs for retrofit projects that achieve 15 to 35 percent energy system savings. For more details on the $4.3 billion appropriated in the IRA to DOE to distribute as grants to these programs, see P.L. 117-169, title V, §50121, August 16, 2022, 136 Stat. 2033.
18 The administration has moved forward with actions related to the federal stock as well (White House 2022a).
There are several caveats to these projections. This analysis assumes that eligible property owners would likely choose the technologies offering the largest credit or rebate (e.g., heat pumps with a $8,000 rebate for the lowest-income eligible households). This may not be additive—that is, many of the households and homebuilders that take the tax credits or rebates may have been purchasing heat pumps anyway. Regardless of motivation, the IRA incentives certainly help secure those purchases and produce the same decarbonization results.
A second concern is ensuring that the lower-income households that are eligible for larger rebates can actually access the incentives, which require them to own property and assume they have sufficient information and resources to initiate a retrofit (or that they are renters in multifamily buildings with willing property owners). Households that are WAP-eligible but have not benefited from the program—as well as the larger pool of low–moderate income households that are not WAP-eligible but eligible for the IRA rebates—will require extensive outreach and engagement programs for which most current programs have not had the capacity to experiment successfully (Cluett et al. 2016). Harnessing the marketing potential of product vendors, retailers, and service providers such as building contractors and remodelers will be critical for the IRA’s building decarbonization ambitions among this population.
Consequently, the third and most critical concern about achieving the IIJA’s and the IRA’s projected outcomes rests in their implementation across various building sector programs. Fundamental challenges in relation to the kinds of information that property owners and occupants receive regarding the various incentives, the potential confusion between incentive benefit duplication, and their eligibility will need to be established via federal program rules (particularly the Departments of the Treasury and Energy) as well as state rebate programs and their expected plans. The burdens associated with proving eligibility and compliance could inhibit take-up as well. The availability of local technical assistance to help households make complicated financial decisions and the gaps in assistance providers’ capacity for both WAP agencies and state and local government energy offices could undermine implementation over the next decade.
Finding 7-6: Recent national laws such as the IIJA and IRA may not have sufficiently streamlined implementation plans and resulting execution to meet those targets. Capacity gaps at all levels of government and service providers may further complicate these policies’ achievements—and will define perceptions of further public appropriation and programming for efforts after the next decade and through the 2050 goals.
Subnational Policy Changes
A significant reason for the increasing take-up of heat pumps prior to the IRA has been the combined mandates and incentives established by state, tribal, and local governments. Most of these laws and programs have focused on the reduction or near-elimination of carbon-based fuels in new construction—that is, electrification—though energy efficiency improvements by regulation as largely represented by state adoptions of model energy codes for new buildings have been the much longer policy vehicle (Berg 2022; Berg et al. 2020). A few states have also incented or mandated distributed renewable energy production.19 For example, California’s goal of achieving zero net energy for new residential buildings by 2020 and by 2030 for commercial buildings has resulted in codes with prescriptive requirements for heat pumps (CPUC n.d.). Other states, including Massachusetts and New York, have also adopted multiple intervention points to meet net-zero building energy legislation, including requiring all-electric new home construction.20
The focus on new construction provides the avoidance of inefficient energy consumption; increased energy codes have resulted in an estimated 45 percent reduction in new home energy consumption and almost 55 percent reduction in new commercial buildings since the first codes were introduced a half-century ago (PNNL and DOE 2022). A mandated gradual increase in the number of new homes with heat pumps during the IRA timeframe leading to a complete requirement by 2033 would yield an additional 180 tBTUs in energy reductions (more than 10 percent of total residential consumption when combined with the IRA incentive reductions).
Several states are also leading the “net-zero” charge. For example, Massachusetts established a Stretch Building Energy Code (i.e., beyond the national model energy code) focused on carbon performance rather than prescriptive construction specifications (State of Massachusetts 2022). The challenge with focusing exclusively on new construction is the extensive increase in construction costs, which, in turn, yield unaffordable housing overall and the transfer of lower-income households to the more inefficient existing stock.
Furthermore, the overwhelming share of building sector energy use comes from existing stock, not new buildings. Yet only a handful of subnational policy
___________________
19 For example, in 2019 and 2022, respectively, California passed new ordinances requiring all new homes to have a solar photovoltaic system and all new commercial buildings to have a solar photovoltaic array and an energy storage system.
20 For examples of mandates, see New York State Climate Action Council Scoping Plan (New York State Climate Action Council 2022). Incentive programs include California’s Building Initiative for Low-Emissions Development Program (California Energy Commission 2023).
interventions have focused on the existing building stock, and they have typically focused on larger commercial and multifamily buildings (BDC 2023; Sobin 2021). For example, building “benchmarking,” or measurement and public reporting of large buildings’ energy use, has become the norm in most larger cities over the past decade (IMT 2022).21 The specific requirements of these laws vary, but typically involve annual reporting of energy consumption and GHG emissions, energy audits, and retrofits. Several of these cities have since used benchmarking of larger properties to set building performance standards to match the cities’ long-term GHG reduction goals or, more prescriptively, to conduct mandatory audits with retrofit or retro-commissioning reporting as well (Hart et al. 2022). New York City’s Local Law 97 is among the most aggressive of these mandates (NYC 2022). However, small cities such as Ithaca, New York, and Menlo Park, California, have committed to the carbon neutrality of their entire building stocks by 2030 (Harding 2021; Woody 2022). While some stakeholders have raised concerns about the costs and burdens of monitoring and evaluation, building data disclosure laws are increasingly recognized as a useful tool for driving energy efficiency and reducing emissions in the built environment (ACEEE 2014; C40 Cities Climate Leadership Group and C40 Knowledge Hub 2019; Palmer and Walls 2015, 2017; Shang et al. 2019).
For existing buildings, state mandates can focus on replacement of energy-consuming equipment (Berg et al. 2022). Although federal law typically leads equipment standards, several states have moved forward with their own increased efficiency requirements and, in some cases, electrification requirements. For example, California recently prohibited natural gas heating equipment beginning in 2030. California has also set goals for the installation of heat pumps and set aside funds for this effort. Other jurisdictions are following suit, although often relying on direct funding or financial incentives to encourage property owners to convert in addition to strict mandates for the equipment (Cohn and Esram 2022). More evidence is needed to determine the effect of these subnational interventions on the building energy consumption within these jurisdictions and to the overall reductions for the nation.
Finding 7-7: State, tribal, and local governments play a major role in decarbonizing the nation’s building stock, particularly for new construction through mandated codes. However, returns on these policies in overall energy reductions and the removal of direct carbon-based fuel are diminishing. There are signs that
___________________
21 Single-family housing, however, does employ common energy labels, although these are typically used only for new construction mandates (NASEO 2022).
governments are turning their attention to retrofits for electrification and efficiency in their existing buildings, although there are only a few cases to date. Most of these, further, are awaiting enaction, so there are few clear implementation plans from which to learn and report upon.
In short, recent adoption of local, state, and national policies are projected to move the nation slowly toward decarbonization goals for 2050. These recently enacted policies are a work in progress and, in cases like the IRA, require significant implementation design and action—not just for the public-sector officials charged with designing and launching programs but also for the building industry and owners (including individual households in the latter) to act, particularly when the policy supports are voluntary. Implementation will involve private industry actors and individual building owners and households—the same stakeholder groups that built and maintain the current built environment. A deep understanding of these stakeholders and their role in manifesting the built environment is needed.
Consequently, after considering all the findings presented in this chapter, the committee is most focused on the implementation challenges of this first decade after IIJA and IRA passage into law and during which the more aggressive state and local policies will have taken shape. A particular concern is the integration of equity and burden during implementation.
Recommendation 7-1: Ensure Clarity and Consistency for the Implementation of Building Decarbonization Policies. The Department of Energy should develop rules for the implementation of energy programs (particularly those funded through the Inflation Reduction Act [IRA]) that increase access for households and other property owners of various incomes while decreasing their burden for participation. Such rules include simplifying the definitions of points-of-sale and income verification protocols for rebates, which might benefit from universal screening for all federal assistance programs. These terms should align with other federal agencies’ definitions for eligibility of services and should also serve to set up an evaluation of building program outcomes (such as across state IRA plans and outputs).
Recommendation 7-2: Promote an Equitable Focus Across Building Decarbonization Policies. The Department of Energy (DOE) should establish requirements for states to market and provide appropriate levels of service to the owners and occupants of rental, multifamily, and low-to-moderate-income occupied buildings as a prerequisite for approval for state energy rebate plans. These plans should include educating households and
commercial building occupants about all the incentives and resources available to them, as the rebate plans will be a gateway for additional education needed to support further decarbonization after 2033. Ensuring that all states’ processes for creating awareness of contemporary financial incentives are consistent, fair, and include authentic local community engagement will ensure that a wider population can access them. DOE’s frequent and comprehensive monitoring of the execution of these processes after approvals of state plans will also allow department staff to assign additional technical assistance and related guidance to states to revise and improve their implementation.
Recommendation 7-3: Expand and Evaluate the Weatherization Assistance Program (WAP). As the predominant national vehicle for energy improvements among low-income households, WAP should be enhanced in its purpose, scope, and activity in addition to the appropriations increases the committee supported in its first report. Given questions about the program’s capacity, the Department of Energy should fund an independent set of evaluations of WAP with an eye toward reform that provides a range of decarbonization interventions discussed in this report that are appropriate to each housing unit. An eligibility analysis should derive alternative population targets that ensure that WAP covers any household not covered by other programs such as rebates and tax credits. An engineering study should determine an appropriate increase in the assistance per household. An implementation study and monitoring program should ensure that decarbonization targets are met, and an outcome study should assess strategies that ensure positive household financial and health outcomes.
Recommendation 7-4: Coordinate Subnational Government Agencies to Align Decarbonization Policies and Implementation. States should focus on utilizing internal governmental expertise to de-silo the knowledge and training required to expand all building-level energy interventions’ implementation within their state government offices and, in turn, county, tribal, municipal, and other subnational government offices. For example, state energy offices should coordinate with community services, housing, and community development agencies to better target weatherization assistance funds as well as state rebate programs. Furthermore, local building inspection and housing departments should be utilized to better support building improvements in new and existing residential and commercial buildings.
Recommendation 7-5: Build Capacity for States and Municipalities to Adopt and Enforce Increased Regulatory Rigor for Buildings and Equipment. Congress should require and provide resources for the Department of Energy’s continued upgrading of building appliance standards (including phasing in electrification of appliances, as applicable). Congress should also require the adoption of increasingly rigorous energy building codes by state and local governments in conjunction with state and municipal block grant programs (also including mandatory electrification) over the next decade. State and local governments should also require that (a) local governments resource, staff, and enhance building departments’ capacity to enforce codes, as well as assist in state and local building benchmarking and audit services for existing buildings; and (b) subnational governments consider adopting mandatory appliance and equipment electrification in new buildings by 2035, after which national standards could increasingly require electric-only alternatives. Relevant regulatory initiatives such as the Infrastructure Investment and Jobs Act’s Cost-Effective Codes Implementation for Efficiency and Resilience Program ($225 million, §40511) and the Inflation Reduction Act’s Technical Assistance for Latest and Zero Building Energy Code Adoption Program ($1 billion, §50131) should dedicate a portion of their available funds to monitoring the effectiveness of this technical assistance as well as tracking its outcomes in energy savings or electrification rates.
BROADER DECARBONIZATION STRATEGIES FOR THE BUILT ENVIRONMENT
The opportunities for decarbonization in the built environment rest on more than just addressing buildings’ energy consumption. Taking into account the life cycle of energy systems, almost twice as much energy is produced for buildings than is used by them—making the building sector alone one of the biggest end use sectors for carbon-based fuels. Electricity used within buildings account for 74 percent of total retail electricity sales and the emissions related to producing and delivering that power—well beyond the “Scope 1” emissions associated solely with buildings’ direct use of energy. Improving the efficiency and managing building energy use through tools like digitalization and demand management, distributed energy generation, and energy storage are as critical to decarbonizing the built environment as are the actions to clean up the electricity grid that supplies buildings with power.
But the GHG implications of the built environment go beyond just the buildings themselves. Where physical infrastructure is located and how it is managed may contribute negatively to local environmental impacts such as air quality and the
health and equity outcomes of neighboring communities, and affect such things as land values, congestion, and other community outcomes. Energy infrastructure shapes the built environment just as the built environment affects demands for energy. These patterns are shaped not only by natural phenomena (such as topography and waterways) but also by societal structures, like land use policies and building regulations—the governing frameworks of the built environment. These policies and regulations vary across and within states. Although complicated and challenging to accomplish, changes in such frameworks may provide opportunities for reducing GHG emissions from the overall built environment.
Finding 7-8: Given the various patterns of development in cities, towns, and rural communities, there is no one size fits all for decarbonization strategies across buildings and communities and regions. Decarbonization of the built environment requires tailored, place-based approaches.
Like increasing building requirements, federal incentives for subnational governments to implement community-level decarbonization strategies should involve appropriate implementation transition periods. Because the process of adopting and implementing community and land use changes in the built environment takes longer than retrofitting of individual buildings, it would be helpful to use modest incentives across a wider range of federal programs for subnational governments (including transportation, water, broadband, housing, and energy block-grant resources) to encourage community decarbonization efforts as early as reasonably possible for outcomes to show up as early as 2033.
Given the range of potential decarbonization interventions in the built environment and the likely diversity of their combinations that might be applied in settings across the nation, issues might arise related to ownership, regulation, and governance structures of different local infrastructure (including such things as electric delivery systems, district heating, microgrids), where current structures stand in the way of efficient and effective development and use of distributed energy resources (DERs). Better coordination among states’ energy offices, public utilities commissions, and housing and community-development agencies could support the provision of broader access for all households and communities to such technology and infrastructure options. Further experimentation, technical resources, funding, and financing resources are needed for community distributed renewable projects. Financing alternatives may include revolving loan funds and green banks, to be paid back from production revenues and household energy savings. Financing from federal seed monies from the new IRA Greenhouse Gas Reduction Fund (§60103) could support such outcomes. Civic and public assistance for lower-income communities should also support sustained maintenance and operation.
There are important research questions for the future that relate to approaches affecting the broader built environment, their potential to reduce GHG emissions in the long term, and the matching of alternative decarbonization strategies with different local conditions. These questions go beyond building science, engineering, and construction research—all of which have been underfunded by the national research agencies.22 Funding for research on community-level decarbonization interventions in the built environment is generally even more limited and less realized than those for individual buildings. DOE, the National Science Foundation, the Department of Housing and Urban Development (HUD), and the General Services Administration (GSA) all have a role to play in identifying important social science, engineering, economic, and legal research and policy analysis questions.
Building Policies and Actions for Rapid Decarbonization in the United States
Policies, incentives, and investments in new and existing buildings and community systems can improve individuals’ quality of life while offering the deep carbon savings needed to reach carbon neutral targets. Technology already exists to pursue greater emissions reductions than current targets specify (DOS and EOP 2021), which would take pressure off other sectors. For example, increased effort in the building sector would reduce the need for the risky reliance in the administration’s plan on large deployments of technologies that are not yet ready, such as direct air capture (see Figure 1-3). A more aggressive target for increased energy efficiency in buildings and the built environment would reduce demand for heating, cooling, and transport electricity. This would reduce perhaps the greatest execution risk the nation faces in decarbonization, by allowing a less daunting pace of deployment for new transmission and renewables infrastructure (see Chapter 6). A number of published emissions scenarios include much more aggressive decreases in emissions from buildings and the built environment (IPCC 2023). The Biden administration explains the targets for the built environment in their Long-Term Strategy by pointing to the longevity of existing buildings and the high average cost and disruption of retrofits (DOS and EOP 2021). This is one sector in which the policy instruments available at subnational scales may provide stronger targets and incentives than those available to the federal government. In large part, this is because building codes and standards in the United States are set at state and local scales.
The following sections take a fresh look at what design, engineering, and innovation can do to reduce emissions from the building environment and conclude that the
___________________
22 Housing-related technological research was estimated at 0.6 percent of all federal non-defense RD&D (Hassel et al. 2001).
federal administration’s goals could be significantly tightened through a variety of national and subnational actions. It is in the nation’s interest to significantly accelerate reductions in energy demand and associated GHG emissions from the built environment by implementing these actions wherever practical and politically possible to do so. In addition, subnational policies in this sector offer an unusually large opportunity to reduce decarbonization risks by broadening the nation’s climate and energy policy portfolio, by adding standards to a portfolio dominated by tax incentives.
The following prioritized actions build on the strategies laid out in NASEM (2021b) and outline what could be achieved through a more aggressive set of policies emphasizing increased efficiency and reduced demand for energy in the built environment, alongside decarbonization opportunities that emerge when building technologies are more tightly coupled to the energy system and the grid. The built environment has the technical potential to reduce emissions by 900 MMtCO2/year by 2050 (Ungar and Nadel 2019), supporting a net-zero future in the built environment with the expansion of renewables, while improving quality of life. In addition to strategically implementing the IIJA and IRA, and extending commitments beyond the next 10 years, the United States needs to increase its portfolio of decarbonization innovations to fully engage the built environment, in keeping with the leading nations around the world.
1. Accelerate Appliance and Equipment Efficiency. 5.6 quads of reduced annual energy demand, 210 MMtCO2 of reduced annual emissions.
In 2019, the American Council for an Energy-Efficient Economy (ACEEE) identified 5.6 quads per year of energy efficiency that could be achieved through specific appliance and equipment upgrades, leading to 210 MMtCO2/y of reductions or an equivalent of 1.64 trillion kWh (Ungar and Nadel 2019, p. 54). Of these savings, 70 percent come from a dozen products that could be accelerated to achieve Energy Star performance in the top 25 percent of their market, including residential water heaters, heat pumps/central air conditioners, boilers and furnaces, refrigerators, as well as commercial/industrial fans, electric motors, transformers, air compressors, and packaged unitary air conditioners and heat pumps. An NREL study found that efficiency improvements in the range of 0.5 to 2 percent per year for electric building technologies could completely offset the electricity load growth associated with building electrification for decarbonization (Steinberg et al. 2017). The Biden administration estimates that increased stringency of appliance and equipment standards have the potential to reduce emissions by 2.4 billion metric tons by 2050
(White House 2022b). With the expansion of the recommendations from the committee’s first report, the further acceleration of appliance efficiency goals for the following appliances will be critical for decarbonization:
- Heating (efficiency with electrification)
- Domestic hot water (DHW) (efficiency with electrification)
- Lighting (even more efficiency possible)
- Refrigerator-freezers (more efficiency possible)
- Air conditioning (with more innovation and decarbonized refrigerants)
- Cooking and clothes drying (efficiency with electrification)
- Miscellaneous electric loads now 30 percent of building electric demand
The committee’s first report and Recommendations 7-5 and 10-2 of this report include energy efficiency and emissions manufacturing standards for appliances as a backstop to the incentives offered in the IIJA and IRA. These standards would include rigorous support for Energy Star certifications and national (or, as historically, state by state) requirements that codify the top 20 percent of performers (receiving Energy Star designation; ASAP 2023) to become the mandatory minimum within 5–10 years, with continuously updated minimums to reflect advances in appliance efficiency.
The most rapid acceleration for appliance and equipment upgrades may occur in response to mandates for building electrification, especially in the installation of heat pumps for heating and hot water. For building electrification to contribute to significant carbon savings, however, renewable electricity sources and demand efficiency measures would need to be fully in place. In addition, heat pump electrification priorities would be needed to ensure carbon benefits and to protect occupants from higher energy bills. Priorities for heat pump installation for these outcomes may necessitate a critical path (Deetjen et al. 2021; DOE 2016; Pantano 2020; Waite and Modi 2020) that includes air conditioner upgrades to heat pumps for heating and cooling; oil heating system upgrades; electric resistance heating system upgrades; gas heating system upgrades in mild climates; and, only then, gas heating system upgrades in cold climates with hybrid fuel capability for extremely cold days—each of these with cooling as needed and potentially integrated hot water. DOE’s Residential Cold Climate Heat Pump Challenge is intended for rapidly improving cold climate heat pumps so that hybrid heat may not be needed in a decade, and reducing the installed cost of geothermal heat pumps may be even more impactful for reducing the large carbon footprint of heating and cooling in the United States (DOE n.d.).
2. Mandate and/or Incentivize Zero-Energy New Homes and Commercial Buildings. 5.7 quads of reduced annual energy demand, 265 MMtCO2 of reduced annual emissions.
The design, engineering, and construction professions have been delivering net-zero new buildings wherever clients or codes mandate, by combining very low energy use per square foot requirements with on-site or purchased renewable energy sources. However, the vast majority of new buildings today are not net zero. The Architecture 2030 Challenge calls for all new construction to be net zero by 2030 and for all major retrofits to achieve a 50 percent emissions reduction by 2030 (Architecture 2030 2023). The 2030 Challenge goals have been adopted by 1,200 architecture firms, 15 cities, and the U.S. Conference of Mayors. If zero-energy goals for new homes and commercial buildings were to be achieved nationally, then the United States would save more than 5.7 quads of energy and 265 MMtCO2/y by 2050 (Ungar and Nadel 2019).
To achieve net-zero energy use intensity in all new residential and commercial buildings by 2030, a full suite of subnational actions would need to be advanced state by state: (1) ASHRAE, Zero Code, and IECC2021 code adoption; (2) tax credits; (3) qualified allocations for passive house construction (especially for low-income housing); and (4) distributed renewable targets and incentives, and stretch code mandates that reduce site Energy Use Intensities (EUIs) below 30 kBtu/ft2/year (e.g., State of Massachusetts 2022). Together, these actions would reduce energy use on site by 70 percent in new residential and commercial buildings by 2030, with the remaining 30 percent met by on-site or purchased renewable sources to achieve net zero (NBI 2019; USGBC MA 2019). Figure 7-2 displays one modeling exercise showing the impact these actions could have on the energy use intensity of new construction compared to the current commercial building stock.
The design expertise, technologies, and standards for net-zero new construction have been demonstrated but not enacted beyond a few leading states (e.g., California, New York, Massachusetts, and Colorado). These states have demonstrated that the incremental financial cost of purchasing and operating a new net-zero building offers some of the most cost competitive carbon savings, with 0–10 percent increased first costs even for low-income projects, offset by operational cost savings (Leach et al. 2014; NBI 2012). The Zero Energy Buildings targets for low-income housing in New York City and Massachusetts address inequities in the built environment, including energy security and resiliency for all citizens during power outages (Cleveland et al. 2019; NASEM 2021b; NBI 2019). The IIJA includes modest related funding for housing, including $225 million for DOE’s Building Technologies Office to offer state grants for advancing sustained, cost-effective implementation of updated building energy codes (fiscal years 2022–2026) (§40522).
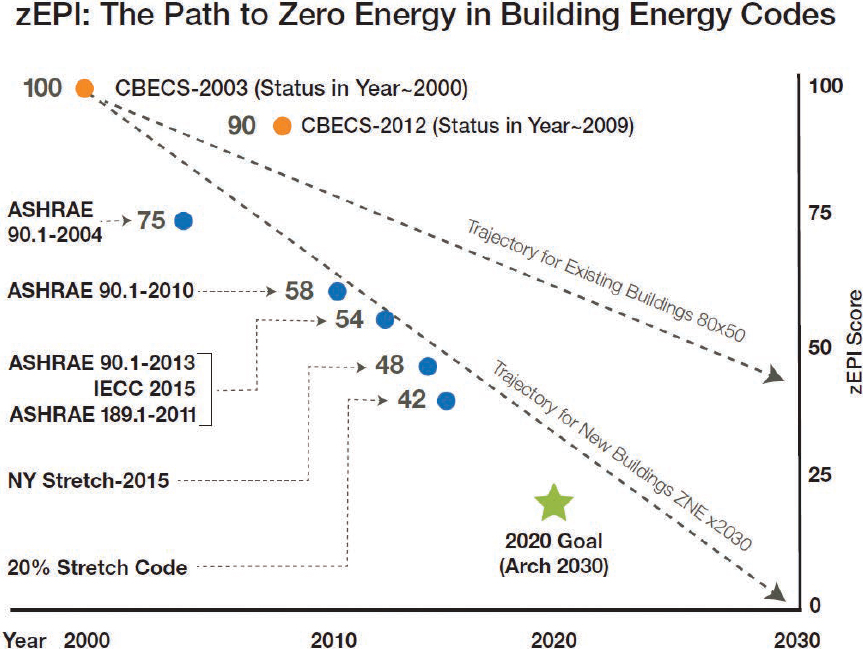
3. Incentivize Retrofits for Existing Homes and Commercial Buildings. 3.8 quads of reduced annual energy demand, 148 MMtCO2 of reduced annual emissions (not including the reductions in plug loads).
Even by 2050, existing buildings will still dominate the residential and commercial portfolio. The largest energy demands in residential and commercial buildings are for heating, cooling, ventilation, and lighting—each of which can be measurably reduced through thermal and air tightness improvements in building roofs, walls, windows, and foundations, which directly impact the sizing and performance of mechanical and electrical equipment. Retrofitting the nation’s existing buildings to improve their energy efficiency would require significant expansion of relevant manufacturing and training, and would need to address the barriers and disruptions that accompany most retrofits, as well as the up-front capital cost, even when reduced operating
costs would more than compensate over time. Many households lack access to financing or even ownership, especially in underserved communities (Kirk 2021; see Chapter 11). On the other hand, the technologies are proven, unlike the atmospheric carbon removal technologies that the nation will need if it cannot do more to reduce emissions from buildings and industry (Chapter 10). To advance a national plan for retrofitting the nation’s current portfolio of buildings, the first step would be the energy use benchmarking, followed by annual reporting of progress. The Environmental Protection Agency’s (EPA’s) Portfolio Manager is the nation’s repository for energy benchmarking and needs to be strengthened to ensure that the data are robust, transparent, and analyzed with the most advanced expertise. Benchmarking would be followed with aggressive goals and funding for prioritized investments. In 2022, President Biden launched the National Building Performance Standards Coalition, a nationwide group of approximately 40 state and local governments that have committed to inclusively design and implement existing building performance policies and programs in their jurisdictions, with shared goals and solutions (National BPS Coalition n.d.). Leaders including New York City, Boston, Denver, and others have matched benchmarking and aggressive goals with prioritized investments in existing buildings based on the detailed understanding of where the energy is lost through end use load breakdowns (Figure 7-3; USGBC MA 2019).
A national program to retrofit existing buildings would perhaps do more to advance environmental justice during the energy transition than any other action, with the possible exception of reduced air pollution exposure from conventional fossil pollutants (Chapter 2). The IIJA and IRA provide a significant start by a commitment to retrofitting 1.3–2.5 million buildings inhabited by low-income households with heat pumps. These residential expenditures will address only a portion of the urgent need for investments in the energy efficiency of both the residential and commercial buildings that house, educate, employ, and service the poorest U.S. residents.
Not only have disadvantaged communities been locked out of home ownership, they are also often fated to live, study, and work in substandard buildings with the highest energy costs, relying on subsidy or sacrifice to pay the bills. Low-income households bear an energy burden that is 3 times greater than that of non-low-income households, with the national average standing at 8.6 percent (DOE 2020a), and even higher for households in the lowest decile of income. While these costs may be classified as an energy burden (unaffordable), they often are accompanied by energy insecurity (reliability and outage risk) as well as full energy poverty (no electricity or gas) with serious risk to health and life (Biswas et al. 2022; see Chapter 2). The federal program known as LIHEAP—the Low Income Home Energy Assistance Program—has been the primary federal instrument aimed at reducing energy burdens and energy
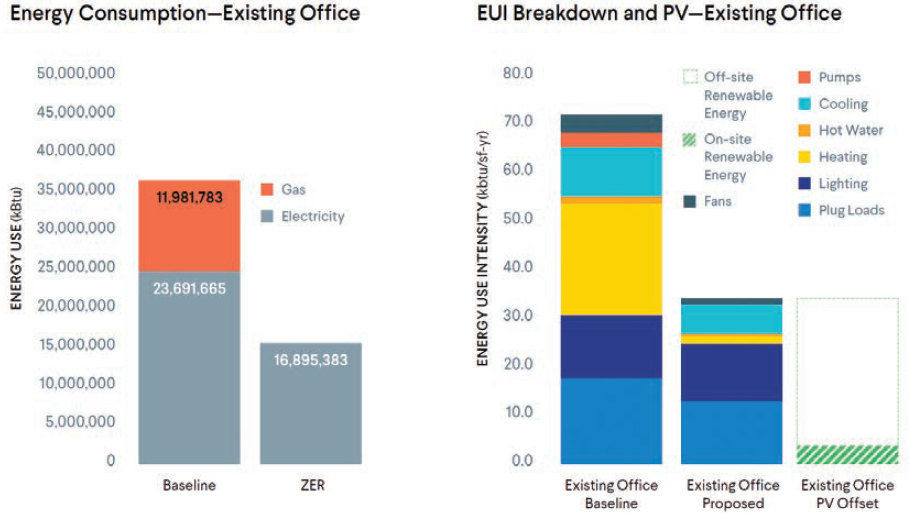
SOURCE: Courtesy of BE+, USGBC MA (2019).
poverty (HHS 2023). These annual subsidies keep the lights and heat on for many low-income households, but do not address the underlying challenge of excessive energy demands of inadequately built and maintained buildings. Thirty percent of U.S. households (38.6 million homes) meet the income requirement for WAP assistance (Drehobl 2020). A national effort to retrofit all existing housing stock would necessarily make the greatest difference in the homes that need upgrades the most and would thus provide the largest benefits to disadvantaged communities. Also, a comprehensive retrofitting effort would cover all eligible households, not just owner-occupied homes (Kirk 2021). Earlier studies estimated the impact could be at least 35 percent residential energy savings and 20 new jobs per million invested (ACEEE 2011; DOE 2011).
The potential of existing building retrofits, both residential and commercial, across all socioeconomic spectrums, can be more than 50 percent savings of the present energy demand in buildings (RMI n.d.). The IRA’s $4.3 billion for grants from DOE to state energy offices to develop and implement a whole-house energy savings rebate program (§50121) and $0.2 billion for states to establish training and education programs for contractors who install home energy efficiency and electrification improvements (§50123) is a critical start and could be expanded to address existing commercial buildings as well.
4. Accelerate the RD&D for Smart Technologies and Systems for Homes and Commercial Buildings. 3.2 quads of reduced annual energy demand, 125 MMtCO2 of reduced annual emissions.
In addition to increased use of proven technologies, an effort to significantly tighten and accelerate national targets for building decarbonization (i.e., Figure 1-3) would benefit from additional investments in RD&D of innovations in the built environment. Four major areas of innovations for buildings are described here to illustrate critical needs: engaging the Internet of Things (IoT) for building energy use controls; developing hydrofluorocarbon (HFC) alternatives; innovating to reduce embodied carbon; and advancing GEBs.
The IoT has created the potential for a transformational change in society but has experienced a surprisingly slow pace of integration into buildings. With each piece of mechanical equipment and every appliance, light fixture, and window control having an IP address, the ability to monitor and control energy consumption while ensuring a high level of occupant service provides unprecedented opportunities for increasing the energy efficiency of buildings. At the residential building level, smart thermostats had been installed in more than 19 million homes at the end of 2021, reducing national energy consumption for space heating and cooling by 1.4 percent and saving residents an average of 8 percent on their heating and cooling bills (Barbour 2021; EnergyStar n.d.; Walton 2022). At the commercial building level, BAS can monitor and control heating, ventilation, cooling, lighting, elevators, and multiple energy-intensive devices, reducing commercial building energy consumption by 10 to 30 percent (EIA 2022a; Fernandez et al. 2017). Sixty percent of large commercial buildings (>50,000 square feet) in the United States have a BAS to control heating, ventilating, and air conditioning; lighting; and more. But only 13 percent of small- to medium-size (<50,000 square feet) buildings have adopted the technology, leaving more than 75 percent of all commercial buildings in the United States primed for opportunity (Trenbath et al. 2022).
The challenge is learning from the growing repository of monitored sensor and controller settings to provide a high level of occupant service with low energy and carbon demand. Given the volume of data and the complexity of optimal control for the significant variations in building types and climates, the addition of an IoT with smart controls for carbon savings in buildings is an ideal challenge for new private and public investment in artificial intelligence and machine learning to be rapidly developed with the building sector.
HFC Alternatives for All Heat Pumps, Refrigerators, and Air Conditioners
Air conditioning is a triple threat to climate—from its operational energy to its impact on peak demand, to its use of refrigerants. The HFC refrigerants in heat pumps, refrigerators, and air conditioners are major sources of fugitive HFC emissions. HFCs have a 100-year global warming potential 3790 times larger than CO2, and the use of air conditioning is growing at a rate of 10–15 percent per year worldwide. HFCs also persist in the atmosphere for an average of 29 years (Climate and Clean Air Coalition 2020). With the Kigali Amendment to the Montreal Protocol, nearly 200 countries have committed to reduce the production and consumption of HFCs by more than 80 percent over the next 30 years to avoid more than 70 billion metric tons of CO2 equivalent emissions by 2050—and to reduce up to 0.5°C of warming by the end of the century (UN 2023). The Senate ratified and President Biden signed this amendment in 2022 (Department of State 2022). The IRA includes a commitment to the American Innovation and Manufacturing Act of 2020 that requires EPA to implement an HFC phasedown plan to reduce 2011–2013 levels 85 percent by 2036—meeting the goals of this agreement. Local and state codes will need to be modified to support HFC-free equipment. Increased federal funding to develop and deploy the next generation of refrigerants would help the United States to remain a leader in the manufacturing of heat pumps, refrigerators, and air conditioners, while helping the United States meet or exceed its target for reducing refrigerant emissions.
Embodied Carbon
As building energy demands continue to drop through significant design and engineering improvements, the energy and carbon costs of building material extraction, production, transportation, installation, and end-of-life disposal become more significant. In the most energy efficient buildings, the embodied carbon in building material selection can be equivalent to 30 years of operational energy (see Figure 7-4; Carbon Leadership Forum 2020). The largest contributors to embodied carbon in buildings are the extensive use of concrete, steel, aluminum, petroleum-based insulation, plastics, and disposable technologies. Consequently, the most significant strategies for reducing embodied carbon in buildings include (1) minimizing concrete and ensuring CarbonStar certification for what is used (CSA Group 2021); (2) 100 percent recycled content in steel and aluminum; (3) shifting to wood construction; (4) design for disassembly so that all steel, aluminum, glass and other materials can be reused without down-cycling; and (5) reusing existing buildings rather than new construction. Today, operational carbon and embodied carbon
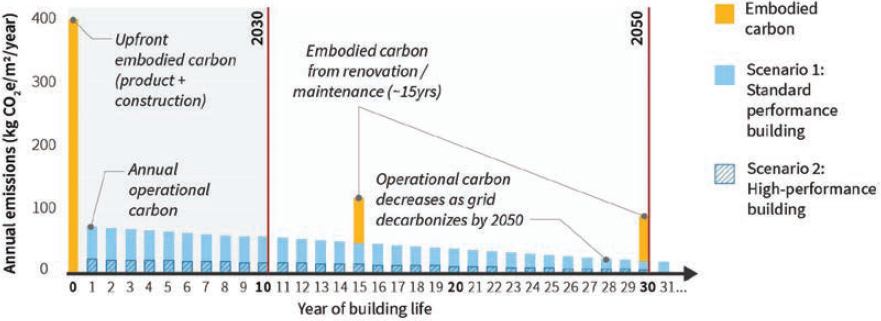
need to be on the same balance sheet, with cutting-edge tools emerging for comprehensive carbon accounting. New research and development are needed to advance design, engineering, and manufacturing of building assemblies and systems that can be produced with reduced GHG emissions (or even negative emissions in the case of some alternatives to concrete and steel).
Innovation for Building Electrification with Grid Integration
The electrification of existing heating, hot water, and gas appliances offers only modest reductions in energy demand, estimated at 0.9 quads in savings by 2050 (76 MMtCO2 reductions) (Ungar and Nadel 2019). However, if electrification in buildings is combined with high energy efficiency, on-site energy storage, and smart technologies with grid integration, the energy and GHG emissions reductions can be substantial. In new residential and commercial buildings, electrification often reduces the first cost of construction, with records of 27 percent lower upfront costs for an all-electric single-family new home (Group14 Engineering 2020). With the addition of smart technologies and on-site energy storage, peak loads are also reduced, supporting greater grid stability. The addition of time-of-day pricing and equipment controls, as well as batteries, can both reduce and align the “camel curves” of conventional electricity demand (high late afternoon demand) and the “duck curves” of renewable energy sources (photovoltaic [PV] displaced late afternoon demand). Increased federal and industry investments in RD&D in building-level thermal and electric energy storage, utility integration of distributed PV and energy storage, and
integrated heating/DHW/cooling/ventilation technologies can accelerate the relatively weak federal target for decarbonizing the built environment (see Figure 1-3). Success may also depend on significant input from the social sciences, beyond economics and behavioral analysis, to fully understand how to improve technology use and impact (Dietz et al. 2013; Gromet et al. 2013; Shove 2021; Stern et al. 1986; Sussman and Chikumbo 2016; and see Chapter 5).
Community Policies and Actions for Rapid Decarbonization in the United States
In addition to modifications to buildings for decarbonization, federal, state, and local policies could promote land use policies that substantially increase the energy efficiency of buildings and infrastructure as described in the next four sections on mixed-use, transit-oriented development; community renewables with micro-grids; smart surfaces; and innovation, research, and rapid development of district energy through GEBs with thermal energy distribution and storage systems.
5. Incentivize Mixed-Use Walkable Infill Instead of Sprawl Communities.
Sprawl is accompanied by infrastructure growth with both a significant carbon footprint and a long-term maintenance cost. A 2020 Transportation for America report identified that the nation’s largest 100 urban areas added 30,511 new freeway lane-miles of roads between 1993 and 2017, a 42 percent increase, while population only increased 32 percent (Bellis 2020). Each lane-mile of road costs between $4.2 million and $15.4 million to build and approximately $24,000 per year to maintain. States alone spent $500 billion annually to expand roads between 1993 and 2017, and the public road infrastructure grew by almost 224,000 miles between 2009 and 2017. The expanding road infrastructure was accompanied by a 50 percent increase in vehicle miles traveled over 25 years (AFDC 2022), with compounded impacts for air quality, health, equity, and family life. Moreover, with every mile of new roadway there are equivalent miles of electric, gas, water, and cable infrastructures, which have embodied carbon and long-term maintenance costs.
The CoolClimate Network has captured California’s household Carbon Footprints in 2010 and 2020 with a vision for 2030 and 2050 that relies heavily on rethinking land use (Figure 7-5), to include low-energy new housing, shifts in household diets, and rethinking transportation with walkability and transit (CoolClimate
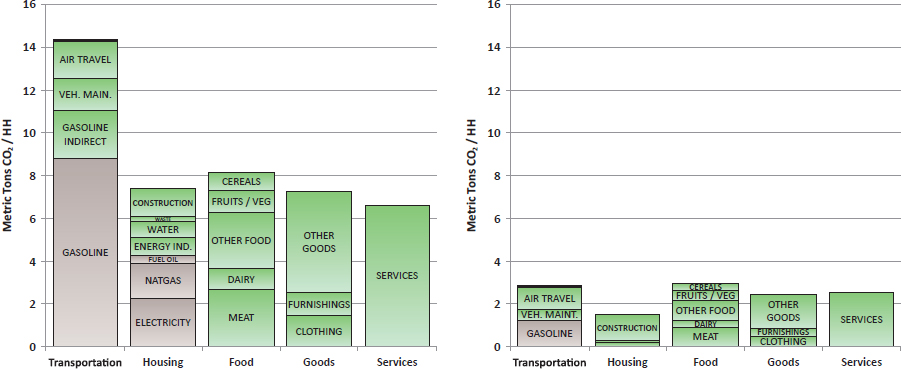
SOURCE: Adapted from Jones et al. (2018), https://doi.org/10.17645/up.v3i2.1218. CC BY 4.0.
Network n.d.). These actions could reduce California’s household total carbon footprint 11–45 tCO2e/y per household through a full menu of actions that significantly feature urban infill in transit-centric low-carbon zones (Figure 7-5; Jones et al. 2018).
A Review of Physical Planning and Transportation Impacts
Feng and Gauthier (2021) identified the range of environmental consequences of today’s subsidized sprawl beyond the growing demand for fossil or electric energy sources—including atmospheric pollution; hydrographic system alteration, increased impervious surfaces and flooding; and loss of biodiversity, forests, and agriculture. Additional social costs include increased spatial segregation, commuting time, and demands for automobile ownership, as well as diminished access to jobs and amenities.
The IRA and IIJA contain a number of programs that would modify urban and suburban land use policies, including $1.893 billion in a Neighborhood Access and Equity Grants Program (IRA §60501) to reconnect communities separated by highways and other infrastructure, and $1.5 billion for grants under the Urban and Community Forestry Assistance Program (IRA §23002) to promote tree planting in communities, both with priority given to underserved populations. However, these programs aim to fill particular needs, and would not be sufficient if the nation were to decide to transform the built environment to reduce emissions and achieve objectives related
to environmental justice (see Chapter 2), health (see Chapter 3), and quality of life (see Chapter 3). A shift in federal and state subsidies that presently incentivize sprawl alongside changes in zoning laws to support mixed-use infill in transit-oriented developments could transform our cities and towns into walkable, bikeable, transit-serviced, low-energy, landscape-rich communities with well-designed and maintainable public infrastructures.
6. Bundle Retrofits for Improved Energy Efficiency, Electrification, and On-Site Power Generation and Storage, at Both Building and Whole Community Scales.
The shift to all-electric buildings and transportation will lead to significant new demands on the electric system (Chapter 6), especially if we continue to rely on personal vehicle–centric mobility and inefficient buildings. Without more intentional policies, the new demands for electricity will increase the needed size of the electric system, including the power generation and transmission infrastructures that will impact rural and urban neighborhoods. Chapters 5 and 6 identify difficulties in siting electricity infrastructure as the single most likely point of failure for the climate and energy provisions in the IRA and IIJA. Delays in siting new transmission lines to support the increased demand for electricity from the shift to electric transport and heat pumps could result in fossil emissions that increase through the 2020s, making decarbonization look like a complete failure (Chapter 6). Thus, accelerating and tightening targets for increased building energy efficiency and energy load management, along with changes in the built environment that will reduce demand for automotive travel, would pay significant dividends beyond their direct effects on emissions (and overall costs of a decarbonized economy). By reducing the amount of new electricity infrastructure needed, these building energy efficiency and load management measures would reduce the number of new electricity infrastructure projects and increase the number of trained people available to facilitate each siting (Chapter 5). Combining electrification with 50 percent reduction in energy demand in both buildings and transportation would significantly improve health and quality of life (Chapter 3).
Moreover, bundling electrification with energy efficiency retrofits reduces the needed size and operational energy demands of the new electrical equipment such as heat pumps. This provides both direct and synergistic reductions in demand for new electricity. Adding rooftop solar and battery storage to the mix would provide additional synergies because the efficiency upgrades and reduced electrical demand reduces the size and expense of the rooftop system and batteries. All three would interact synergistically to decrease the amount of new generating capacity and distribution and transmission investments needed locally and regionally.
While the IRA, IIJA, and Justice40 Initiative provide significant resources to address community infrastructure improvements, they do not explicitly bundle these improvements into integrated community action plans. Bundling would provide additional synergies if deployed in community-scale retrofit packages—which could combine new community solar power projects with rooftop solar installations; heat pump DHW heaters with water saving fixtures; and heat pump heating and cooling with insulation, reglazing, and air tightness—in municipal as well as privately owned buildings. The community could also decrease automotive travel by offering tax and other incentives for employers and people to locate close to the municipal center, and by improving electric mass transit and corridors for walking and biking. These upgrades could reduce both directly and synergistically the new transmission needed by the municipality, provide direct revenue from the solar power generation, and provide a new source of employment. The synergies created by bundled community-scale retrofitting would provide significant benefits, not evident in separate analyses of each option alone.
The Lawrence Berkeley National Laboratory (LBNL) has analyzed how trade-offs and synergies among building efficiency, electrification, and the various options for electric power generation affect energy demand and greenhouse emissions. LBNL concluded that the most aggressive strategies would bundle building efficiency, electrification, and grid decarbonization to offer approximately 90 percent reductions in carbon emissions from the built environment by 2050 (Figure 7-6; Langevin et al. 2022).
A final advantage of bundling distributed photovoltaics, battery storage, and increased efficiency is that the bundled system could provide sufficient on-site electricity during short-duration power outages, which are increasingly frequent (Bowen et al. 2019; EIA 2021, 2023b). Concerns about reliability have contributed to the explosive increase in fossil fuel–powered standby generators (low-, medium-, and high-power gensets) with health and carbon consequences (CARB n.d.). DERs including rooftop and community solar and microgrids can make buildings more self-reliant and resilient to disruptions of service, although DERs also require significant coordination between customers and grid operators (NASEM 2018, 2021b, 2023).
7. Ensure Smart Surfaces for Carbon Reduction and Equity.
More than 25 percent of land in U.S. urban areas is impervious today, combining the areas of dark and impervious roofs, parking lots, streets, and sidewalks (Center for Sustainable Systems 2021; Nowak and Greenfield 2018). The most effective way to reduce the intensity of the urban heat island is to decrease the area of dark,
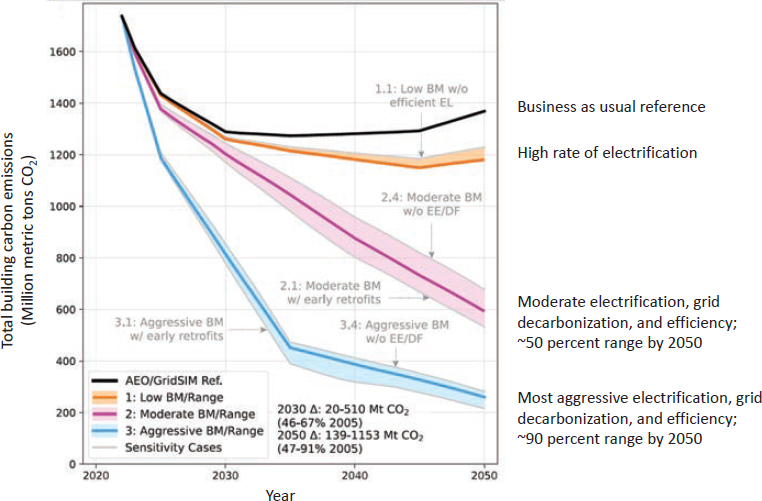
paved, concrete surfaces, and increase the number of reflective, vegetated, and porous surfaces, which reduce absorption of shortwave solar radiation, improve rainwater management, and support evaporative cooling (Strohbach et al. 2019; Taha 2021). Decreasing the use of concrete also reduces the associated carbon emissions. The integrated deployment of green, porous, and reflective surfaces, as well as trees and solar PV, allows cities to save money, mitigate and adapt to climate change, reduce public health and equity risks, and cut urban heat and flooding. The Smart Surfaces Coalition has quantified the major contributions of smarter surfaces to neighborhood quality of life, reducing urban heat island temperatures over 2°C, reducing flooding devastation, while also reducing or sequestering carbon and generating electricity (SSC 2022; Table 7-7).
Expansion of the electric grid and providing sufficient charging points for vehicles will force substantial changes in the built environment that will be challenging everywhere, but particularly in areas with high population density. The urban scape would be most resilient, walkable, and aesthetic if transmission lines were to move underground (out of the way of storms). The need for millions of new charge points
TABLE 7-7 CO2e Reduction Potential of Integrated Deployment of Smart Surfaces in Baltimore, Maryland
Intervention | Adoption Scenario | Climate Mitigation Mechanism | CO2e Reduction (metric tons) | CO2e Reduction as a Percent of Total City Emissions (from 2017) |
---|---|---|---|---|
Reflective Roofs | 80% low-slope | Negative radiative forcing | 2,860,000 | 1.9% |
20% steep-slope | Negative radiative forcing | 715,000 | 0.5% | |
Reflective Roads | 15% of road area | Negative radiative forcing | 748,000 | 0.5% |
Reflecting Parking Lots | 50% of parking area | Negative radiative forcing | 216,000 | 0.1% |
Trees | 40% tree coverage (11% increase) | Carbon sequestration and reduced cooling demand from shading | 345,000 | 0.2% |
Solar PV | 40% low-slope | Electricity generation | 8,907,000 | 6% |
20% steep-slope | Electricity generation | 3,492,000 | 2.3% |
NOTE: Integrated deployment of porous and reflective surfaces as well as trees and solar PV would reduce urban heat island temperatures more than 2°C, reduce flooding, and generate electricity to offset as much as 8 percent of Baltimore’s CO2 emissions. SOURCE: Data from Kats et al. (2022), https://smartsurfacescoalition.org/analysis/baltimore-report.
could be coordinated with other upgrades to streets and sidewalks, including the removal or repurposing of natural gas infrastructures; upgrades or repair of data, water, and waste infrastructures; storm water management; greater walkability; added greenspace; and improved electric mass transit.
8. Innovate and Deploy Community and District Thermal Energy Systems Including Combined Heating, Cooling, and Power. 4.0 quads of reduced annual energy demand, 150 MMtCO2 of reduced annual emissions.
There are abundant alternatives to the use of electricity for space heating, hot water, and even cooling loads that combined are responsible for 37 percent to 46 percent of the total energy consumed in the United States (EIA 2022a). Campus and city district thermal
energy systems distribute “waste” energy through shallow, insulated networks of hot water and chilled water, with a wide array of thermal conditioning sources, including
- Waste heat utilization from high cooling demand buildings and data center combined heat and power (CHP) for electric uninterrupted power supply and heating;
- Low-temperature geothermal (with heat pumps) and geo-exchange (closed loop exchange with the earth or bodies of water for conditioning);
- High-efficiency gas and biogas district heating systems;
- High-efficiency heat pump cooling, open and closed loop aquifer cooling;
- Waste to energy (highly managed waste, addressing a parallel national challenge);
- Regional CHP (reducing the 70 percent source to site thermal waste of power generation to 30 percent or less; Litjens et al. 2018); and
- Sewer mining.
District heating and cooling systems that combine ground source heat pumps with PV and building energy storage systems have resulted in major residential carbon savings in Europe and the United Kingdom, under climatic conditions present in many regions of the United States (Litjens et al. 2018). In a detailed study, Andrés et al. (2018) concluded that local, urban, and regional district energy systems have evolved significantly in the past 50 years, and can now supply low-energy thermal conditioning with substantially lower and more effective infrastructure costs. Real-world examples include waste heat recovery from data centers (Brunswick, Germany), sewage water (Nice, France), cooling systems in tertiary buildings (Madrid, Spain), and waste heat recovery underground railway stations (Bucharest, Romania). Despite examples in Minneapolis and other locations, relatively few district and community energy systems have been installed in the United States (DOE 2020b). Björnebo et al. (2018) concluded that small-scale district heating that replaces oil-fired heating in cool and cold climates would provide the largest GHG emissions reductions per dollar in the United States.
The United Nations Environment Programme (UNEP) concluded that modern district energy systems can achieve a 50 percent reduction in building conditioning energy demand through the full range of thermal energy sources, with corresponding reductions in CO2, SOx, NOx, particulates, mercury, and other pollutants from conventional fossil sources of heat and electric power (UNEP 2019). With data gathered from cities around the world, they quantified the CO2 emissions reductions from a variety of options, including geothermal (with heat pumps) and geo-exchange (no heat pumps), solar thermal, industrial waste heat recovery, data center heat recovery, waste to energy, district boilers, district chillers, combined heat and power
and combined heating, cooling (absorption chillers), and power (expanding on Riahi [2015]). District energy systems are predominantly piped infrastructures, and the Building Decarbonization Coalition, in public commentary to the committee,23 suggests that these new thermal infrastructures can provide a just transition for gas workers, using existing rights-of-way, and reengaging labor with state training.
Grid Actions for Integrating the Built Environment in Rapid Decarbonization
More than 70 percent of the nation’s electricity is consumed by or within a building (Figure 7-7a; DOE 2015; EIA 2023a; EPA 2023), and buildings offer the opportunity to play a critical role in supporting grid stability. Buildings create the largest peak demand on the electric grid, challenging both capacity during peak hours and effective renewable energy use during non-peak hours (Figure 7-7b; Hale et al. 2018). Moreover, peak demands that typically emerge in summer heat waves will be outpaced by peak demands in winter cold snaps as heating loads are shifted to electric sources.
In addition to ensuring that new transmission infrastructures improve communities, there are two key opportunities that depend on integration of the grid and the built environment beyond a simple utility service: advancing buildings (and their cars) as batteries and peak load managers for grid resilience; and incorporating distributed renewables and district energy with GEBs into an interconnected grid of grids.
9. Advance Buildings as Batteries and Peak Load Managers.
Buildings can be critical partners in reducing and shifting electricity demand, supporting distributed renewables with load shifting and storage, and extracting low-temperature energy to replace electricity. Because of the size of their peak and total demand for electricity, buildings disproportionately shape the overall needs of the electric system. However, with deep efficiency, the growth of rooftop and community renewables and storage, and replacement of some uses of electricity with low-quality thermal energy, buildings could become critical active partners in managing peak electrical loads, rather than being simply one-way users of the grid. All of the
___________________
23 Joanna Partin, Building Decarbonization Coalition, October 21, 2022; https://www.nationalacademies.org/event/10-20-2022/accelerating-decarbonization-in-the-united-states-technology-policy-and-societal-dimensions-perspectives-on-priority-actions-for-the-built-environment-and-building-technologies-rd-d-needs.
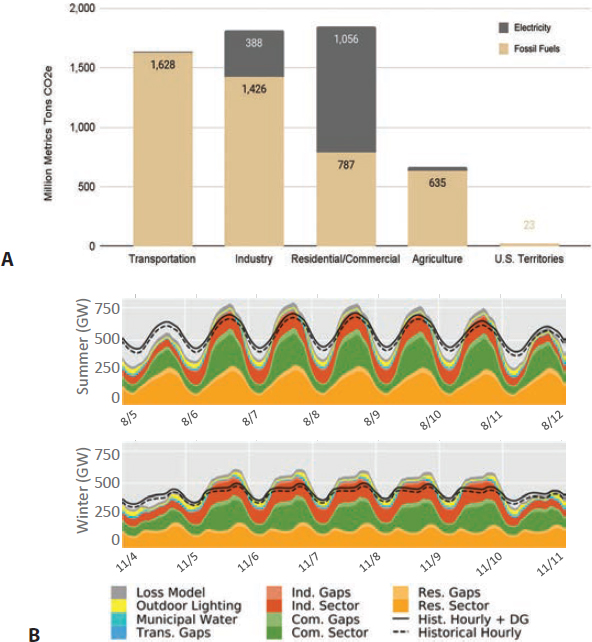
innovations needed for fully engaging buildings in grid management are not yet on the shelf. (See NASEM 2021b.) To realize the potential of buildings as batteries and peak load managers for grid resilience, the United States would need to increase long-term RD&D investments in a number of technologies, including
- Heat pumps for integrated heating, cooling, and hot water (buildings);
- Elimination of “parasitic or vampire” miscellaneous electric loads (buildings);
- Solar DHW and energy storage systems (buildings);
- Grid-connected smart building systems—equipment, appliances, controls (buildings);
- Geothermal heat pumps for heating, cooling, hot water (communities);
- Grid-connected Building Electric and Thermal Energy Storage systems (communities);
- Grid-integrated distributed batteries (i.e., car batteries and building thermal and electric batteries) that can run homes or feed the grid (communities);
- Building and community distributed renewables with deeply efficient buildings; and
- New grid infrastructures that meet multiple performance and decarbonization goals.
10. District, Community, and Building Renewables with GEBs.
The IRA dedicates $65 billion to funds for grid reliability and resiliency actions and supports a Grid Deployment Office for critical minerals and supply chains for clean energy technology; key technologies including carbon capture, hydrogen, direct air capture, and energy efficiency; and energy demonstration projects outlined in the bipartisan Energy Act of 2020. These federal funding opportunities do not include a focused RD&D program to develop grids that fully engage distributed renewables, storage, and GEBs as an integral player in the grid. Such a program would encompass a range of technological advances: V2G (vehicle to grid) bi-directional charging to store up to 80 to 100 kilowatt-hours of electricity, sufficient to run a U.S. home for 2–3 days (Blumsack 2022); ice storage, water storage, and phase change materials that can provide long-term cooling for buildings with off-peak power; smart time-of-day controls for appliances and equipment; and new approaches to reducing AC to DC conversion losses through strategic management or replacement with direct DC from PV. The potential of these technologies would be invaluable to shave and shift peak loads and avoid brownouts and blackouts. A grid that flexibly integrates building and community renewable energy supplies would help the nation meet its renewable energy goals and advance resiliency.
The barriers to rapid advances in building-scale PV systems are both regulatory and profit driven. In the United States, soft costs (including installation labor, customer acquisition through sales and marketing, and permitting/inspection/interconnection) are more than 65 percent of the overall costs of rooftop solar panels (EERE 2023). Reducing soft costs and increasing incentives for distributed PV will spur U.S. manufacturing and installation jobs and bring the United States in line with international gains in DERs (Birch 2018).
Community-owned renewables are emerging across the United States, led by special-purpose community entities, the local utility, or a third party (which might be a private developer or nonprofit organization). Minnesota’s community solar comprises more than 70 percent of the state’s total solar photovoltaic capacity of
1,057 megawatts, followed by 18 percent of New York State’s 3,950 megawatts, and 15 percent of Massachusetts’s total solar capacity of 2,805 megawatts (Heeter et al. 2021; McCoy 2022). HUD has updated guidance to support community solar on public and assisted multifamily housing (HUD 2023), and multiple states have launched low- and moderate-income solar programs to facilitate community solar by helping to overcome barriers such as lack of access to capital, insufficient tax burden to take advantage of tax credits, the large fraction of renters who are often ineligible for incentives, frustrating interconnection policies, and lack of familiarity with solar products (Mai et al. 2018).
Given their growing popularity, building- and community-scale renewables can be critical partners in the expansion of the electric grid. To date, the United States has been focused on utility-scale renewables with the lowest cost and legislative challenges, rather than those likely to be easiest to site (Chapter 5). With national and utility leadership, Europe and Australia have far more successfully integrated building and community renewables into the grid, as shown for Germany in Figure 7-8.
In the United States, 20 states and the District of Columbia (DC) have laws ensuring that consumers can participate in community solar power generation, 28 states authorize or allow third-party Power Purchase Agreements for solar PV, and 39 states (plus DC, American Samoa, Guam, Puerto Rico, and the U.S. Virgin Islands) have mandatory net metering rules (Heeter et al. 2019, pp. 28 and 30, 2021). Extensions of these programs to all states would facilitate deployment of DERs and keep the United States from falling behind at a time in which the future architecture of the grid is in development. Interconnected building- and community-owned renewable electricity, from wind and photovoltaic installations, can be designed to act as a microgrid to operate both with the regional grid or independently, as needed. Microgrids can increase reliability in the event of power outages owing to hurricanes and other natural and human-made disasters, and can provide electricity access, jobs, and revenues in poor, rural, and isolated communities.
Fully engaging the electricity industry and its regulatory bodies in the critical frontier of GEBs is a strategic opportunity for decarbonizing the United States. DOE, ACEEE, GSA, and RMI have released a national roadmap that illustrates the potential and the urgency of integrating buildings into the future grid, moving well beyond grid-communicating buildings to grid interactive for reducing, shifting, storing, and offsetting energy loads with innovation (Figure 7-9; Dean et al. 2021; Satchwell et al. 2021).
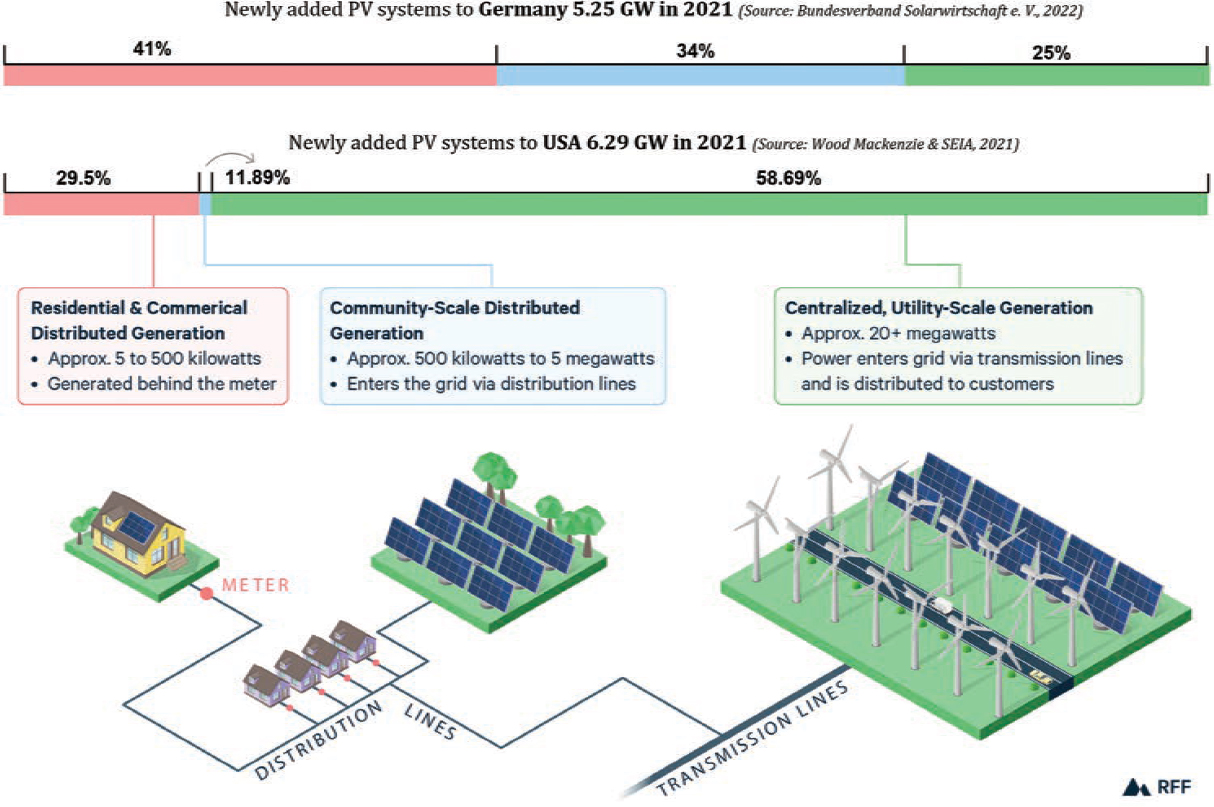
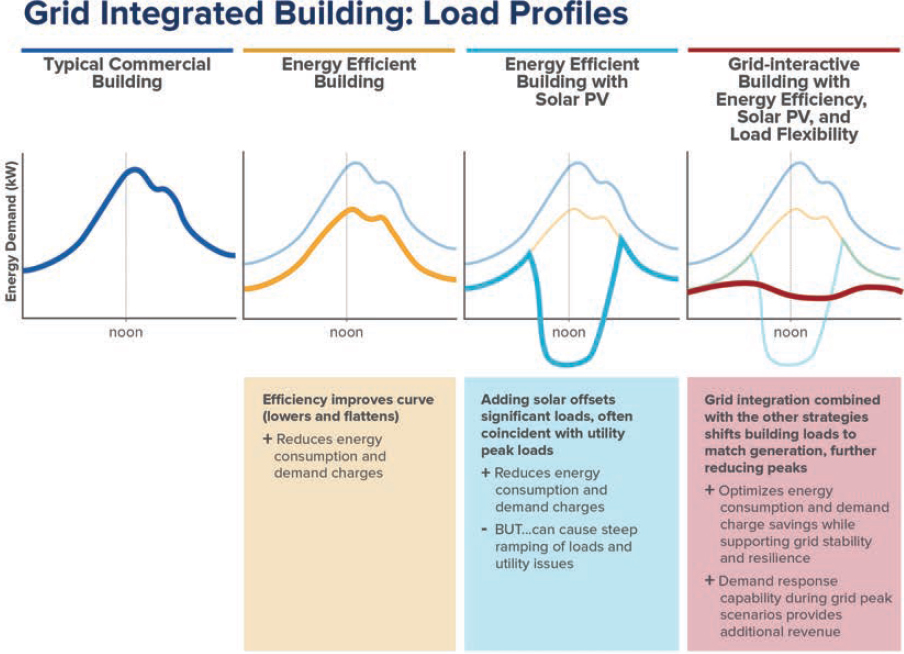
Summary of 10 Actions
Consistent long-term federal and subnational leadership and incentives, combined with subnational mandates, could significantly accelerate the pace of emissions reductions associated with the built environment. For this to succeed, there would need to be clarity and coordination across federal, state, and local building programs, with a commitment to ensure equity across all programs and policies. Significant reductions in demand could be ensured by (1) increased regulatory rigor for buildings and equipment, including accelerated federal appliance and equipment efficiency standards, especially with electrification; (2) subnational mandates and incentives for zero energy new homes and commercial buildings; and (3) accelerated retrofits of existing homes and commercial buildings, with benchmarking
and reporting. Research has also demonstrated that the ability of incentives and other strategies to increase adoption of technologies and their effective use to reduce energy consumption can be significantly enhanced through appropriate use of social and behavior insights to inform user-centered and community-centered design (Dietz et al. 2013; Gromet et al. 2013; Stern et al. 1986; Sussman and Chikumbo 2016). Federal and subnational entities adopting new incentives or rules should be sensitive to and consult social and behavioral experts about the variety of non-financial design elements that can dramatically improve long-term efficiency gains for the same financial investment.
There is an equally critical need for national investment in RD&D of low-carbon and smart technologies, and systems for homes, commercial buildings, and GEBs with distributed renewables and storage. Beyond the building level, there is the challenging but transformational pursuit of community-level decarbonization of buildings and community infrastructures, including incentivizing mixed-use walkable infill instead of sprawl communities; accelerating building and community renewables; and innovating in addressing low-temperature thermal (heating and cooling demands) to preserve electricity for higher and better uses. The extent of the carbon savings in the built environment and the cost-effectiveness among decarbonization strategies should further elevate the ongoing commitment to investment, policy, and innovation at the building, community, and grid-levels.
Recommendation 7-6: Increase Research, Development, Demonstration, and Deployment for Built Environment Decarbonization Interventions. Congress should provide funding in support of a Department of Energy (DOE)-led research agenda on the wide-ranging operational and embodied energy savings; greenhouse gas emissions reductions, including refrigerants; and socioeconomic outcomes of different strategies for implementing advanced building and community decarbonization technologies. DOE and state research and development agencies and public utility commissions should expand their budgetary and regulatory support (respectively) for community-level pilots and demonstrations for distributed renewable electric and thermal installations, including district energy systems and grid-integrated efficient buildings. These should include multi-disciplinary socioeconomic strategies to inform the implementation approaches best suited for different communities, including through the potential of alternative ownership strategies over distributed energy and transmission.
BEYOND THE NEXT 10 YEARS: EVALUATION AND GROWTH
The built environment is composed of a range of buildings and infrastructure, and is designed, built, and maintained by a diverse set of service providers such as developers, builders, and remodelers, along with the range of energy auditors, product manufacturers, retail and supplier distributing building inspectors, real estate agents, lenders, appraisers, and title agents, to name a few of the stakeholders in addition to building owners, occupants, and tenants that are vested in current building practices (Hassel et al. 2003; Martín and McCoy 2019). Many of these same actors would need to be trained, educated, or otherwise made aware of the long-term decarbonization goals for individual buildings and properties—a challenge that is further discussed in Chapter 4.
The planning, design, construction, maintenance, and disposition of human settlement and the built environment defines a significant portion of energy demand. Yet this sector collectively retains a unique social, technological, and economic role in the United States in addition to their energy and environmental consequences. Construction and real estate transactions have averaged 8.4 percent of U.S. gross domestic product over the past half-century (U.S. Bureau of Economic Analysis 2022). From our individual homes and workplaces, through our neighborhoods, and to the greater metropolitan and regional geographies that define our collective sense of place and daily social and economic activities, the built environment’s demand for energy has evolved in complex way.
A broad range of public policies and private decisions associated with the built environment significantly define how, how much, and what kind of energy is consumed in the United States. For example, improvements in energy efficiency within new buildings have modestly offset the sheer growth in these buildings’ numbers. National decarbonization strategies should undoubtedly consider the multifaceted role that the built environment plays in defining the speed, quality, equity, and consequences of current and future energy transitions in the history of this sector’s carbon-based development. In the same way that the transformation for electrification and energy provision in buildings over a century ago required substantial coordination across these groups, so is its decarbonization (Hughes 1993). Indeed, as this chapter describes, there is a much greater potential for emissions reductions from the built environment with even more ambitious strategies for reducing energy demand and more tightly weaving building technologies into the broader energy system.
The IRA, the IIJA, and state and local policies have initiated this transition and suggest ways in which the committee’s first recommendations need to be revised.
Indeed, the first report’s recommendations for individual buildings remain relevant, but require
- More rigor in the form of advanced targets for both the current decade and through 2050;
- Attention to the market transformations, incentives, and other voluntary transitions that must occur among current built environmental sectors, particularly by the end of the first decade to ensure sufficient evidence of their outcomes and feasibility;
- Articulation of the pivot from voluntary incentives such as those established in the IIJA and IRA for the first decade to mandatory decarbonization across new and existing development leading up to 2050; and
- Consideration of the interactions across intervention types and their resulting contributions to both comprehensive decarbonization of the sector and the inequitable access and impacts across populations—particularly for low-income, energy-burdened, energy-insecure households; households in severely inadequate housing; and other disadvantaged groups.
For the next decade, investments in market transformation across all building and community intervention points are needed to meet the goal of reducing the built environment’s contribution to carbon-based energy demand and its resulting GHG emissions by 2050. Public-sector investments are most needed for workforce training, for fomenting of building company specialization and property fuel provider transition, and in consumer awareness and incentives for existing building retrofits. The market transformation must be conscious of but not neglect the need to increasingly require all intervention types for new development over the same time. Increasing national appliance and equipment standards for efficiency and electrification goals are included in this immediate regulatory framework, as are requirements of federally funded public works, federal facilities, and federally assisted housing.
After the next decade, increasing requirements by subnational governments on developments—and consequently sequenced national regulations with technical assistance and other resources for subnational governments—will be needed to complete transformations for all existing construction in the built environment using all intervention types by 2050. However, the rollout of the newest federal, state, and local policies along with the continued innovation and experimentation of the building and land use industries over the next decade are certainly setting us on the course. The question then becomes: what happens in 2033?
In both the market transformations and regulations sequenced to reach 2035 and 2050 targets, more resources—including direct grants and service provision—will be needed to assist low- and moderate-income households and those living in historically disadvantaged communities. These resources must be allocated simultaneous to market incentives for ensuring that these communities benefit from the transformations, and to regulations for ensuring that they are not punitively burdened by lack of compliance. The resources can come through existing assistance programs for the lowest-income households, such as WAP, if they are armed with ample resources, more flexible eligibility requirements, broader intervention action allowances, improved outreach and community engagement protocols, and safeguards to minimize the adverse effects of improvements such as housing unaffordability or displacement. Expanded product and service rebates and tax credits with similar changes but for a wider population of low- and moderate-income households will ensure comprehensive diffusion of all building decarbonization intervention types for these communities.
Recommendation 7-7: Extend Current Decarbonization Incentives Beyond the Next Decade While Scaling Up Mandates. By 2033, Congress should continue appropriation for rebates and tax credits of residential and commercial decarbonization to incentivize voluntary upgrades for energy efficiency and electrification for an additional decade. Larger incentives must be allotted to building audit services and to owner and occupant service management to ensure the technologically appropriate selection and installation order of decarbonization technologies that are suited to the housing unit and household or owner capacity. For example, the creation of additional targets for 2040–2043 could ensure a higher adoption rate of heat pumps beyond the 25 percent and 15 percent targets laid out in the committee’s first report for residential and commercial buildings by 2030. The total appropriation could be equivalent to the funding provided in the Inflation Reduction Act (IRA), but the per household rebate caps and eligible activities should be redesigned based on evaluation of the IRA’s outcomes. Extensions to tax credits for both residential and commercial improvements could also be extended just as the IRA extended these from their previous enactment. Furthermore, Congress should increase support for Weatherization Assistance Program (WAP) services by an order of magnitude equivalent to meet the needs of decarbonizing all WAP-eligible households living in homes that use carbon-based energy in the next decade (approximately $33.8 billion annually).
Table 7-8 summarizes all the recommendations in this chapter to support decarbonizing the built environment.
SUMMARY OF RECOMMENDATIONS ON THE BUILT ENVIRONMENT
TABLE 7-8 Summary of Recommendations on the Built Environment
Short-Form Recommendation | Actor(s) Responsible for Implementing Recommendation | Sector(s) Addressed by Recommendation | Objective(s) Addressed by Recommendation | Overarching Categories Addressed by Recommendation |
---|---|---|---|---|
7-1: Ensure Clarity and Consistency for the Implementation of Building Decarbonization Policies | Department of Energy (DOE) |
|
|
Rigorous and Transparent Analysis and Reporting for Adaptive Management Ensuring Procedural Equity in Planning and Siting New Infrastructure and Programs Tightened Targets for the Buildings and Industrial Sectors and a Backstop for the Transport Sector |
7-2: Promote an Equitable Focus Across Building Decarbonization Policies | DOE |
|
|
Ensuring Procedural Equity in Planning and Siting New Infrastructure and Programs Tightened Targets for the Buildings and Industrial Sectors and a Backstop for the Transport Sector |
Short-Form Recommendation | Actor(s) Responsible for Implementing Recommendation | Sector(s) Addressed by Recommendation | Objective(s) Addressed by Recommendation | Overarching Categories Addressed by Recommendation |
---|---|---|---|---|
7-3: Expand and Evaluate the Weatherization Assistance Program | DOE |
|
|
Rigorous and Transparent Analysis and Reporting for Adaptive Management Ensuring Procedural Equity in Planning and Siting New Infrastructure and Programs Tightened Targets for the Buildings and Industrial Sectors and a Backstop for the Transport Sector |
7-4: Coordinate Subnational Government Agencies to Align Decarbonization Policies and Implementation | State and municipal government offices |
|
|
Tightened Targets for the Buildings and Industrial Sectors and a Backstop for the Transport Sector Building the Needed Workforce and Capacity |
Short-Form Recommendation | Actor(s) Responsible for Implementing Recommendation | Sector(s) Addressed by Recommendation | Objective(s) Addressed by Recommendation | Overarching Categories Addressed by Recommendation |
---|---|---|---|---|
7-5: Build Capacity for States and Municipalities to Adopt and Enforce Increased Regulatory Rigor for Buildings and Equipment | Congress |
|
|
A Broadened Policy Portfolio Rigorous and Transparent Analysis and Reporting for Adaptive Management Tightened Targets for the Buildings and Industrial Sectors and a Backstop for the Transport Sector Building the Needed Workforce and Capacity |
7-6: Increase Research, Development, Demonstration, and Deployment for Built Environment Decarbonization Interventions | Congress |
|
|
Siting and Permitting Reforms for Interstate Transmission Research, Development, and Demonstration Needs |
7-7: Extend Current Decarbonization Incentives Beyond the Next Decade While Scaling Up Mandates | Congress; DOE |
|
|
A Broadened Policy Portfolio Tightened Targets for the Buildings and Industrial Sectors and a Backstop for the Transport Sector |
REFERENCES
ACEEE (American Council for an Energy-Efficient Economy). 2011. “How Does Energy Efficiency Create Jobs?” Fact Sheet. https://www.aceee.org/files/pdf/fact-sheet/ee-job-creation.pdf.
ACEEE. 2014. “Residential Energy Use Disclosure: A Guide for Policymakers.” https://www.aceee.org/toolkit/2014/01/residential-energy-use-disclosure-guide-policymakers.
ACEEE. 2022. “Home Energy Upgrade Incentives: Programs in the Inflation Reduction Act and Other Recent Federal Laws.” Policy Brief. https://www.aceee.org/sites/default/files/pdfs/home_energy_upgrade_incentives_9-27-22.pdf.
AFDC (Alternative Fuels Data Center). 2022. “Annual Vehicle Miles Traveled in the United States.” Updated June 2022. Department of Energy. https://afdc.energy.gov/data/10315.
Allcott, H., and M. Greenstone. 2012. “Is There an Energy Efficiency Gap?” Journal of Economic Perspectives 26(1):3–28. https://doi.org/10.1257/jep.26.1.3.
Andrés, M., M. Regidor, A. Macía, A. Vasallo, and K. Lygnerud. 2018. “Assessment Methodology for Urban Excess Heat Recovery Solutions in Energy-Efficient District Heating Networks.” Energy Procedia. 16th International Symposium on District Heating and Cooling, DHC2018, 9–12 September 2018, Hamburg, Germany, 149(September):39–48. https://doi.org/10.1016/j.egypro.2018.08.167.
Architecture 2030. 2023. “The 2030 Challenges.” Architecture 2030. https://architecture2030.org/the2030challenges.
ASAP (Appliance Standards Awareness Project). 2023. “National.” Appliance Standards Awareness Project. https://appliance-standards.org/national.
Azerbegi, R. 2022. “The Inflation Reduction Act and Its Impact on Your Next Building Project.” Mead and Hunt. https://meadhunt.com/ira-impact-building-projects.
Barbour, N. 2021. “Smart Thermostats Gain Traction in US, Point to Modest Electricity Savings.” S&P Global Market Intelligence (blog), July 20. https://www.spglobal.com/marketintelligence/en/news-insights/blog/smart-thermostats-gain-traction-in-us-point-to-modest-electricity-savings.
BDC (Building Decarbonization Coalition). 2023. “Zero Emission Building Ordinances.” Building Decarbonization Coalition. https://buildingdecarb.org/zeb-ordinances.
Bellis, R. 2020. “The Congestion Con: How More Lanes and More Money Equals More Traffic.” Transportation for America. https://t4america.org/wp-content/uploads/2020/03/Congestion-Report-2020-FINAL.pdf.
Berg, W. 2022. “Leading States Chart Path for Cutting Emissions with Electrification, Pointing Way for Peers.” ACEEE, July 21. https://www.aceee.org/blog-post/2022/07/leading-states-chart-path-cutting-emissions-electrification-pointing-way-peers-1.
Berg, W., S. Vaidyanathan, B. Jennings, E. Cooper, C. Perry, M. DiMascio, and J. Singletary. 2020. The 2020 State Energy Efficiency Scorecard. Washington, DC: ACEEE. https://www.aceee.org/research-report/u2011.
Berg, W., E. Cooper, and M. DiMascio. 2022. State Energy Efficiency Scorecard: 2021 Progress Report. Washington, DC: ACEEE. aceee.org/research-report/u2201.
Birch, A. 2018. “How to Halve the Cost of Residential Solar in the US.” Greentech Media, January 5. https://www.greentechmedia.com/articles/read/how-to-halve-the-cost-of-residential-solar-in-the-us.
Biswas, S., A. Echevarria, N. Irshad, Y. Rivera-Matos, J. Richter, N. Chhetri, M.J. Parmentier, and C.A. Miller. 2022. “Ending the Energy-Poverty Nexus: An Ethical Imperative for Just Transitions.” Science and Engineering Ethics 28(4):36. https://doi.org/10.1007/s11948-022-00383-4.
Björnebo, L., S. Spatari, and P.L. Gurian. 2018. “A Greenhouse Gas Abatement Framework for Investment in District Heating.” Applied Energy 211:1095–1105. https://doi.org/10.1016/j.apenergy.2017.12.003.
Blumsack, S. 2022. “Can My Electric Car Power My House? Not Yet for Most Drivers, But Vehicle-to-Home Charging Is Coming.” Fast Company, March 31. https://www.fastcompany.com/90736485/can-my-electric-car-power-my-house-not-yet-for-most-drivers-but-vehicle-to-home-charging-is-coming.
Bowen, T., I. Chernyakhovskiy, and P.L. Denholm. 2019. “Grid-Scale Battery Storage: Frequently Asked Questions.” NREL/TP-6A20-74426, 1561843. https://doi.org/10.2172/1561843.
Brown, M., L. Berry, and L. Kinney. 1994. Weatherization Works: Final Report of the National Weatherization Evaluation. Oak Ridge, TN: Oak Ridge National Laboratory. ORNL/CON-395. https://technicalreports.ornl.gov/cppr/y2001/rpt/109939.pdf.
Bundesverband Solarwirtschaft e.V. 2022. “2021: Zehn Prozent mehr Solarmodule installiert.” January 3. https://www.solarwirtschaft.de/2022/01/03/2021-zehn-prozent-mehr-solarmodule-installiert.
C40 Cities Climate Leadership Group and C40 Knowledge Hub. 2019. “How to Use Reporting and Disclosure to Drive Building Energy Efficiency.” C40. April. https://www.c40knowledgehub.org/s/article/How-to-use-reporting-and-disclosure-to-drive-building-energy-efficiency?language=en_US.
California Energy Commission. 2023. “Building Initiative for Low-Emissions Development Program.” https://www.energy.ca.gov/programs-and-topics/programs/building-initiative-low-emissions-development-program.
CARB (California Air Resources Board). n.d. “Use of Back-Up Engines for Electricity Generation During Public Safety Power Shutoff Events.” https://ww2.arb.ca.gov/resources/documents/use-back-engines-electricity-generation-during-public-safety-power-shutoff. Accessed March 31, 2023.
Carbon Leadership Forum. 2020. “1—Embodied Carbon 101.” https://carbonleadershipforum.org/embodied-carbon-101.
Carmichael, C., M. Jungclaus, P. Keuhn, and K.P. Hydras. 2019. “Value Potential for Grid-Interactive Efficient Buildings in the GSA Portfolio: A Cost-Benefit Analysis.” RMI and GSA. https://rmi.org/wp-content/uploads/2019/07/value-potential-grid-integrated-buldings-gsa-portfolio.pdf.
Center for Sustainable Systems, University of Michigan. 2021. “U.S. Cities Factsheet.” Pub. No. CSS09-06.
Cleveland, C.J., P. Fox-Penner, M.J. Walsh, M. Cherne-Hendrick, S. Gopal, J.R. Castigliego, and T. Perez, et al. 2019. “Carbon Free Boston Summary Report.” Boston University Institute for Sustainable Energy. https://www.bu.edu/igs/2019/01/29/carbon-free-boston-summary-report.
Climate and Clean Air Coalition. 2020. HydroFlurocarbons (HFCs). https://www.ccacoalition.org/en/slcps/hydrofluorocarbons-hfcs.
Cluett, R., J. Amann, and S. Ou. 2016. Building Better Energy Efficiency Programs for Low-Income Households. Washington, DC: American Council for an Energy-Efficient Economy. Report No. A1601. https://www.aceee.org/sites/default/files/publications/researchreports/a1601.pdf.
Cohn, C., and N.W. Esram. 2022. Building Electrification: Programs and Best Practices. Washington, DC: American Council for an Energy-Efficient Economy. https://www.aceee.org/research-report/b2201.
CoolClimate Network. n.d. “California Carbon Footprints Under Climate Targets v2.0.” https://cal.maps.arcgis.com/apps/MapSeries/index.html?appid=2c0d0d53239c45ba980211842837cc32. Accessed March 31, 2023.
CPUC (California Public Utilities Commission). n.d. “Zero Net Energy.” Cpuc.ca.Gov. https://www.cpuc.ca.gov/industries-and-topics/electrical-energy/demand-side-management/energy-efficiency/zero-net-energy. Accessed August 10, 2023.
CSA Group. 2021. “CSA SPE-112:21 | CarbonStar®: Technical Specification for Concrete Carbon Intensity Quantification and Verification.” CSA Group. https://www.csagroup.org/store/product/CSA%20SPE-112%3A21.
Dean, J., P. Voss, D. Gagne, D. Vasquez, and R. Langner. 2021. “Blueprint for Integrating Grid-Interactive Efficient Building (GEB) Technologies into U.S. General Services Administration Performance Contracts.” NREL/TP-7A40-78190, 1784273, MainId:32099. https://doi.org/10.2172/1784273.
Deetjen, T.A., L. Walsh, and P. Vaishnav. 2021. “US Residential Heat Pumps: The Private Economic Potential and Its Emissions, Health, and Grid Impacts.” Environmental Research Letters 16(8):084024. https://doi.org/10.1088/1748-9326/ac10dc.
Department of State. 2022. “U.S. Ratification of the Kigali Amendment.” https://www.state.gov/u-s-ratification-of-the-kigali-amendment.
DOE (Department of Energy). 2011. “Saving Money by Saving Energy (Fact Sheet).” DOE/GO-102011-3280, 1024064. https://doi.org/10.2172/1024064. Accessed August 10, 2023.
DOE. 2015. Chapter 5, “Increasing Efficiency of Building Systems and Technologies.” In Quadrennial Technology Review 2015. Washington, DC.
DOE. 2016. “10 CFR Parts 429 and 430 [Docket No. EERE-2016-BT-TP-0029] RIN 1904-AD71 Energy Conservation Program: Test Procedures for Central Air Conditioners and Heat Pumps.” Office of Energy Efficiency and Renewable Energy, Department of Energy. Final Rule.
DOE. 2019. “Low-Income Household Energy Burden Varies Among States—Efficiency Can Help in All of Them.” https://www.energy.gov/sites/prod/files/2019/01/f58/WIP-Energy-Burden_final.pdf.
DOE. 2020a. “Low-Income Energy Affordability Data (LEAD) Tool.” https://lead.openei.org/docs/LEAD-Factsheet.pdf.
DOE. 2020b. “Minnesota Implementation Model—Combined Heat and Power Action Plan.” https://www.energy.gov/scep/slsc/articles/minnesota-implementation-model-combined-heat-and-power-action-plan.
DOE. n.d. “Residential Cold Climate Heat Pump Challenge.” https://www.energy.gov/eere/buildings/residential-cold-climate-heat-pump-challenge.
DOE Office of Policy. 2022. “The Inflation Reduction Act Drives Significant Emissions Reductions and Positions America to Reach Our Climate Goals.” Department of Energy. DOE/OP-0018. www.energy.gov/sites/default/files/2022-08/8.18%20InflationReductionAct_Factsheet_Final.pdf.
DOS and EOP (Department of State and Executive Office of the President). 2021. The Long-Term Strategy of the United States: Pathways to Net-Zero Greenhouse Gas Emissions by 2050. Washington, DC. https://www.whitehouse.gov/wp-content/uploads/2021/10/US-Long-Term-Strategy.pdf.
Drehobl, A. 2020. “Weatherization Cuts Bills and Creates Jobs But Serves Only a Tiny Share of Low-Income Homes.” American Council for an Energy-Efficient Economy. https://www.aceee.org/blog-post/2020/07/weatherization-cuts-bills-and-creates-jobs-serves-only-tiny-share-low-income.
Drehobl, A., L. Ross, and R. Ayala. 2020. How High Are Household Energy Burdens? Washington, DC: American Council for an Energy-Efficient Economy. https://www.aceee.org/research-report/u2006.
EERE (Department of Energy Office of Energy Efficiency and Renewable Energy). 2022a. “How Historic Weatherization Investments Will Make Life Better for Low-Income Families.” https://www.energy.gov/eere/articles/how-historic-weatherization-investments-will-make-life-better-low-income-families.
EERE. 2022b. “Weatherization Assistance Program Fact Sheet.” DOE/EE-2124. https://www.energy.gov/sites/default/files/2022-06/wap-fact-sheet_0622.pdf.
EERE. 2023. “Soft Costs: Solar Energy Technologies Office.” https://www.energy.gov/eere/solar/soft-costs.
EIA (U.S. Energy Information Administration). 2013. “2009 RECS Survey Data.” https://www.eia.gov/consumption/residential/data/2009.
EIA. 2018. “2015 RECS Survey Data.” May. https://www.eia.gov/consumption/residential/data/2015.
EIA. 2021. “U.S. Electricity Customers Experienced Eight Hours of Power Interruptions in 2020.” https://www.eia.gov/todayinenergy/detail.php?id=50316.
EIA. 2022a. “2018 CBECS Survey Data.” https://www.eia.gov/consumption/commercial/data/2018/index.php?view=consumption.
EIA. 2022b. “2020 RECS Survey Data.” May. https://www.eia.gov/consumption/residential/data/2020/hc/pdf/HC%206.1.pdf.
EIA. 2022c. Monthly Energy Review: 2. Energy Consumption by Sector. December. https://www.eia.gov/totalenergy/data/monthly/pdf/sec2.pdf.
EIA. 2022d. “Today in Energy: Homes and Buildings in the West and Northeast Have the Largest Share of Small-Scale Solar.” https://www.eia.gov/todayinenergy/detail.php?id=54379.
EIA. 2023a. “Electric Power Monthly—Table 2.1A Energy Consumption: Residential, Commercial, And Industrial Sectors.” https://www.eia.gov/electricity/monthly/epm_table_grapher.php.
EIA. 2023b. “Electric Power Monthly—Table B.1 Major Disturbances and Unusual Occurrences, Year-to-Date 2023.” https://www.eia.gov/electricity/monthly/epm_table_grapher.php.
EIA. 2023c. “Energy Consumption by Sector.” https://www.eia.gov/totalenergy/data/monthly/pdf/sec2.pdf.
Energy Saver. 2022. “Heat Pump Systems.” Department of Energy Office of Energy Efficiency and Renewable Energy. https://www.energy.gov/energysaver/heat-pump-systems.
EnergyStar. n.d.(a). “Energy Efficiency Program Sponsor Frequently Asked Questions About ENERGY STAR Smart Thermostats.” https://www.energystar.gov/products/heating_cooling/smart_thermostats/smart_thermostat_faq. Accessed August 10, 2023.
EnergyStar. n.d.(b). “When Is It Time to Replace?” www.energystar.gov/saveathome/heating_cooling/replace.
Energymag n.d. “Daily Energy Demand Curve.” https://energymag.net/daily-energy-demand-curve.
EPA (Environmental Protection Agency). 2023. “Inventory of U.S. Greenhouse Gas Emissions and Sinks: 1990–2021.” EPA 430-D-23-001. https://www.epa.gov/ghgemissions/draft-inventory-us-greenhouse-gas-emissions-and-sinks-1990-2021.
Evans, B. 2023. “IRA Update: The New Economics for Allowing Public Sector Buildings to Go Green.” U.S. Green Building Council. April 25. https://www.usgbc.org/articles/ira-update-new-economics-allowing-public-sector-buildings-go-green.
Feng, Q., and P. Gauthier. 2021. “Untangling Urban Sprawl and Climate Change: A Review of the Literature on Physical Planning and Transportation Drivers.” Atmosphere 12(5):547. https://doi.org/10.3390/atmos12050547.
Fernandez, N., S. Katipamula, W. Wang, Y. Xie, M. Zhao, and C. Corgin. 2017. Impacts of Commercial Building Controls on Energy Savings and Peak Load Reduction. PNNL Report to DOE. May. PNNL-25985. https://buildingretuning.pnnl.gov/publications/PNNL-25985.pdf.
Fisher Sheehan & Colton. 2021. “What Is the Home Energy Affordability Gap?” Fisher Sheehan & Colton Public Finance and General Economics. http://www.homeenergyaffordabilitygap.com/01_whatIsHEAG2.html.
Fowlie, M., M. Greenstone, and C. Wolfram. 2018. “Do Energy Efficiency Investments Deliver? Evidence from the Weatherization Assistance Program.” Quarterly Journal of Economics 133(3):1597–1644. https://doi.org/10.1093/qje/qjy005.
Frank, M., and S. Nowak. 2015. “Who Is Participating, and Who Is Not? An Analysis of Demographic Data from California Residential Energy-Efficiency Programs, 2010–2012.” “Behavior, Energy and Climate Change.” Presented at BECC Conference, October 2015. https://beccconference.org/wp-content/uploads/2015/10/presentation_frank.pdf.
Group14 Engineering. 2020. “Electrification of Commercial and Residential Buildings: An Evaluation of the System Options, Economics, and Strategies to Achieve Electrification of Buildings.” Denver, CO: Group 14 Engineering, PCB. Prepared for Community Energy Inc., November 2020. https://www.communityenergyinc.com/wp-content/uploads/Building-Electrification-Study-Group14-2020-11.09.pdf.
Guidehouse Insights. 2022. “The IRAs Impact on Building Efficiency and Decarbonization Markets.” Latest Insights, September 26. https://guidehouseinsights.com/news-and-views/the-iras-impact-on-building-efficiency-and-decarbonization-markets.
Hale, E., H. Horsey, B. Johnson, M. Muratori, E. Wilson, B. Borlaug, C. Christensen, et al. 2018. “The Demand-Side Grid (Dsgrid) Model Documentation.” NREL/TP-6A20-71492, 1465659, MainId:16928. https://doi.org/10.2172/1465659.
Harding, T. 2021. “Ithaca Becomes First City in U.S. to Try and Electrify All Buildings.” Ithaca Times, November 9. https://www.ithaca.com/news/ithaca/ithaca-becomes-first-city-in-u-s-to-try-and-electrify-all-buildings/article_03c6e99-841bb-11ec-9a84-47a7c90ee120.html.
Hart, Z., C. Majersik, and J. Eagles. 2022. Leveling Up Building Performance Regulations: How Governments Can Craft Equitable, Effective Building Performance Standards to Drive Widespread Market Transformation. Institute for Market Transformation. https://www.imt.org/wp-content/uploads/2022/08/SummerStudy22-BPS-Equity-Hart.pdf.
Hassell, S., A. Wong, A. Houser, D. Knopman, and M.A. Bernstein. 2003. “Building Better Homes: Government Strategies for Promoting Innovation in Housing.” RAND Corporation.
Heeter, J., K. Xu, and E. Fekete. 2020. “Community Solar 101 [Slides].” NREL/PR-6A20-75982, 1602184. https://doi.org/10.2172/1602184.
Heeter, J., K. Xu, and G. Chan. 2021. Sharing the Sun: Community Solar Deployment, Subscription Savings, and Energy Burden Reduction. National Renewable Energy Laboratory. NREL/PR-6A20-80246. https://www.nrel.gov/docs/fy21osti/80246.pdf.
Hernández, D., Y. Aratani, and Y. Jiang. 2014. Energy Insecurity Among Families with Children. New York: National Center for Children in Poverty (NCCP), Columbia University Mailman School of Public Health. https://www.nccp.org/wp-content/uploads/2020/05/text_1086.pdf.
HHS (Department of Health and Human Services). 2023. “Low Income Home Energy Assistance Program (LIHEAP).” https://www.acf.hhs.gov/ocs/programs/liheap.
HUD (Department of Housing and Urban Development). 2023. “HUD Publishes Updated Public and Assisted Housing Guidance for Treatment of Solar Programs for Residents to Benefit from President Biden’s Investing in America Agenda.” https://www.hud.gov/press/press_releases_media_advisories/hud_no_23_162.
Hughes, T.P. 1993. “Networks of Power: Electrification in Western Society, 1880–1930.” Baltimore, MD: Johns Hopkins University Press. https://www.press.jhu.edu/books/title/2031/networks-power.
IMT (Institute for Market Transformation). 2022. “U.S. City, County, and State Policies for Existing Buildings: Benchmarking, Transparency, and Beyond.” Institute for Market Transformation. https://www.imt.org/wp-content/uploads/2022/06/IMT-Benchmarking-Map-1.pdf.
IPCC (Intergovernmental Panel on Climate Change). 2023. “Sixth Assessment Report.” Intergovernmental Panel on Climate Change. https://www.ipcc.ch/assessment-report/ar6.
Jones, C.M., S.M. Wheeler, and D.M. Kammen. 2018. “Carbon Footprint Planning: Quantifying Local and State Mitigation Opportunities for 700 California Cities.” Urban Planning 3(2:35–51). https://doi.org/10.17645/up.v3i2.1218.
King, J. 2018. “Energy Impacts of Smart Home Technologies.” American Council for an Energy-Efficient Economy. https://www.aceee.org/research-report/a1801.
Kirk, C. 2021. “Los Angeles Building Decarbonization: Tenant Impact and Recommendations.” Strategic Actions for a Just Economy (SAJE). https://www.saje.net/wp-content/uploads/2021/12/LA-Building-Decarb_Tenant-Impact-and-Recommendations_SAJE_December-2021-1.pdf.
Kresowik, M., and L. Reeg. 2022. “Funding Our Future: Creating a One-Stop Shop for Whole-Home Retrofits.” RMI. https://rmi.org/creating-a-one-stop-shop-for-whole-home-retrofits.
Langevin, J., A. Satre-Meloy, A.J. Satchwell, R. Hledik, J. Olszewski, K. Peters, and H.C. Putra. 2022. “The Role of Buildings in U.S. Energy System Decarbonization by Mid-Century.” SSRN Scholarly Paper. https://doi.org/10.2139/ssrn.4253001.
Leach, M., S. Pless, and P. Torcellini. 2014. “Cost Control Best Practices for Net Zero Energy Building Projects.” National Renewable Energy Laboratory. NREL/CP-5500-61365. https://www.nrel.gov/docs/fy14osti/61365.pdf.
Litjens, G.B.M.A., E. Worrell, and W.G.J.H.M. van Sark. 2018. “Lowering Greenhouse Gas Emissions in the Built Environment by Combining Ground Source Heat Pumps, Photovoltaics and Battery Storage.” Energy and Buildings 180:51–71.
Mai, T., P. Jadun, J. Logan, C. McMillan, M. Muratori, D. Steinberg, L. Vimmerstedt, R. Jones, B. Haley, and B. Nelson. 2018. Electrification Futures Study: Scenarios of Electric Technology Adoption and Power Consumption for the United States. Golden, CO: National Renewable Energy Laboratory. NREL/TP-6A20-71500. https://www.nrel.gov/docs/fy18osti/71500.pdf.
Martín, C., and A. McCoy. 2019. Building Even Better Homes: Strategies for Promoting Innovation in Home Building. Washington, DC: Department of Housing and Urban Development. https://www.huduser.gov/portal/sites/default/files/pdf/BuildingEvenBetterHomes.pdf.
Martín, C., M. Bueno, M. Johnson, F. Montes, and R. Frost. 2022. “Targeting Weatherization: Supporting Low-Income Renters in Multifamily Properties Through the Infrastructure Investment and Jobs Act’s Funding of the Weatherization Assistance Program and Beyond.” Harvard University Joint Center for Housing Studies. www.jchs.harvard.edu/research-areas/working-papers/targeting-weatherization-supporting-low-income-renters-multifamily.
McCoy, M. 2022. “The State(s) of Distributed Solar—2021 Update.” Institute for Local Self-Reliance. https://ilsr.org/the-states-of-distributed-solar.
NAR (National Association of Realtors). 2020. “How Long Do Homeowners Stay in Their Homes?” Economists’ Outlook (blog), January 8. www.nar.realtor/blogs/economists-outlook/how-long-do-homeowners-stay-in-their-homes.
NASCSP (National Association for State Community Services Programs). 2019. Weatherization Assistance Program PY2019 Funding Report. Washington, DC: National Association of State Community Service Programs, WAP. https://nascsp.org/wp-content/uploads/2021/01/NASCSP-2019-WAP-Funding-Survey_Final.pdf.
NASEM (National Academies of Sciences, Engineering, and Medicine). 2017. Enhancing the Resilience of the Nation’s Electricity System. Washington, DC: The National Academies Press. https://doi.org/10.17226/24836.
NASEM. 2021a. Accelerating Decarbonization of the U.S. Energy System. Washington, DC: The National Academies Press. https://doi.org/10.17226/25932.
NASEM. 2021b. The Future of Electric Power in the United States. Washington, DC: The National Academies Press. https://doi.org/10.17226/25968.
NASEM. 2023. The Role of Net Metering in the Evolving Electricity System. Washington, DC: The National Academies Press. https://doi.org/10.17226/26704.
NASEO (National Association of State Energy Officials). 2022. “Home Energy Labeling.” National Association of State Energy Officials. November 2022. https://naseo.org/issues/buildings/home-energy-labeling.
National BPS (Building Performance Standards) Coalition. n.d. “White House National Building Performance Standards Coalition.” National BPS Coalition. https://nationalbpscoalition.org. Accessed August 10, 2023.
NBI (New Buildings Institute). 2012. “Getting to Zero 2012 Status Update: First Look at the Costs and Features of Zero Energy Commercial Buildings.” https://newbuildings.org/wp-content/uploads/2015/11/GettingtoZeroReport_01.pdf.
NBI. 2017. “Model Stretch Code Provisions for a 20% Performance Improvement in New Commercial Construction.” https://newbuildings.org/wp-content/uploads/2017/11/20percent_code_provisions_SummaryDoc_FINAL.pdf.
NBI. 2019. “2019 New York Getting to Zero Status Report.” New Buildings Institute for NYSERDA. https://newbuildings.org/wp-content/uploads/2019/04/NY-GTZ-Status-Report_0419.pdf.
New York State Climate Action Council. 2022. “New York State Climate Action Council Scoping Plan.” https://climate.ny.gov/-/media/project/climate/files/NYS-Climate-Action-Council-Final-Scoping-Plan-2022.pdf.
Nowak, D.J., and E.J. Greenfield. 2018. “Declining Urban and Community Tree Cover in the United States.” Urban Forestry and Urban Greening 32:32–55. https://doi.org/10.1016/j.ufug.2018.03.006.
NYC (New York City). 2022. “Sustainable Buildings: Compliance.” City of New York. https://www.nyc.gov/site/sustainablebuildings/requirements/compliance.page.
Palmer, K., and M. Walls. 2015. “Can Benchmarking and Disclosure Laws Provide Incentives for Energy Efficiency Improvements in Buildings?” Discussion Paper RFF-DP-15-09. Resources for the Future. https://media.rff.org/documents/RFF-DP-15-09.pdf.
Palmer, K., and M. Walls. 2017. “Using Information to Close the Energy Efficiency Gap: A Review of Benchmarking and Disclosure Ordinances.” Energy Efficiency 10(3):673–691. https://doi.org/10.1007/s12053-016-9480-5.
Pantano, S. 2020. “Heating Electrification: The Next Opportunity for Coordinated Climate Action.” CLASP. https://www.clasp.ngo/updates/heating-electrification-the-next-opportunity-for-coordinated-climate-action.
Philadelphia Energy Authority. 2023. “The Inflation Reduction Act (IRA) and Commercial Properties.” Philadelphia Energy Authority. https://philaenergy.org/the-inflation-reduction-act-ira-and-commercial-properties.
PNNL and DOE (Pacific Northwest National Laboratory and Department of Energy). 2022. “Historical Model Energy Code Improvement.” Tableau Public. December 21. https://public.tableau.com/app/profile/doebecp/viz/HistoricalModelEnergyCodeImprovement/CombinedHistoricalCodeImprovement_1.
Riahi, L. 2015. “District Energy in Cities: Unlocking the Potential of Energy Efficiency and Renewable Energy.” United Nations Environment Programme. http://www.unep.org/resources/report/district-energy-cities-unlocking-potential-energy-efficiency-and-renewable-energy.
RMI. n.d. “The Retrofit Depot.” https://rmi.org/our-work/buildings/deep-retrofit-tools-resources/deep-retrofit-case-studies.
Shang, L., H.W. Lee, S. Dermisi, and Y. Choe. 2020. “Impact of Energy Benchmarking and Disclosure Policy on Office Buildings.” Journal of Cleaner Production 250(March):119500. https://doi.org/10.1016/j.jclepro.2019.119500.
Shove, E. 2021. “Time to Rethink Energy Research.” Nature Energy 6(2):118–120. https://doi.org/10.1038/s41560-020-00739-9.
Smedick, D., R. Golden, and A. Petersen. 2022. “The Inflation Reduction Act Could Transform the US Buildings Sector.” RMI. https://rmi.org/the-inflation-reduction-act-could-transform-the-us-buildings-sector.
Sobin, R. 2021. “State and Local Building Policies and Programs for Energy Efficiency and Demand Flexibility.” NASEO. https://www.naseo.org/data/sites/1/documents/publications/NASEO%20BldgPolicies%20EE%20and%20DF%20Feb%202021.pdf.
SSC (Smart Surfaces Coalition). 2022. “Cooling Cities, Slowing Climate Change, and Enhancing Equity: Costs and Benefits of Smart Surfaces Adoption for Baltimore.” https://smartsurfacescoalition.org/baltimore-report.
State of Massachusetts. 2022. “Stretch Energy Code Development 2022.” https://www.mass.gov/info-details/stretch-energy-code-development-2022.
Steinberg, D., D. Bielen, J. Eichman, K. Eurek, J. Logan, T. Mai, C. McMillan, A. Parker, L. Vimmerstedt, and E. Wilson. 2017. “Electrification and Decarbonization: Exploring U.S. Energy Use and Greenhouse Gas Emissions in Scenarios with Widespread Electrification and Power Sector Decarbonization.” NREL/TP—6A20-68214, 1372620. https://doi.org/10.2172/1372620.
Strohbach, M.W., A.O. Döring, M. Möck, M. Sedrez, O. Mumm, A.K. Schneider, S. Weber, and B. Schröder. 2019. “The ‘Hidden Urbanization’: Trends of Impervious Surface in Low-Density Housing Developments and Resulting Impacts on the Water Balance.” Frontiers in Environmental Science 29. https://doi.org/10.3389/FENVS.2019.00029.
Taha, H. 2021. “Development of an Urban Heat Mitigation Plan for the Greater Sacramento Valley, California, a Csa Koppen Climate Type.” Sustainability 13(17):9709. https://doi.org/10.3390/su13179709.
Tonn, B., E. Rose, and B. Hawkins. 2018. “Evaluation of the US Department of Energy’s Weatherization Assistance Program: Impact Results.” Energy Policy 118:279–290. https://doi.org/10.1016/j.enpol.2018.03.051.
Tyler, M., D. Winiarski, M. Rosenberg, and B. Liu. 2021. Impacts of Model Building Energy Codes—Interim Update. USDOE. PNNL-31437. https://doi.org/10.2172/1808877.
UN (United Nations). 2023. “2.f. Amendment to the Montreal Protocol on Substances That Deplete the Ozone Layer.” United Nations Treaty Collection. https://treaties.un.org/Pages/ViewDetails.aspx?src=IND&mtdsg_no=XXVII-2f&chapter=27&clang=_en.
UNEP (United Nations Environment Programme). 2019. District Energy in Cities Initiative—[Factsheet]. https://wedocs.unep.org/20.500.11822/31588.
Ungar, L., and S. Nadel. 2019. Halfway There: Energy Efficiency Can Cut Energy Use and Greenhouse Gas Emissions in Half by 2050. U1907. Washington, DC: American Council for an Energy-Efficient Economy. https://www.aceee.org/research-report/u1907.
U.S. Bureau of Economic Analysis. 2022. “Gross Domestic Product (Third Estimate), Corporate Profits (Revised Estimate), and GDP by Industry, Third Quarter 2022: Table 18. Price Indexes for Gross Output by Industry Group: Percent Change from Preceding Period.” https://www.bea.gov/sites/default/files/2022-12/gdp3q22_3rd.pdf.
U.S. Census Bureau. 2022. “American Housing Survey (AHS).” Department of Housing and Urban Development. https://www.census.gov/programs-surveys/ahs.html.
USGBC MA (U.S. Green Building Council Massachusetts). 2019. Zero Energy Buildings in Massachusetts: Saving Money from the Start 2019 Report. USGBC MA and Integral Group. https://builtenvironmentplus.org/wp-content/uploads/2019/09/ZeroEnergyBldgMA2019.pdf.
Waite, M., and V. Modi. 2020. “Electricity Load Implications of Space Heating Decarbonization Pathways.” Joule 4(2): 376–394. https://doi.org/10.1016/j.joule.2019.11.011.
Walton, R. 2022. “Slow Adoption of Smart Thermostats in the US Misses Big Potential Energy Savings: S&P.” Utility Dive, August 31. https://www.utilitydive.com/news/smart-thermostats-us-slow-adoption-misses-energy-savings/630901.
White House. 2022a. “Fact Sheet: Biden-Harris Administration Announces First-Ever Federal Building Performance Standard, Catalyzes American Innovation to Lower Energy Costs, Save Taxpayer Dollars, and Cut Emissions.” Briefing Room, Statements and Releases. https://www.whitehouse.gov/briefing-room/statements-releases/2022/12/07/fact-sheet-biden-harris-administration-announces-first-ever-federal-building-performance-standard-catalyzes-american-innovation-to-lower-energy-costs-save-taxpayer-dollars-and-cut-emissions.
White House. 2022b. “Fact Sheet: Biden-Harris Administration Takes More Than 100 Actions in 2022 to Strengthen Energy Efficiency Standards and Save Families Money.” https://www.whitehouse.gov/briefing-room/statements-releases/2022/12/19/fact-sheet-biden-harris-administration-takes-more-than-100-actions-in-2022-to-strengthen-energy-efficiency-standards-and-save-families-money.
Wood Mackenzie and Solar Energy Industries Association. 2021. US Solar Market Insight, Executive Summary, Q4 2021. https://www.woodmac.com.
Woody, T. 2022. “Silicon Valley’s Menlo Park Plans to Electrify 10,000 Buildings.” Bloomberg, June 17. https://www.bloomberg.com/news/articles/2022-06-17/silicon-valley-s-menlo-park-plans-to-electrify-10-000-buildings.